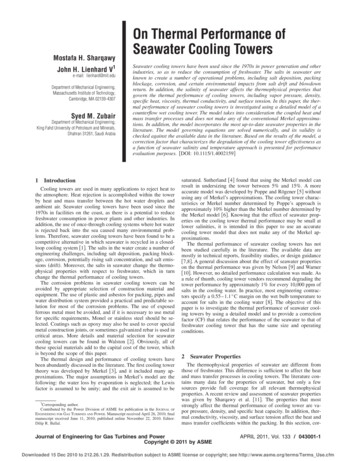
Transcription
Mostafa H. SharqawyJohn H. Lienhard V1e-mail: lienhard@mit.eduDepartment of Mechanical Engineering,Massachusetts Institute of Technology,Cambridge, MA 02139-4307Syed M. ZubairDepartment of Mechanical Engineering,King Fahd University of Petroleum and Minerals,Dhahran 31261, Saudi Arabia1On Thermal Performance ofSeawater Cooling TowersSeawater cooling towers have been used since the 1970s in power generation and otherindustries, so as to reduce the consumption of freshwater. The salts in seawater areknown to create a number of operational problems, including salt deposition, packingblockage, corrosion, and certain environmental impacts from salt drift and blowdownreturn. In addition, the salinity of seawater affects the thermophysical properties thatgovern the thermal performance of cooling towers, including vapor pressure, density,specific heat, viscosity, thermal conductivity, and surface tension. In this paper, the thermal performance of seawater cooling towers is investigated using a detailed model of acounterflow wet cooling tower. The model takes into consideration the coupled heat andmass transfer processes and does not make any of the conventional Merkel approximations. In addition, the model incorporates the most up-to-date seawater properties in theliterature. The model governing equations are solved numerically, and its validity ischecked against the available data in the literature. Based on the results of the model, acorrection factor that characterizes the degradation of the cooling tower effectiveness asa function of seawater salinity and temperature approach is presented for performanceevaluation purposes. 关DOI: 10.1115/1.4002159兴IntroductionCooling towers are used in many applications to reject heat tothe atmosphere. Heat rejection is accomplished within the towerby heat and mass transfer between the hot water droplets andambient air. Seawater cooling towers have been used since the1970s in facilities on the coast, as there is a potential to reducefreshwater consumption in power plants and other industries. Inaddition, the use of once-through cooling systems where hot wateris rejected back into the sea caused many environmental problems. Therefore, seawater cooling towers have been found to be acompetitive alternative in which seawater is recycled in a closedloop cooling system 关1兴. The salts in the water create a number ofengineering challenges, including salt deposition, packing blockage, corrosion, potentially rising salt concentration, and salt emissions 共drift兲. Moreover, the salts in seawater change the thermophysical properties with respect to freshwater, which in turnchange the thermal performance of cooling towers.The corrosion problems in seawater cooling towers can beavoided by appropriate selection of construction material andequipment. The use of plastic and asbestos for packing, pipes andwater distribution system provided a practical and predictable solution for most of the corrosion problems. The use of exposedferrous metal must be avoided, and if it is necessary to use metalfor specific requirements, Monel or stainless steel should be selected. Coatings such as epoxy may also be used to cover specialmetal construction joints, or sometimes galvanized rebar is used incritical areas. More details and material selection for seawatercooling towers can be found in Walston 关2兴. Obviously, all ofthese special materials add to the capital cost of the tower, whichis beyond the scope of this paper.The thermal design and performance of cooling towers havebeen abundantly discussed in the literature. The first cooling towertheory was developed by Merkel 关3兴, and it included many approximations. The major assumptions in Merkel’s model are thefollowing: the water loss by evaporation is neglected; the Lewisfactor is assumed to be unity; and the exit air is assumed to be1Corresponding author.Contributed by the Power Division of ASME for publication in the JOURNAL OFENGINEERING FOR GAS TURBINES AND POWER. Manuscript received April 26, 2010; finalmanuscript received June 11, 2010; published online November 22, 2010. Editor:Dilip R. Ballal.saturated. Sutherland 关4兴 found that using the Merkel model canresult in undersizing the tower between 5% and 15%. A moreaccurate model was developed by Poppe and Rögener 关5兴 withoutusing any of Merkel’s approximations. The cooling tower characteristics or Merkel number determined by Poppe’s approach isapproximately 10% higher than the Merkel number determined bythe Merkel model 关6兴. Knowing that the effect of seawater properties on the cooling tower thermal performance may be small atlower salinities, it is intended in this paper to use an accuratecooling tower model that does not make any of the Merkel approximations.The thermal performance of seawater cooling towers has notbeen studied carefully in the literature. The available data aremostly in technical reports, feasibility studies, or design guidance关7,8兴. A general discussion about the effect of seawater propertieson the thermal performance was given by Nelson 关9兴 and Warner关10兴. However, no detailed performance calculation was made. Asa rule of thumb, cooling tower vendors recommend degrading thetower performance by approximately 1% for every 10,000 ppm ofsalts in the cooling water. In practice, most engineering contractors specify a 0.55– 1.1 C margin on the wet bulb temperature toaccount for salts in the cooling water 关8兴. The objective of thispaper is to investigate the thermal performance of seawater cooling towers by using a detailed model and to provide a correctionfactor 共CF兲 that relates the performance of the seawater to that offreshwater cooling tower that has the same size and operatingconditions.2Seawater PropertiesThe thermophysical properties of seawater are different fromthose of freshwater. This difference is sufficient to affect the heatand mass transfer processes in cooling towers. The literature contains many data for the properties of seawater, but only a fewsources provide full coverage for all relevant thermophysicalproperties. A recent review and assessment of seawater propertieswas given by Sharqawy et al. 关11兴. The properties that moststrongly affect the thermal performance of cooling tower are vapor pressure, density, and specific heat capacity. In addition, thermal conductivity, viscosity, and surface tension affect the heat andmass transfer coefficients within the packing. In this section, cor-Journal of Engineering for Gas Turbines and PowerCopyright 2011 by ASMEAPRIL 2011, Vol. 133 / 043001-1Downloaded 15 Dec 2010 to 212.26.1.29. Redistribution subject to ASME license or copyright; see http://www.asme.org/terms/Terms Use.cfm
Fig. 1 Seawater specific heat calculated using Eq. „2 Fig. 2 Seawater density calculated using Eq. „3 relations of seawater properties to be used in the cooling towermodel are described. All liquid properties are given at 1 atm pressure.The vapor pressure of seawater is less than that of freshwater,which reduces the potential for water evaporation. The vapor pressure can be calculated using Raoult’s law, which states that thevapor pressure of seawater is equal to the product of water molefraction in seawater and water’s vapor pressure in the pure state.The mole fraction of water in seawater is a function of the salinity.Using these results, an equation for seawater vapor pressure basedon Raoult’s law is given byThe density of seawater is higher than that of freshwater due tothe salt content. This increases the mass flow rate of seawater forthe same volumetric flow rate and consequently increases thepumping power. The density of seawater can be calculated usingEq. 共3兲 关11兴, which best fits the seawater density data measured byIsdale and Morris 关15兴 and Millero and Poisson 关16兴 and is ingood agreement with IAPWS 关17兴. The freshwater density isgiven by Eq. 共4兲, which best fits the freshwater density data extracted from the IAPWS 关18兴 formulation of pure liquid water.Equation 共3兲 has an accuracy of 0.1% and is valid for temperatures of 0 – 180 C and salinities of 0–160 g/kg,pv,w/pv,sw 1 0.57357 冉S1000 S冊共1兲where S is the seawater salinity in g/kg on the referencecomposition salinity scale defined by Millero et al. 关12兴, which iscurrently the best estimate for the absolute salinity of seawater.From Eq. 共1兲, it is shown that the seawater vapor pressure decreases with the increase in salinity. This decrease reaches about8% at salinity of 120 g/kg. Consequently, the humidity ratio andthe enthalpy of saturated air above the water surface decrease. Aswill be seen later in the cooling tower model, the difference between the enthalpy of saturated humid air at water temperatureand the enthalpy of humid air at air temperature and humidity isthe driving force for water evaporation and mass transfer 关13兴.Therefore, the reduction of vapor pressure by salinity decreasesthe amount of water evaporated into the air stream and hencereduces the heat rejection capability.The specific heat of seawater is less than that of freshwater,which reduces the amount of sensible heat that can be transferredat the same temperature difference. The specific heat capacity canbe calculated by using Eq. 共2兲 given by Jamieson et al. 关14兴,which fits the experimental measurements with an accuracy of 0.3%. Equation 共2兲 is valid for temperatures of 0 – 180 C andsalinities of 0–180 g/kg,c p,sw A BT CT2 DT3 sw w S共b1 b2t b3t2 b4t3 b5St2兲共3兲 w 共a1 a2t a3t2 a4t3 a5t4兲共4兲where sw and w are in kg/ m , t is in C, and S is in g/kg, and3a1 9.999 102,a2 2.034 10 2,a4 2.261 10 5,a3 6.162 10 3a5 4.657 10 8,b2 2.001 10 3,b3 1.677 10 5,b1 0.8020b4 3.060 10 8b5 1.613 10 11Figure 2 shows the density of seawater calculated from Eq. 共3兲as it changes with temperature and salinity. It is shown in Fig. 2that the density of seawater is higher than that of freshwater byabout 10% at 120 g/kg salinity.The viscosity of seawater is higher than that of freshwater byabout 40% at a salinity of 120 g/kg 共see Fig. 3兲. It can be calculated using Eq. 共5兲 given by Sharqawy et al. 关11兴, which is valid共2兲where c p,sw is in kJ/kg.K, T is in K, and S is in g/kg, andA 5.328 9.76 10 2S 4.04 10 4S2B 6.913 10 3 7.351 10 4S 3.15 10 6S2C 9.6 10 6 1.927 10 6S 8.23 10 9S2D 2.5 10 9 1.666 10 9S 7.125 10 12S2Figure 1 shows the specific heat of seawater calculated from Eq.共2兲 as a function of temperature and salinity. It is shown that thespecific heat of seawater is less than that of freshwater by about12% at 120 g/kg salinity.043001-2 / Vol. 133, APRIL 2011Fig. 3Seawater viscosity using Eq. „5 Transactions of the ASMEDownloaded 15 Dec 2010 to 212.26.1.29. Redistribution subject to ASME license or copyright; see http://www.asme.org/terms/Terms Use.cfm
Fig. 4 Seawater surface tension using Eq. „7 Fig. 5 Seawater thermal conductivity using Eq. „9 for temperatures of 0 – 180 C and salinities of 0–150 g/kg and hasan accuracy of 1.5%. The pure water viscosity is given by Eq.共6兲, which fits data extracted from the IAPWS 关19兴 release with anaccuracy of 0.05% and is valid for t 0 – 180 C, sw w共1 AS BS 兲共5兲2 w 4.2844 10 共0.157共t 64.993兲 91.296兲 52共6兲 1where sw and w are in kg/m s, t is in C, and S is in g/kg, andA 1.541 10 3 1.998 10 5t 9.52 10 8t2B 7.974 10 7.561 10 t 4.724 10 6 8t sw 1 共0.000226 t 0.00946兲ln共1 0.0331 S兲 w冉t 273.15647.096冊 冋冉1.2561 0.625 1 10 2The surface tension of seawater is higher than that of freshwaterby about 1.5% at salinity of 40 g/kg 共see Fig. 4兲. Unfortunately,the available data and correlations for seawater surface tension arelimited to temperatures of 40 C and salinities of 40 g/kg 关11兴.Surface tension can be calculated using Eq. 共7兲, which is valid fortemperatures of 0 – 40 C and salinities of 0–40 g/kg with an accuracy of 0.2%. Pure water surface tension is given by Eq. 共8兲关20兴, which is valid for t 0 – 370 C and has an accuracy of 0.08%, w 0.2358 1 共7兲t 273.15647.096冊册共8兲There is negligible heat transfer between the tower wallsand the external environment.There is constant mass transfer coefficient throughout thetower.The Lewis factor that relates the heat and mass transfer coefficients is not unity.Water mass flow lost by evaporation is not neglected.Uniform temperature throughout the water stream at anyhorizontal cross section.The cross-sectional area of the tower is uniform.The atmospheric pressure is constant along the tower and isequal to 101.325 kPa.A steady-state heat and mass balance on an incremental volumeleads to the following differential equation 关6兴: energy balance onmoist air,dha MR Me 关Le共hs,w ha兲 共1 Le兲共 s,w 兲hv兴dz共10兲mass balance on water vapor,d MR Me 共 s,w 兲dz共11兲energy balance on seawater,where sw and w are in N/m, t is in C, and S is in g/kg.The thermal conductivity of seawater is less than that of freshwater by about 1% at 120 g/kg 共see Fig. 5兲. It can be calculatedusing Eq. 共9兲 given by Jamieson and Tudhope 关21兴, which is validfor temperatures of 0 – 180 C and salinities of 0–160 g/kg with anaccuracy of 3%,log10共ksw兲 3 log10共240 0.0002S兲冉 0.434 2.3 343.5 0.037St 273.15冊冉1 t 273.15647 0.03S冊0.333共9兲where ksw is in mW/m.K, t is in C, and S is in g/kg.3Cooling Tower ModelA schematic diagram of the counterflow cooling tower is shownin Fig. 6, including the important states and boundary conditions.The assumptions that are used to derive the modeling equationsare as follows:Journal of Engineering for Gas Turbines and PowerFig. 6 Schematic diagram of a counterflow cooling towerAPRIL 2011, Vol. 133 / 043001-3Downloaded 15 Dec 2010 to 212.26.1.29. Redistribution subject to ASME license or copyright; see http://www.asme.org/terms/Terms Use.cfm
冋册 冋11 dhadtswd 共tsw tref兲dzMR 共 o 兲c p,sw dzdz册共12兲Mass balance on salts冋册 SdSd dzMR 共 o 兲dzwhereLe hc/hDc p,a 0.8650.667冉冊冉共13兲 s,w 0.622 s,w 0.622 1 /ln s,a 0.622 s,a 0.622冊共14兲MR ṁw,i/ṁa共15兲Me hD aV/ṁw,i共16兲It is important to mention that in the cooling tower literature,the mass flow rate ratio 共MR兲 is usually referred to by L/G 共liquidto-gas flow rate ratio兲, and the Merkel number 共Me兲 is usuallyreferred to by KaV/L, where K is the mass transfer coefficient andL is the water mass flow rate. However, in recent studies 关6,22兴,these symbols have been replaced by the ones used in this paper.In addition, the multiplication of the mass flow rate ratio and theMerkel number 共MR Me兲 is referred to in the literature as thenumber of transfer units 共NTU兲 共Braun et al. 关23兴兲.For a given NTU, the mass flow rate ratio 共MR兲 and inlet conditions 共tw,i , Si , ta,i , i兲, Eqs. 共10兲–共13兲 can be solved numericallyto find the exit conditions for both air and seawater streams. Thesolution is iterative with respect to the outlet air humidity, outletseawater temperature, and outlet seawater salinity 共 o , tw,o , So兲. Inthis solution, seawater properties are calculated along the towerlength using the equations presented in the previous section. TheLewis factor is calculated using Eq. 共14兲 given by Bosnjacovic关24兴, and the moist air properties are calculated using the correlations provided by Klopper 关25兴. In addition, seawater vapor pressure 共Eq. 共1兲兲 is used to determine the humidity ratio and enthalpyof the saturated moist air at seawater temperature.In the above cooling tower model, the heat and mass transfercoefficients are related by the Lewis factor based on Chilton–Colburn analogy. However, the mass transfer coefficient 共hD兲should be determined in order to know the number of transferunits. Unfortunately, general correlations for the mass transfer coefficient in terms of physical properties and packing specificationsdo not exist for cooling towers. For that reason, experimentalmeasurements are normally carried out to determine the transfercharacteristics for different packing types. It is, however, important to note that in the present work an empirical correlation givenby Djebbar and Narbaitz 关26兴 is used to calculate the change innumber of transfer units of a particular packing when seawaterproperties are used instead of freshwater properties. This equationis a modified form of Onda’s correlation 共Onda et al. 关27兴兲 and hasan average error of 26% relative to experimental data. A comparison between the packing characteristic 共Merkel number兲 calculated using Djebbar and Narbaitz’s correlation and the experimental values given by Narbaitz et al. 关28兴 is shown in Fig. 7共a兲.Despite the deviation between Djebbar and Narbaitz’s modeland the experimental measurements, the effect of physical property variation with salinity on the mass transfer coefficient is verysmall, as shown in Fig. 7共b兲. In this figure, the number of transferunits decreases by about 7% at a salinity of 120 g/kg. This reduction agrees well with the data presented by Ting and Suptic 关29兴.They recommended rating the cooling tower as if it was usingfreshwater and then increasing the water flow rate to compensatefor the reduction in the number of transfer units by applying amass flow rate correction factor. This correction factor method canbe used in a design stage of the cooling tower. However, for ratingof cooling towers, if we assume a seawater cooling tower working043001-4 / Vol. 133, APRIL 2011Fig. 7 „a Merkel number calculated using Djebbar and Narbaitz’s correlation and experimental values from the literature.„b Change in the NTU with salinity and water temperature.at the same number of transfer units as of a freshwater tower, it isimportant to calculate the reduction in the cooling tower effectiveness. Therefore, it is assumed in the following analysis that thenumber of transfer units is the same as for a freshwater coolingtower, and the reduction in the effectiveness is calculated subsequently.The mathematical model given by Eqs. 共10兲–共13兲 subject to theboundary conditions shown in Fig. 6 was transferred to finite difference equations and solved by a successive over-relaxationmethod followed a procedure outlined by Patrick 关30兴. A convergence criterion of 共tn 1 tn兲 ⱕ 10 5 was used for the present computations, where n is the number of iterations. Numerical solutions for the air and water temperature distribution along the toweras well as the air humidity and seawater salinity were obtained atdifferent inlet conditions.4Results and DiscussionTo illustrate the results of the present work, the air effectivenessof the cooling tower is calculated at different inlet conditions. Theair effectiveness is defined as the ratio of the actual to maximumpossible air-side heat transfer that would occur if the outlet airstream was saturated at the incoming water temperature 共Narayanet al. 关31兴兲, given by a ha,o ha,ihs,w,i ha,i共17兲To examine the validity of the numerical solution, the results atzero salinity were compared with those given by Braun et al. 关23兴,who solved the same set of equations for the Lewis factor of unityand constant properties. The comparison is achieved by makingTransactions of the ASMEDownloaded 15 Dec 2010 to 212.26.1.29. Redistribution subject to ASME license or copyright; see http://www.asme.org/terms/Terms Use.cfm
Fig. 8 Comparison of tower air effectivenessFig. 11 Effectiveness for inlet water temperature of 80 Cthe necessary adjustments to the present model to suit Braun’sassumptions. Figure 8 shows a comparison between the coolingtower air effectiveness from the present work and from Braun etal. 关23兴. The numerical solution of the present work is in excellentagreement with that of Braun. In addition, Fig. 8 shows the numerical results using Merkel assumptions. The Merkel assumptionsolution differs by 1–3% at these particular conditions; however,at higher water temperatures 共40– 60 C兲, the amount of waterevaporation increases and the difference may reach 10–15%.Figures 9–11 show the air effectiveness of the cooling tower asit changes with the number of transfer units at different mass flowratios and seawater salinity values. In these figures, the dry andwet bulb temperatures of the inlet air are 30 C and 25 C, respectively. In Fig. 9, the inlet water temperature is 40 C, and thesalinity of the inlet seawater is taken as 0 g/kg 共freshwater兲, 40g/kg, and 80 g/kg. As shown in this figure, the air effectivenessdecreases as the salinity increases. The decrease in the effectiveness is a weak function of NTU and MR. The air effectivenessdecreases by about 5% at a salinity of 40 g/kg and by about 10%at a salinity of 80 g/kg.To examine whether the reduction of air effectiveness dependson the seawater inlet temperature, numerical results are obtainedat different seawater inlet temperatures. Figures 10 and 11 showthe air effectiveness versus NTU at seawater inlet temperatures of60 C and 80 C, respectively. It is found that the average reduction in the effectiveness is about 5% at a salinity of 40 g/kg andabout 10% at a salinity of 80 g/kg. This salinity-dependent reduction is the same when the water inlet temperature is 40 C.In Figs. 9–11, it is clear that the air effectiveness of the coolingtower decreases with an increase in the seawater salinity. Thisreduction is a linear function of the salinity, as shown in Fig. 12.However, the slope of this linear relationship depends on the approach 共App兲, which is the difference between the outlet watertemperature and the inlet air wet bulb temperature given byApp Tw,o Twb,i共18兲Figure 12 shows that at a lower approach, the reduction in theeffectiveness is higher than at higher approaches for the sameseawater salinity. This is because at lower approaches the potential for water evaporation decreases 共the difference between saturated air enthalpy at water temperature and air enthalpy is lower兲.Therefore, the effect of reducing the vapor pressure due to theFig. 9 Effectiveness for inlet water temperature of 40 CFig. 10 Effectiveness at inlet water temperature of 60 CJournal of Engineering for Gas Turbines and PowerFig. 12 Effect of salinity on the air effectivenessAPRIL 2011, Vol. 133 / 043001-5Downloaded 15 Dec 2010 to 212.26.1.29. Redistribution subject to ASME license or copyright; see http://www.asme.org/terms/Terms Use.cfm
increase in salinity decreases the air effectiveness by 5–20% relative to the freshwater cooling tower. A correction factor correlation is obtained, which relates the effectiveness of the seawatercooling tower with that of freshwater cooling tower for the sametower size and operating conditions. This correction factor equation is valid up to a salinity of 120 g/kg and is accurate within 2% with respect to the present numerical results.AcknowledgmentThe authors would like to thank King Fahd University of Petroleum and Minerals in Dhahran, Saudi Arabia for funding theresearch reported in this paper through the Center for Clean Waterand Clean Energy at MIT and KFUPM.NomenclatureFig. 13 Effect of approach on the air effectivenesssalts becomes significant on the effectiveness. This is found to betrue for the range of NTU, MR, and tw,i studied in this paper.Therefore, a simple expression is obtained for the reduction in theair effectiveness. The slope of the linear relationship between thesalinity and effectiveness reduction is plotted versus the approachin Fig. 13, and a best fit equation is obtained, as shown in thisfigure. Consequently, a relationship between the air effectivenessreduction as a function of salinity and approach can be expressedas1 a oa 共0.1324 0.0033 App兲 S共19兲where oa and App are the air effectiveness and approach at zerosalinity, respectively. Equation 共19兲 can be rewritten in the form ofa correction factor CF for the air effectiveness. This correctionfactor is the ratio between the air effectiveness at any salinity andthat at zero salinity, written asCF a oa 1 共0.1324 0.0033 App兲 S共20兲It is important to note that Eq. 共20兲 estimates the reduction ofthe cooling tower air effectiveness within 2% from that calculated using the full numerical solution. This can be considered asan accurate estimation at higher salinities where the reduction inthe air effectiveness is high 共14–18%兲. However, at lower salinities, it is recommended to solve the governing equations numerically to get a better estimate. In addition, this correction factorassumes that the number of transfer units is independent of thesalinity, which is an approximation with the following accuracy:The NTU decreases by a maximum of 7% at a salinity of 120 g/kg共for the particular packing shown in Fig. 7兲, which in turn reducesthe effectiveness by an additional 3%. However, for typical seawater salinity of 40 g/kg, the reduction of NTU is about 2% whichreduces the effectiveness by about 0.85%. It is somewhat difficultto combine the effect of salinity on the NTU and the effectivenesssince this calculation must be carried out for a particular packingwith known specifications. Therefore, further reduction in the effectiveness should be considered when using Eq. 共20兲 to accountfor the effect of salinity on the NTU. This reduction ranges from0.85% at typical seawater salinity 共40 g/kg兲 to 3% for salinity of120 g/kg.5ConclusionThe thermal performance of a seawater cooling tower is investigated in this paper. The thermophysical properties of seawaterthat affect the thermal performance are discussed and given as afunction of salinity and temperature. A detailed numerical modelfor a counterflow cooling tower is developed, and a numericalsolution for the air effectiveness is obtained. It is found that an043001-6 / Vol. 133, APRIL 2011a effective surface area for heat and mass transfer per unit volume, m2 m 3App cooling tower approach given by Eq. 共18兲, Kc p specific heat at constant pressure, J kg 1 K 1CF correction factor given by Eq. 共20兲h specific enthalpy, J kg 1hc convective heat transfer coefficient, W m 2 K 1hD mass transfer coefficient 共also K兲, kg m 2 s 1hv specific enthalpy of water vapor, J kg 1k thermal conductivity, W m 1 K 1Le Lewis factor defined by Eq. 共14兲ṁ mass flow rate 共also L兲, kg s 1Me Merkel number 共also KaV/L兲 defined by Eq.共16兲MR inlet water to air mass flow ration number of iterationsNTU number of transfer unitsPv vapor pressure, PaS seawater salinity, g kg 1t temperature, CT temperature, Ktref reference temperature taken as 0 C, CV volume of cooling tower, m3z dimensionless height of packing in the coolingtowerGreek Symbols Subscriptsaiosswwwb effectivenessdensity, kg m 3dynamic viscosity, kg m 1 s 1surface tension, N m 1humidity ratio, kg kg 1moist airinletoutletsaturatedseawaterpure waterwet bulbReferences关1兴 Nester, D. M., 1971, “Salt Water Cooling Tower,” Chem. Eng. Prog., 67共7兲,pp. 49–51.关2兴 Walston, K. R., 1975, “Materials Problems in Salt Water Cooling Towers,”Materials Performance, 14共6兲, pp. 22–26.关3兴 Merkel, F., 1926, “Cooling of Evaporation Temperature,” Journal of the Association of German Engineers, 70共4兲, pp. 123–128.关4兴 Sutherland, J. W., 1983, Thermal Environmental Engineering, 2nd ed.,Prentice-Hall, New York.关5兴 Poppe, M., and Rögener, H., 1991, “Berechnung von Rückkühlwerken,” Section Mj in Verein Deutscher Ingenieure 共The Association of German Engineers兲, VDI-Gesellschaft Verfahrenstechnik und Chemieingenieurwesen共GVC兲: VDI-Wärmeatlas 共VDI Heat Atlas, German edition, Berlin兲, pp. 1–15.Transactions of the ASMEDownloaded 15 Dec 2010 to 212.26.1.29. Redistribution subject to ASME license or copyright; see http://www.asme.org/terms/Terms Use.cfm
关6兴 Kloppers, J. C., and Kröger, D. G., 2005, “Cooling Tower Performance Evaluation: Merkel, Poppe, and -NTU Methods of Analysis,” ASME J. Eng. GasTurbines Power, 127共1兲, pp. 1–7.关7兴 Ying, B. Y., and David, S., 1991, The Use of Cooling Towers for Salt WaterHeat Rejection, The Marley Cooling Tower, Overland Park, KS.关8兴 Eftekharzadeh, S., Baasiri, M. M., and Lindahl, P. A., 2003, “Feasibility ofSeawater Cooling Towers for Large-Scale Petrochemical Development,” Cooling Tower Institute, Report No. TP03-17.关9兴 Nelson, J. A., 1986, “Cooling Tower and Salt Water,” The Marley CoolingTower, Overland Park, KS.关10兴 Warner, M. E., 1974, “Salt Water Natural-Draft Cooling Tower Design Considerations,” Proceedings of the American Power Conference, Vol. 36, pp.442–453.关11兴 Sharqawy, M. H., Lienhard, J. H., V., and Zubair, S. M., 2010, “Thermophysical Properties of Seawater: A Review of Existing Correlations and Data,”Desalination and Water Treatment, 16, pp. 354–380.关12兴 Millero, F. J., Feistel, R., Wright, D., and McDougall, T., 2008, “The Composition of Standard Seawater and the Definition of the Reference-CompositionSalinity Scale,” Deep-Sea Res., Part I, 55, pp. 50–72.关13兴 Baker, D., 1984, Cooling Tower Performance, Chemical Publishing Co., NewYork.关14兴 Jamieson, D. T., Tudhope, J. S., Morris, R., and Cartwright, G., 1969, “Physical Properties of Sea Water Solutions: Heat Capacity,” Desalination, 7共1兲, pp.23–30.关15兴 Isdale, J. D., and Morris, R., 1972, “Physical Properties of Sea Water Solutions: Density,” Desalination, 10共4兲, pp. 329–339.关16兴 Millero, F. J., and Poisson, A., 1981, “International One-Atmosphere Equationof State of Seawater,” Deep-Sea Res., Part A, 28共6兲, pp. 625–629.关17兴 International Association for the Properties of Water and Steam, 2008, “Release on the IAPWS Formulation for the Thermodynamic Properties of Seawater.”关18兴 International Association for the Properties of Water and Steam, 1996, “Release on the IAPWS Formulation 1995 for the Thermodynamic Properties ofJournal of Engineering for Gas Turbines and PowerOrdinary Water Substance for General and Scientific Use.”关19兴 International Association for the Properties of Water and Steam, 2008, “Release on the IAPWS Formulation 2008 for the Viscosity of Ordinary WaterSubstance.”关20兴 International Association for the P
cooling towers can be found in Walston 2 . Obviously, all of these special materials add to the capital cost of the tower, which is beyond the scope of this paper. The thermal design and performance of cooling towers have been abundantly discussed in the literature. The first cooling tower theory was developed by Merkel 3 those of freshwater.