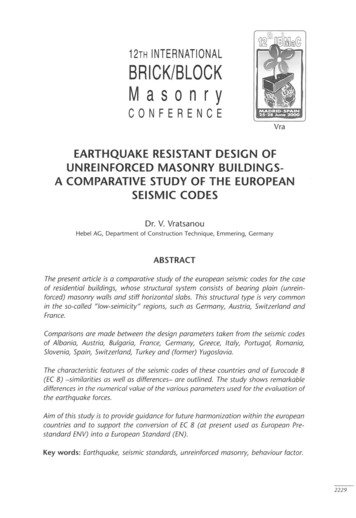
Transcription
12TH INTERNATIONALBRICK/BLOCKMasonryc ONF ERE NCEVraEARTHQUAKE RESISTANT DESIGN OFUNREINFORCED MASONRY BUILDINGSA COMPARATIVE STUDY OF THE EUROPEANSEISMIC CODESDr. V. VratsanouHebel AG, Department of Construction Technique, Emmering, GermanyABSTRACTThe present article is a compara tive study of the european seismic codes for the caseof residentia/ bui/dings, whose structura/ system consists of bearing p/ain (unreinforced) masonry walls and stiff horizontal s/abs. This structura/ type is very commonin the so-called "/ow-seimicity" regions, such as Germany, Austria, Switzer/and andFrance.Comparisons are made between the design parameters taken from the seismic codesof A/bania, Austria, Bu/garia, France, Germany, Greece, /ta/y, Portugal, Romania,S/ovenia, Spain, Switzer/and, Turkey and (former) Yugos/avia.The characteristic features of the seismic codes of these countries and of Eurocode 8(EC 8) -simi/arities as well as differences- are out/ined. The study shows remarkab/edifferences in the numerica/ va/ue of the various parameters used for the eva/uation ofthe earthquake forces.Aim of this study is to provide guidance for future harmonization within the europeancountries and to support the conversion of EC 8 (at present used as European Prestandard ENV) into a European Standard (EN).Key words: Earthquake, seismic standards, unreinforced masonry, behaviour factor.2229
1. INTRODUCTIONA survey of the development of the european seismic codes can be found in (1,2). In 1964 the European Association for Earthquake Engineering (EAEE) was established . Ever since t he beginning of its activity, the subject of harmonization ofseismic codes has been one of its important concerns . Nevertheless, although international scientific cooperation has led to significant convergence concerningthe basic concepts of seismic design, for d ifferent reasons this is not yet fu lly reflected in the existing national codes .A number of comparative studies have already been performed in the case of steeland r.c. buildings (1, 2, 3, 4); significant differences in the choise and numericalvalue of the various parameters used for the evaluation of the earthquake forceswere apparent. However, no such study has been done in the case of residentialbuildings consisted of plain masonry structural walls, although this structural typestill represents the bulk of the construction volume in Europe. Unreinforced masonry structures are predominantly constructed in the so-called "Iow-seismicity"regions -such as Austria, Germany, France and Switzerland- , wh ich according toEC 8 (5) are characterized by an effective ground acceleration less than 1 m fs2(0, 1 9).On the ot her hand EC 8 - Design provisions for earthquake resistance of structures- (5), in 1994 as European Prestandard (ENV) introduced, promises to bean important milestone for the future of the seismic codes harmonization in Europe . Its influence is apparent in the present day revisions of the national codes,in some cases explicitly recognized (e.g. the Slovenian Code (6)) and in othercases by the trend of the proposed changes (e.g. Draft for the new German Seismic Code DIN 4149 (7)) . Moreover, one can expect that the importance of EC8 shall be furthe r enhanced with its conversion to a European Standard (EN),presumably til l 2001 (8).In order to illustrate this situation, in the following a comparison will first be madebetween several design parameters, taken from different european nationalcodes, and subsequently some consideration wil l be given to the characteristicfeatures of EC 8 that appear to support its further improvement and give guidance for future harmonization.2. EUROPEAN SEISMIC CODES - SIMllARITIES AND DIFFERENCES2.1. General conceptSince the concept of the response spectrum was introduced into earthquake engineering by Benioff (1934) and Biot (1941), this technique has been w idely usedto estimate force and deformation demands of structures imposed to earthquakeground motions (9). The response spectrum modal ana lysis is used at present inali european seismic codes (6), i.e. the so-called "dynamic" approach has been2230
adopted. The earthquake induced vibrations influence the value of the seismicloads and their distribution over the height of the building. The elastic responsespectrum Se can be generally described -compare also (10)- as a function of:Se Se {Og, p, S, f3(T), I}where :09design ground acceleration for the reference return period; it accounts forthe seimicity of the zonepseismic risk coefficient; it accounts for the return period of the earthquakeSsoil and foundation coefficientf3(l)amplification facto r corresponding to the vibration period T; it accountsfor the dynamic behaviour of the building subjected to free oscillations.The dynamic facto r corresponds to the fundamental vibration modelshape.importance coefficient; it accounts for the purpose of the building andconsequently its social importance (school, hospital, etc.)Linear elastic response spectra provide a reliable tool to estimate the levei offorces and deformations developed in structures responding elastically duringearthquakes. As a result of currently used seismic design philosophy for buildingstructures that accept structural damage in the event of severe earthquakeground motions, design lateral forces are lower, and in some cases much lowerthan those required to maintain the structure in the elastic range. The capacity ofthe structural systems to resist seismic actions in the nonlinear range permits generally their design for forces smaller than those corresponding to a linear analysis.Thus for economic reasons the positive effect of the inelastic behaviour must betolerated in the structural system .In older codes the positive influence of the nonlinear behaviour of the structureon the seismic load is taken into consideration implicitly by introducing in thestructural analysis design spectral values considerably lower than the elastic response spectral values (2). This is the case for example of the German StandardDIN 4149 (11), where implicitly a reduction factor of 1,8 for ali structural typesand materiais is used for the consideration of the influence of the nonlinear behaviour (10, 12).Nowadays, most of the european seismic codes have adopted the explicit consideration of the positive effect of the nonlinear structural behaviour. To avoidcomplicated nonlinear structural analysis in design, the energy dissipation capacity of the structure, mainly through ductile behaviour of its elements and/or other mechanisms, is taken into account by performing a linear analysis based on aresponse spectrum, which is reduced with respect to the elastic one and henceforth called " design spectrum" . This reduction is accomplished by introducing aforce reduction factor, which reflects generally the ductility of the structural type223 7
Table 7. Design parameters for the evaluation of the design response spectrum adopted in theeuropean seismic codes and their dependance from the influence factors.CountryOesign para meterdepending onreturnperiododbuildingimportanceAlbania (1989)k,Austria (1997)k.Bulgaria (1987)CFrance (1990)seismicintensityk,E,k.soil hk" P(T)P(T)k, (T)'Vk, (T), k,k" k, (T)K:P(T)P(T)Ra,R. (T)R. (T)q'(T)Germany (1981)a!lo, aKP(T)Germany (Oraft, 1998)y,a,,5 d(T)5, S.(T)Sd(T)q, Sd(T)y" R,,(T)Ia, R,,(T)a, R,,(T)R,,(T)q, R,,(T)5ER(T)PaPo(T)Po(T) 'Vq, 5.(T)Greece (1995)Italy (1986)Portugal (1983)Romania (1 992)aK,P, (T)P, (T)Slovenia (1994)y,ao/ 5d(T)ab5, Sd(T)a(T)5d(T)a(T)ah(T)ah(T)ah(T)PK, CdR,(T)Spain (1992)pSwitzerland (1989)PKTurkey (1996)IAo5(T)5(T)f.Yugoslavia (1981)K,K,K.,(T)Kd(T)KpEurocode 8 (1994)y,a, Sd(T)5, Sd(T)q, 5d(T)k, · k",k.(T)5d(T)k.(T)ISO/CO 3010 (1 999)y"ok.T vibrational period of the system.and construction materiais as well as the provided overstrength and increase indamping . The factor used for reducing the elastic response spectrum is called behaviour factor q in EC 8, structural factor RD in ISO/CD 3010, response modification factor R in NEHPR, whereas it is referred to simply as the R coefficient in UBC(5, 14, 15, 16).In the following the term behaviour factor q will be used - in accordance with EC8- although this term does not offer a clear indication of the nature of this factor.Thus the design response spectrum Sd can be expressed as:SP) S.(T) / qThe fundamental flaw of this approach is that it attempts to represent a highlynonlinear problem, which is governed by inelastic deformations, with a simpleelastic problem that is governed by forces (13). It can be argued that the distribution of forces resulting from such analyses often bears little resemblance tothat expected during the actual earthquake; nevertheless, the concept of dividing the elastic response spectrum bya single factor to arrive at the (inelastic) design spectrum is a practical one and has been adopted by most seismic codes,including among others the majority of the european national standards, Eurocode 8 and the american codes, the Un iform Building Code (UBC) and theNEHRP Provisions (14).2232
The definitions of the various design parameters vary widely between the european seismic codes . In Table 1 the parameters for the evaluation of the design response spectrum as well as their dependance from the influence factors are summarized. The parameters according to EC 8 (5) and the Oraft ISO/CO 3010 (15)have also been considered .2.2. Behaviour factor qMost earthquake resistant design codes propose design procedures based -asstated above -on the use of the behaviour factor q. Nevertheless, the general waythe factor q is usually defined, one semi-empirical value for a large group of structures, does not always represent the real structural response. On the other handthe value of this factor is affected from many parameters involved in the designprocedure; more than 12 such parameters have been identified (17).The value of the behaviour facto r mainly depends on the ductility of the structure(which relates to the detailing of the structural members), on the strength reserves that normally exist in a structure (depending mainly on its redundancy, onthe overstrength of individual members, and on the effective damping of thestructure); ali these factors directly affect the energy dissipation capacity of thestructure. Although these principies have long been recognized and implicitlyadopted in various seismic codes, an appropriate definition of the behaviour factor was only relatively recently suggested (19) in the form :q qm. q, . qxwhere qm is the ductility-dependent component, q, the overstrength-dependent component, and qx the damping-dependent component of the behaviourfacto r; the latter is of interest mainly in the case of structures with supplemental damping devices and will not be addressed in the remainder of this paper. Some authors suggest also a separate facto r q, relating to the structure'sredundancy only, but as this is generally difficult to separate from the overstrength factor, in the following discussion it will be included in q" comparealso (16) .A similar formula is proposed in ISO/ CO 3010 (15): the structural factor R may bedivided into two other factors Rm and R, and can be expressed as the product ofboth of them as follows :R Rm . R,where Rm is related to ductility, acceptable deformation and restoring force and-as recent studies indicate- to the natural period of vibration. The factor R, is related to the overstrength and is practically a function of the difference betweenthe actual strength and the calculated strength and varies according to themethod of strength calculation .2233
In EC 8 (5) and in ali seismic codes, that have adopted the principies of the EC 8,such as the French Code (6), the Greek Code (18), the Draft for the new GermanSeismic Code DIN 4149 (7), the Slovenian Code (6), etc., the behaviour factor q"accounts for the non-linear response of a structure, associated with the material, the structural system and the design procedure". The behaviour facto r q is anapproximation of the ratio of the seismic forces, that the structure would experience if its response were completely elastic w ith 5% viscous damping, to the minimum seismic forces that may be used in design -with a conventionallinear model- still ensuring a satisfactory response of the structure (5).However, in the majority of the european seismic codes no explicit definition ofthe behaviour factor is given. Moreover, its physical meaning is not clear, compare also (1). In (19) it is stated: " . it is difficult to judge the rational for the values recommended for these . factors due to a luck of discussion or even any indication of how these values have been derived and what they are meantphysically to represent" .Quantification of the factors qmand qs is still a matter of debate, and in the mosteuropean codes -with the exception of the Swiss Code (6), where the design factor Cd 0,65 is explicitly introduced to compensate for the difference betweenthe design resistance capacity and effective resistance capacity- one generic termq has been adopted. In EC 8 (5) a behaviour factor ofq 1,5is proposed for unreinforced masonry. This value has not been defined on the basis of special studies; it is mainly based on the available experience from the behaviour of masonry buildings during earthquakes, as well as on a criticai reviewof the available theoretical and experimental data (20).There are many efforts in progress, or already implemented, for improving thebehaviour factor by making it period dependent, accounting for overstrengththrough empirical coefficients, or applying modifications for different effectivedamping. Ali these efforts are moves in the right direction, but they are insufficient unless they pay careful and explicit attention to the inelastic behaviour characteristics and the fact that response at the collapse prevention levei is controlledmostly by deformations than forces (13).3. COMPARISON OF THE SEISMIC CODESThe seismic codes of the following countries have been examined: Albania (AL),Austria (A), Bulgaria (BG), France (F), Germany (D), Greece (GR), Italy (I), Portugal (P), Romania (RO), Slovenia (SLO), Spain (E), Switzerland (CH), Turkey (TR)and former Yugoslavia (YU). For the evaluations of the design parameters thecode editions reprinted in (6) have been used with the exception of the Austri an Code (21), the Greek (ode (18) and the Draft for the new German (ode DIN4 149 (7).2234
The calculated spectral values correspond -according to EC 8 (5)- to buildings ofthe importance category 111, i.e. ordinary buildings such as residential and officebuildings where no great loss of human life is expected .The structural system consists of bearing walls made of plain (unreinforced) masonry and stiff horizontal diaphragms.Ali seismic data used correspond to a nominal seismic intensity VIII. The seismicintensity scales -MSC, MM or EMS- used in the european seismic codes vary,but in this paper no try has been given to convert values from one intensityscale to those of another by formula or look-up table, though several such tables have been published (22, 23). As stated in (24):Experience shows thatcomparing different intensity scales is far from straightforward, since values often vary more from investigator to investigator using the same scale, than values change from scale to scale if the investigator remains the same. This is particularly true for the main twelve degree scales because of their essentialsimilarity of outline. If one attempts a comparative evaluation either one highlights small differences in a very literal way or one uses the scales in a more natural, flexible way, and any differences disappear in the interpretation " . Whereas the correspondence between nominal macroseismic intensities and spectralvalues is not given explicitly in the code, it has been assumed on the basis ofthe information given in (25), personal communications, previous code editionsor comparison with similar studies (1, 2) .li".In the case of Germany two codes have been compared: the valid standard DIN4149 (1981) and the Draft DIN 4149 (7) being still under preparation. The DraftDIN 4149 is based on the new German Seismic Map, which was developed byGrünthal (26); the design and safety concepts are based on EC 8 (5).In the case of Slovenia the code of former Yugoslavia is still in use, however EC 8has also been officially adopted (6) . In this study the calculated values correspondto the National Application Document (NAD) and the seismological map ofSlovenia in connection with EC 8.In Figure 1 and 2 the design response spectra Sd for stiff and mean soil conditionsrespectively are represented. Taking into account that the fundamental period Tof plain masonry buildings is approximatelyT 0,1 0,2 sfor masonry buildings up to two storeysT 0,5 sfor masonry buildings up to 5 storeys-compare also (27)- it is obvious that the plateau spectral values are desicive forthe assessment of the low-rise masonry buildings. These values are summarizedin Table 2 and 3 together with the beginning (TB-value) and end (Tc value) of theplateau . In the majority of the cases the soil quality influences only the width ofthe plateau and not its numerical value .2235
Table 2. Design ground acceleration (a9), elastic response spectra (5,), behaviour factors (q),design spectra (5d ), beginning (T,) and end (TJ period of the "plateau" for residentialbuildings consisting of plain masonry structural wal/s in seismic zones of the nominalintensity VIII and stiff soi! conditions.I)1)l)')j)Countrya, (g)max S, (g)qmax Sd S.lq (g)T, (s)T, (s)Albania (1989)0,16')0,3681/0,45 2,220,1660,000,30Austria (1997)0,14')')0,4251,500,2840,100,40Bulgaria (1987)0,15')0,3751/0,40 ,450,20france (1990)0,25')')Germany (1981 )0, 10')Germany (Draft)0,08')0,2001,500,1330,05Greece (1995)0,24')0,6001,500,4000,100,40Italy (1986)0,06')0,126')0,000,80Portugal (1983)0,10')0,6001,000,600')0,000,18Romania (1992)0,20')0,5001/0,30 3,330,1500,000,70Slovenia (1994)0,20')0,5001,500,3330,100,40Spain (1992)0,15')0,3751/0,76 1,320,2850,150,340,33Switzerland (1989)0,16')0,3401,2/0,65 1,850,1840, 10Turkey (1996)0,30')0,7502,50,3000, 100,30Yugoslavia (1981)0,05')0,150')0,000,50Correlation between se ism ic intensi ty and zone is given directly in lhe codeMaximun va lue given in the codeAfte r personal com m unication w ith A. PeckerOn th e basis 01 th e inlormati on given in (25)After personal com mu nication w ith N. Theodou li dis") After com parison w ith sim il ar studics (1, 2)7) After persona l com mu nication w ith E. C. Carva lho, see al50 (28)' ) Compare with the previous code editi on (29)' ) Multiplied by the safety fa ctor 1,54, DISCUSSION OF THE RESULTSAs illustrated in Tab le 2 and 3 a great diversity of solutions for the same problem,that is the description of a design spectrum for a given nominal intensity, a given soil and a given structure, appears in the different codes. To the same conclusion comes also Keintzel (1, 2) in the case of r.c. structures of the importance class11 (buildings with greater gathering of people). It can be seen that a large varietyof elastic spectral values max Se -from 0,20 to 0,75- is combined with a large variety of behaviour factors -from 1,00 to 3,33- to result to a large variety of designspectral va lues max Sd -from 0,08 to 0,60-.In order to gain a reali stic idea about the concepts for seismic protection allowedin the different countries, this diversity has to be connected with three other diversities.The first diversity is the design levei with global or partial safety coefficients. Thisdiversity concerns not only the load side (seismic actions) but also the materialside (resistance). The actual levei of resistance that the building possesses, depends not only on the levei of the design forces but also on the allowable stresses and strains specified in the codes. The relationship between the load and the2236
Table 3. Design ground acceleration (a.), elastic response spectra (5,), behaviour factors (q),design spectra (5d ), beginning (T,) and end (TJ period of the "plateau" for residentialbuildings consisting of plain masonry structural walls in seismic zones of the nominalintensity VIII and mean soil conditions.1)2)l)')S)6)')')')max S. S.lq (g)T, (s)0,1980,000,400,2840,150,60Countrya, (g)max S, (g)Albania (1989)0,22')0,440Austria (1997)0,14')')0,4251,50Bulgaria (1987)france (1990)0,15')0,3751/0,40 1,50Germany (1981)Germany (Draft)Greece (1995)0,10')Italy (1986)0,06')Portugal (1983)Romania (1992)0,10')0,6000,20')0,500Slovenia (1994)0,20')0,500q1/0,45 2,221,001/0,30 3,331,50 400,1500,001,000,3330,150,600,59Spain (1992)0,15')0,3300,2510,20Switzerland (1989)Turkey (1996)0,16')0,3401,2/0,65 1,850,1 840,100,500,30')0,7502,50,3000,150,60Yugoslavia (1981)0,05 ')0,150')0,000,701/0,76Correlatlon between S ISm IC Intenslly and zone 15 glven dlrectly In lhe codeMaximun value given in lhe codeAfter personal com munication with A. PederOn the basis of the information given in (25)After personal communication with N. TheodoulidisAfte r comparison w ith similar studies (1, 2)After personal communication with E. C. Carvalho, see al50 (28)Compare w ith the previous code edition (29)Multiplied by the safety factor 1,5material safety coefficients depends in the most of the cases on economic criteria. Thus the same result can be obtained by specifying a high levei of seismicforces with high allowable stresses and strains or a lower levei of actions with alower levei or resistance. This has been proved in the case of the German Standards through the calculations of Ótes (27, 34) . Whereas the combination of theDraft DIN 4149 (7) with EC 6 (35) ("high" levei standards) or the combinationof the valid DIN 4149 (11) with DIN 1053 (36) ("Iow" levei standards) seems tobe satisfactory, the combination of the Draft DIN 4149 ("high" levei standard)with DIN 1053 ("Iow" levei standard) does not necessarily ensure the satisfaction of the ultimate limit state check. The latter contradicts the "good" engineering experience from the behaviour of the common low-rise masonry buildings in Germany.This diversity is combined with another philosophy, as shown in (1, 2, 30) :whether or not the seismic action is considered an accidental or a variable action .In the most of the cases the internai forces are introduced into the ultimate limitstate check as accidental actions, but there are also exceptions; e.g . according tothe Portuguese Code (6, 28) the internai forces resulting from the application ofthe design spectrum of Figure 1 and 2 are introduced into the ultimate limit statecheck multiplied by the partial safety coefficient for variable actions Yq 1,5.2237
Figure 1. Design response spectra for residential buildings with plain masonry structural wallsin seismic zones of the nominal intensity VIII and stiff soil conditions.0.70p0,600,500,40'CIJ)0,300,200, 10YU0,000,00,51,01,52,0T [5]The second diversity concerns the probability of the occurence of the nominal intensity respectively peak ground acceleration. For example in the Portuguese andthe Bulgarian Code a return period of 1000 years is admitted (6, 28, 32); in theSlovenian and the Yugoslavian Code a return period of 500 years is taken into account (6), whereas in the Austrian Code, the Draft of the New German Code and2238
Figure 2. Design response spectra for residential buildings with plain masonry structural watlsin seismic zones of the nominal intensity VIII and mean soil conditions.0,70p0.600 ,500,400,300,200 ,10RO0.000,00,51,01,52,0T [5]the Greek Code as well as in EC 8 the "standard" return period of 475 years hasbeen adopted (7, 18, 21). For the Swiss Code the return period is 400 years according to (33); the French Code adopts a return period of 250 years (25). TheRomanian and the Spanish Code are based on a return period of 50 years (6). Thisdiversity is also connected with the difterences in the definition of the "eftective"2239
peak ground acceleration. Apart from the Austrian Code (21), where the effectiveground acceleration represents 70% of the peak ground acceleration, the definition of the effective ground acceleration is not given exp licitly in the europeanseismic codes.The third diversity concerns -as stated in chapter 2.2- the definition and the numerical values of the adopted behaviour factors. Differences in the numerical values of the behaviour factor specified in the various codes for the same type ofstructure can be quite substantial; to mention a typical example, the Roman ianCode adopts a "high" behaviour factor of 3,33 (6), whereas according to t he Portuguese Code the positive effect of the nonl inear behaviour of masonry is not tobe considered and as a consequence the use of unreinforced masonry with structural function is not allowed in zones of intensity VIII (28).It has to be noted, however, that the use of a specific value of q for masonry structures in the seismic codes is always t ied w ith other requirements concerning theconfiguration of the build ing and the detailing of the structural members. If thevalue of q alone is used in an earthquake resistant design of masonry structureswithout any other requirements, the resulting design wi ll not be reliable.5. CONCLUSIONSThe comparison of the european seismic standards in the case of residential buildings consisting of bearing plain masonry walls showed considerable differences inthe numerical values of the design response spectra. The disagreements can be explained through 1) the different design levei for seismic actions as well as for resistance, 2) the diversities concerning the seismic data taken as input and 3) the apparent inconsistencies in the way the behaviour factor q is defined and evaluated ineach country. It is to be expected that if appropriate adjustments are made to theseparameters (for actions as well for resistance), differences will become smaller.It is the scope of th is paper to point out the need for harmonization in the caseof masonry structures. Due to their rationality EC 8 (5) combined with EC 6 (35)seem to be promising for the harmonization of the diversity of solutions found inthe present european standards; est ablishing an agreement about the basic philosophy, the requirements and the format, they give to the various countries thepossibility to adapt the codes w ith the help of numerical parameters to their specific needs.Further research is needed to define and eva luate the behaviour factor q in thecase of plain masonry structures. A promising effort and move to the right direction is the methodology used in the Slovenian National Building and Civil Institute (ZAG) in Ljubliana (31). The evaluation of the ductility- dependent component q , together with estimations of the overstrength-dependent component q,can lead to a more rational and transparent seismic design approach than the approach currently used in seismic codes.2240
ACKNOWlEDGEMENTSThe author is indebted to Dr. Keintzel for his helpful suggestions. The help of Dr.Brüstle, Dr. Grünthal and Prof. Kappos is also acknowledged for the informationprovided .REFERENCES1. Keintzel, E.: Trends and achievements in the development of the European Codes for earthquake resistant design o 6'" Internationale Conference on Soil Dynamics and Earthquake Engineering (SDEE), Bath/ UK (1993)2. Keintzel, E.: Development of the European Codes for earthquake resistant designo In: Earthquake Prognostics Strategy. Edited by Andreas Vogel et aI., EPICenter (1998)3. Gandolfi, A.: Analyse comparative des normes sismiques internationales. In: acier-stahl-steel,1/1979, 27-36 (1979)4. Hampe, E.: Bauwerke unter seismischen Einwirkungen - Teil 1 und 2. Studienmaterial. Institut für Aus-und Weiterbildung im Bauwesen, Leipzig (1985)5. Eurocode 8 (EC 8): Design Provision s for Earthquake Resistance of Structures - Part 1 (ENV1998-1-1): Seismic Actions and General Requirements of Structures - Part 2 (ENV 1998-12): General Rules for Buildings - Part 3 (ENV 1998-1-3): Specific Rules for various Materiaisand Elements (1994)6. IAEE (lnternational Association for Earthquake Engineering): Regulations for seismic designoA wor/d list - 1996. Tokyo/Japan (1996)7. Entwurf DIN 4149 - Bauten in deutschen Erdbebengebieten; Auslegung von Hochbautengegen Erdbeben. DIN Deutsches Institut für Normung e.V., Berlin (2000)8. Bossenmayer, H.: Eurocodes für den konstruktiven Ingenieurbau. In: Mauerwerk-Kalender2000, Ernst & Sohn, Berlin, 685-697 (2000)9. Miranda, E.: Evaluation of site-dependent inelastic seismic design spectra. In: Journal ofStructural Engineering, Vol. 119, No. 5, 1319-1338 (1993)10. Sedlacek, G.: Erdbebenberechnung von Bauwerken im internationalen Vergleich. db 11 /8 0,20-23 (1980)11. DIN 4149 - Bauten in deutschen Erdbebengebieten. DIN Deutsches Institut für Normunge.V., Ber/in (1981)12. Müller, F.P., Keintzel, E.: Erdbebensicherung von Hochbauten. Ver/ag Ernst & Sohn, Berlin(1984)13 . Krawinkler, H.: New trends in seismic design and methodology. 10'" European Conferenceon Earthquake Engineering (ECEE). Balkema, Rotterdarm (1995)14. Kappos, A. J.: Evaluation of behaviour factors on the basis of ductility and overstrength studies. In: Engineering structures, 21 , 823-835 (1999)15 . Draft ISO / CD 3010 - Basis for design of structures - Seismic actions on structures (1999)16. Kappos, A.J ., Kyriakakis, P., Athanassiadou, CJ.: Site-dependent inelastic spectra and ductility-based behaviour factors for Greece. 11 '" European Conference on Earthquake Engineering (ECEE), Paris/ France (1998)17. Postelnicu, T.; Zamfirescu, D.: Methodology for the calibration of the
BRICK/BLOCK Masonry c O N F E R E N C E Vra EARTHQUAKE RESISTANT DESIGN OF UNREINFORCED MASONRY BUILDINGS-A COMPARATIVE STUDY OF THE EUROPEAN SEISMIC CODES Dr. V. Vratsanou Hebel AG, Department of Construction Technique, Emmering, Germany ABSTRACT The present article is a compara tive study of the european seismic codes for the case