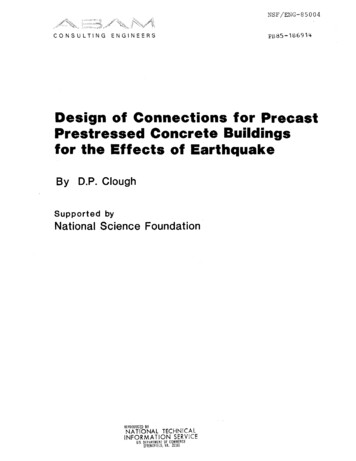
Transcription
NSF/ENG-85004CONSULTINGENGINEERSPl385-186914Design of Connections for PrecastPrestressed Concrete Buildingsfor the Effects of EarthquakeBy D.P. CloughSupported byNational Science FoundationREPROD1JCtD 9YNATIQNAL TECHNICALINFORMATION SERVICEu.s. DEPARTMENTOF COMMERCESPRINGFiElD, VA. 22161
, 50212-101REPORT DOCUMENTATIONPAGE11.REPORT NO.Reoort No. NSF-ENG-850044. TItle and Subtitle5. Report DateMarch 1985Design of Connections for Precast Prestressed ConcreteBuildings for the Effects of Earthquake1. Author(s)8. Performing Organization Rept. No:Douglas P. Clough9. Performing Organization Name and Address10. Project/Task/Work Unit No.ABAM Engineers, Inc.500 South 336th Street, Suite 200Federal Way, WA 9800311. Contract(C) or Grant(G) No.(C)(G)12. Sponsoring Organization Name and AddressNSF GrantNo. CEE-812173313. Type of Report & Period CoveredNational Science FoundationDesign Research ProgramCivil and Environmental DivisionWashington, DC 20550Final Technical Report14.15. Supplementary Notes"16. Abstract (Umit: 200 words)Buildings designed in conformance with typical building code criteria will yield during asignificant earthquake.- Conventional design procedures do not, however, deal explicitlywith the resulting inelastic deformations.In some types of precast concreteconstruction, inelastic deformations concentrate in the' connections between precastelements and careful detailing is required to guard agafnst brittle failure. -In thepresent work, a design methodology has been developed which takes into accountquantitative estimates of plastic deformations and enables a more rational approach toconnection detailing. A simple concept, 4nvolving internal strain energy associated withthe structure's seismic response; is used to estimate the maximum inelastic displacementat roof level. inematic principles are used to transform this II gl obal ll displacement intoplastic deformation estimates for individual joints and connecto s. Results from otherinvestigators are applied to estimate the number of reversed inelastic loading cycles-which must be· sustained during the response to a damaging earthquake. The designmethodology is demonstrated with two example structures typical of precast constructionfor zones of moderate sei smi city in the United States. Strength and deformati onrequirements derived by this approach are presented for selected joints to facilitate theexperimental validation of specific connector designs. Guidelines are presented whichenable quantitative comparison of strength, plastic elongation, and cyclic load reversaldemands on connectors within similar structures sited in zones of hioher seismir-it\l 17. Document Analysisa. DescriptorsEarthquake resistant structures.Precast concrete.Ductility.b. Identifiers/Open-Ended TermsStructural engineering.CoStructural design.Buildings.COSATI FIeld/GrouplL Availability Statement19. security Class (This Report)No restriction on distribution. Available fromNational Technical Information Service,Snrinnfield VA 22161 Renor t No. C -A'21733.(S. . ANSI-Z39.18)UNCLASSIFIED20. security Class (This Page)21. No. of Pages17622. PrieeUNCIA ; ;TFTFOSe. Instructions on ReverseOPTIONAL FORM 212 (4-7n(Formerly NTIS--35)Department of Commerce
DESIGN OF CONNECTIONS FORPRECAST PRESTRESSED CONCRETE BUILDINGSFOR THE EFFECTS OF EARTHQUAKEPhase 2 of a Three-Phase Programby D.P. CloughA Research InvestigationbyABAM Engineers Inc.500 South 336th Street, Suite 200Federal Way, Washington 98003Supported byNational Science FoundationWashington, DCGrant No. CEE-8121733with Assistance fromPrestressed Concrete InstituteChicago, IllinoisMarch 1985"II
ACKNOWLEDGEMENTSIn addition toEngineersInc.the listed author,othersmade significant contributions toon the staff of ABAMthis work.J. S. Apolisassisted in computer model preparation and processing of results for theparking structure example.R. E. Harn assisted in the detail design of con-nections for the parking structure and the bearing wall apartment building.P . E. Kastens prepared many of the figures and M. L. McDonald was responsible for word processing.Special thanks are due to L. R. McCoy for hiseditorial assistance, to C.J. Birkeland for acting as a sounding board work,andtoR. F. Mast for his assistance in planning the project and his comments duringpreparation of the final report.J.R. Janney,D.P. Jenny,L.D. Martin,S.E. Moustafa,andJ. R. Salmons served as a PCI Technical Input Group, and assisted in selecting the examples used to illustrate the design methodology.· In particularIthe author appreciates L.D. Martin's contribution of detailed information onthe Metro-Space Parking System, upon which the example parking structurepresented in this work is based.The bearing wall apartment building which served as the basisfor thesecondI.J. Speyer,exampleS. Firnkas,wasdevelopedbyW. G. Lischka,T.J. Ecsedi, M. Fintel,A. Aswad,J . M. Becker,F.J. Jacques,N. Ladduwahetty, and D. P. Clough, in association with the PCI Committee onPrecast Bearing Wall Buildings, chaired by Alexander Popoff.This structurewas the focal point for a PCI design seminar presented by the committee inOctober 1983.The committee's permission to use its building as an examplein the present research is gratefully acknowledged.This material is based upon work supported by the National ScienceFoundationunderGrantNo. CEE-8121733.Any opinions,findings,con-elusions, or recommendations expressed in this publication are those of theauthorand do not necessarily reflect the views of the National ScienceFoundation or the Prestressed Concrete Institute.iii
TABLE OF CONTENTSChapter1ACKNOWLEDGEMENTS .iiiINTRODUCTION. . . .1.11.12Seismic- Resistant Precast Construction in theUnited States -- An Historical Perspective.1.11.2Toward Improved Building Code Provisions1.31.3Background of the Present Research Effort1.41.4Overview of the Proposed SeismicDesign Methodology . . . . . . .1.51.5Objectives of the Phase 2 Report1.71.6Technical Input Group1.71.7Industry Awareness and Participation are Vital1.8PRECAST CONCRETE CONSTRUCTIONVERSUS EARTHQUAKES.2.12.1Introduction.2.12.2The Origination a-nd Propagation ofSeismic Ground Motions . . . . . .2.22.32.52.52.7Earthquake Response of Buildings.2.3.1Energy as a Measure of Seismic Response2.3.2How Buildings Absorb Seismic Energy . .2.3.3Factors Tending to Limit theAbsorption of Seismic Energy . . . . . .Storage Tank Analogy for the Absorption2 ., . 4and Dissipation of Seismic Energy2.3.5Design Implications . . . . . . .2.132.152.4Multi-Degree-of-Freedom Systems . . . .2.4.1Limitations of the Swing Analogy2.4.2Mode Shapes.2.4.3Modal Response to Dynamic Loads2.172.172.192.192.5Practical Seismic Design Approach. . . .2.20Preceding page blankv2.10
TABLE OF CONTENTS (continued)Chapter2.6Code Methods for Determination ofDesign Lateral Force Magnitude . .2.6.1Uniform Building Code (UBC) Approach2.6.2Applied Technology Council(ATC-3) Approach . . . . .2.242.7Categories of Precast Concrete Construction .2.282.8Differences in the Seismic Behavior of Jointedand Monolithic Precast Structures . . . . . .2.28Toward Rational Design of Seismic-ResistantPrecast Concrete Structures. . . . . . . . .2.292.934A RATIONAL METHODOLOGY FORTHE DERIVATION OF CONNECTORPERFORMANCE led Technical Overview .3.2.1Definition of Structural System3.2.2Concept for Lateral Load Resistance .3.2.3Elastic Analyses for Undamaged Condition3.2.4Specification of Seismic Loads . .3.2.5Assignment of Member Strengths3 .2.6Prediction of Global InelasticDisplacement Magnitude . . . . .3.2.7Kinematic Analysis of Post-Yield Behavior3.2.8Number of Cyclic Load Reversalsto be Sustained . . . . . .3.2.9Specification of ConnectorPerformance Criteria3.2.10 Beyond Traditional Bounds3.23.23.23.43.63.73.83.133.153.153.17EXAMPLE 1 -- FRAME/SHEAR WALLPARKING'STRUCTURE . . . . . . .4.14.1Description of Basic Structural System4.14.2Concept for Lateral Load Resistance. .4.14.3Elastic Analysis for Undamaged Condition4.54.4Specification of Seismic Loads . .4.104.5Assignment of Member Strengths4.10vi
T ABLE OF CONTENTS (continued)Chapter4.6Prediction of Global InelasticDisplacement Magnitude . . .4.134.7Kinematic Analysis of Post-Yield Behavior4.184.8Number of Cyclic Load Reversals to be Sustained4.184.9Specification of Connector Performance Criteria .4.9.1Connections and Special Ductile MembersWithin Primary Lateral Resisting System4.9.2Connections Intended to Ride Alongwith Deformations of the LateralForce Resisting System4.9.3Other Locations4.18Summary4.364.105.4.194.304.34EXAMPLE 2 -- BEARING WALLAPARTMENT BUILDING . . . .5.15.1Description of Structure.5.15.2Concept for Lateral Load Resistance .5.15.3Elastic5.3.15.3.25.3.35.45.45.65.65 .4Specification of Seismic Loads .5.65.5Assignment of Member Strengths5.135. 6Prediction of Global Inelastic Displacements5.165.7Kinematic Analysis of Post-Yield Behavior5.165.8Number of Cyclic Load Reversalsto be Sustained . . . . . . . . .5.225.9Analyses for Undamaged ConditionMember Properties. . .Foundation Properties .Elastic Analysis ResultsSpecification of Connector Performance Criteria5.9.1Load Bearing Wall to HollowCore Floor Joint. . . . . . . . .5.9.2Non-Load-Bearing Wall-to-Wall Joint5.9.3Non-Load-Bearing Wall to HollowCore Floor Joint. . . . . . .5.9.4Corridor Support to Wall Joint. .vii5.225.225.355.425.49
TABLE OF CONTENTS (continued)Chapter5.9.55.9.65.9.75.106Wall-to-Wall Joint . . . . . . . . . . .Hollow-Core to Hollow-Core Edge Joint.Other Locations5.515.595.635.63SummarySUMMARY AND S . .R.16.1viii
CHAPTER 1INTRODUCTION1.1SEISMIC-RESISTANT PRECAST CONSTRUCTION IN THEUNITED STATES -- AN HISTORICAL PERSPECTIVEPrecast concrete buildings have been most widely accepted in regionsof low to moderate seismicity, where they compete effectively with buildings ofcast-in-place concrete and other construction materials.Whilesuperiorprecastcanto rialseconomic considerationsprecast construction its most important competitive advantage.qualityprobablygiveTo gain thegreatest competitive edge, precast manufacturers in the buildings market haveevolved sophisticated modular systems which make maximum repetitive use ofstandard components and connection details.Precast building design hasbecome highly process oriented, involving production, storage, transportation,' handling, and erection of preengineered components which are joined atthe site with a minimum of field labor.Precastbuildingsystems most widely used in theUnitedStatesevolved at a time and in regions where seismic resistance was not a significant design objective.Connection details,standard precast components,production fixtures, and the basic framing concepts of many precast buildingsystems reflect this history to some degree.The present state of the artreflects a substantial investment in design and production tooling for modular1'.systems not ideally suited to earthquake resistance.Retooling costs can discourage large-scale experimentation with newdesign approaches; significant up-front expenses are involved when production facilities must be modified and these place the precaster at a disadvantage with respect to his competitors using cast-in-place construction.Yet there are indications that change will be required in the precastindustry's approach to earthquake-resistant design.1.1Precasters express a
growinginterestregions,andinexpanding their market into moreseismically activeseismic risk assessments for regions traditionally viewed asearthquake-free are being revised upward as seismologists continue to compileand evaluate geological and historical data.The ability to adapt to these new conditions may be important for thecontinued vitality of the precast buildings industry.While continued growthinto seismic regions may provide an opportunity for new profit, the prospectof increased seismic requirements in existing markets is the more urgentmotivation to quantify seismic demands and to develop cost-effective adaptations of existing technology.There is a need for experimental and analytical investigations into thedynamic behavior of "jointed" structures, in which gaps between precast elementsopenseismicand closeduring the response to ground shaking.design procedures must beRationaldeveloped which address the specificrequirements of precast concrete buildings.The nature and magnitude ofchanges required in existing precast connection details and modular framingsystems must be determined.While the attainment of these objectives willtake time, some of the results can be anticipated.As stated by Martin and Korkosz [1], issues of economy and suitability for service in seismic regions are intertwined.Much of the economy of precast prestressed concrete structural framing lies in its simplicity. It is best used in simplespan beams and deck members. The absence of continuityand redundancy has caused some designers to questionstability under high lateral loads.Due to lack of continuity, the precast systems most readily constructed tendto be less rugged or forgiving of extreme overload than typical cast-in-placestructures.To make sure that precast structures intended for service inseismic regions deliver all necessary capabilities as well as reduced cost (inI.other words, true economy), considerations of redundancy and ductility mustbe introduced in the design process."standardprecast,equipment"withTo the extent that these qualities arecast-in-placeand"extra-costoptions"withthe adoption of more rigorous design procedures could soften thecompetitive stance of precast concrete in zones of higher seismicity.On the other hand, rational procedures which enable prediction ofductility demand will facilitate the development and experimental validation of1.2
suitable connection details.Rather than emulating cast-in-place construction,designers of precast buildings for service in earthquake regions will be ableto develop competent lateral resisting systems which retain the economic andaesthetic benefits of precast concrete.Considerable research and develop-ment work will be required, but these steps appear essential to the greateracceptance of precast concrete construction in seismic regions.1.2TOWARD IMPROVED BUILDING CODE PROVISIONSBuilding code provisions for precast concrete, as for other of design,analysis,andproduction techniques against the knowledge obtained by experimentation andpractical application.betweendesignThus, progress depends upon effective ucers,andcodeauthorities.Improvementsin seismic code provIsIOns for cast-in-place concreteduring recent decades,for example, reflect major advances in seismology,structural dynamics, and empirical knowledge of the reversed cyclic, inelasticbehavior of reinforced concrete structures.productivity of the research community,dedication of design practitioners,This progress attests to thethe vitality of code bodies,theand the effectiveness of communicationamong the three groups.The research facilities and technical competence for producing recast concreteexist.Unfortunately, it appears that much of the current body of experimental dataregardingconnectionsforprecast structures hasbeen obtained throughprivately funded research and is held as proprietary.moneyspent forconnection testingseemsspecific short-term goals of the sponsor.Much of the privateto have been directed towardIf improved design procedures andcode provisions for precast structures are desired, improved communicationamong researchers, producers, designers, and code officials will likely accelerate their development.An important communications resource is the significant number ofresearchersandacademiciansConcrete Institute (PCl).amongthemembershipof thePrestressedThe Institute was founded in 1954 for the purpose1.3
of advancing the design,manufacture,anduseof prestressed,precastconcrete, and represents the prestressed concrete industry in the UnitedStates andCanada.Because all segmentsof the profession engaged inseismic-resistant design of precast concrete structures are represented in itsmembership,this organization is a logical forum for developmental efforts.PCl traditionally has engaged in activities that promote communicationamong members of the structural design and construction community.Theseinclude documenting the evolution of the "state of the art," disseminatingresearch findings to design practitioners, developing design standards andcodes of recommended practice, and participating in the development of building code recommendations.1.3BACKGROUND OF THE PRESENT RESEARCH EFFORTThis report was produced during Phase 2 of a three-part program,conceived by PCI and intended to advance the state of the art of estructures.ThePhase 1report [1], funded by the National Science Foundation (NSF) and preparedbythe ConsultingEngineersGroup,Inc.,presentsan overview of thecurrent state of the art.IncludedinthePhase 1 report are a discussion of basic seismicresponse concepts, a review of research findings on seismic ductility demandin cast-in-place concrete structures, design formulas for computing primaryload reinforcement at the connections of precast structures, and a compilationof the most commonly used connection details.The connections were evaluated by the precast industry with regardto simplicity, durability, and volume change accommodation.It is revealing ofthe current state, of the art that strength and ductility were not among thej'ratingcriteria.Althoughseismicductility demand is a topic of currentresearch, specific steps to assess ductility requirements and to ensure thatthey are satisfied in a particular structure have not thus far been incorporated in the design methodologies available to building engineers.InPhase 2,also funded by NSF,a rational methodology for thederivation of connector strength and ductility requirements has been developed, suitable connection details for representative example structures have1.4
been conceived,higherguidelines for adapting existing technology to regions ofseismicity have been formulated,testing of selected connectionpresents these developments.detailsand recommendations for physicalhavebeenprepared.This reportPhysical testing is to be conducted by othersin Phase 3.1.4OVERVIEW OF THE PROPOSED SEISMIC DESIGN METHODOLOGYSatisfactory seismic performance of a precast structure depends to agreat extent on fundamental issues of building configuration and framingscheme.Accordingly, as shown in Fig. 1.1, the design methodology whichhas been developed in the present research begins with the selection of asuitable lateral force resisting system, proceeds through the determination ofdesign loads and the estimation of global inelastic displacements during adamagingearthquake,andconcludes withan interpretation of kinematicproperties of the yielded lateral resisting system in terms of the deformationalrequirements for connectors in specific joints.Another important feature of the proposed technique is that it enablesa range of design strategies with varying degrees of reliance on inelasticaction.Hence, in the methodology developed here, the designer is offered achoice among possibilities which range from producing a ductile structure withyieldstrength significantly smaller than the "elastic strength demand" toproducing a high-strength structure which will experience reduced ductilitydemands during the largest earthquake anticipated at the site.Such anapproach could be advantageous for some types of construction (for example,low panelized buildings in regions of moderate seismicity) because it is sometimes easier, more reliable, and less expensive to provide xtraconnectionstrength than to provide a degree of ductility consistent with the reduceddesign force levels 'prescribed by typical building codes.In addition, the proposed approach enables the designer to computerational estimates of connection ductility and deformational compliance demandsin critical regions throughout the structure.This additional information canfacilitate the appropriate selection of structural layout, jointing strategy, andconnection details if the designer compares results obtained from alternativestructural configurations.1.5
.0Layout ".".", '.,m.'."'.'0"0'SllategyAddress VolumeChange Restraint.Select ModularPrecast ElementsJ- Identify LateralForce ReslltlngSystemSelsmoc ZoneJ,JDesign for UsualCode Forces andDuctility ReqmlsHSelect SelsmocResistancePhilosophyDesign for HigherSelect Trial Membe Forces and ReducedSizes & ConnectorDuctility ReQmtsConceptsCompute NaturalPeriod, TCompute "TC-3Elastic StrengthDemand (ESD)(Ie: R: 1)R ESDUSC FyVSASEESD -R----.,JT"sslgn Member& ConnectorStrengthsfCompute USCUltimate BaseShear (Fy)Select R Valuefor Design'--ESOUSC FyR Global ents ·p No. of CyclicReversals1bV (R';1)J.StrengthDuctility JPerform Kinematic"na'yals R'T & Seismic ZoneMore EffectiveSelamlc RealltantConnectionsFIGURE 1.1DESIGN METHODOLOGY FOR SEISMICRESIST ANT PRECAST CONCRETE BUILDINGS1.6
1.5OBJECTIVES OF THE PHASE 2 REPORTBeyond the presentation of an improved design methodology,thePhase 2 report is intended to explain seismic response of buildings in a waythat is useful to design practitioners having no formal training in earthquakeengineering or structural dynamics.AnotherobjectiveistoThis is the subject of Chapter 2.apply the methodology torepresentativeexamples of precast construction in the United States, illustrating the appropriate degree of analytical refinement and identifying inadequacies of presentknowledge concerning the behavior of jointed precast assemblages.Based onthe example structures, connection details and associated seismic performancecriteria havebeen proposed forphysicaltestingby others in Phase 3.Chapters 4 and 5 contain detailed applications of the proposed methodologypresented in Chapter 3.A final objective is to draw general conclusions for structures similarto those treated in the examples, to serve as interim guidelines for seismicresistant connection detailing before completion of the Phase 3 tests.Accord-ingly, a summary and conclusions are provided in Chapter 6.1.6TECHNICAL INPUT GROUPTo ensure that the Phase 2 study addressed the topics of greatestpractical concern across the country, the work was planned in cooperationwithaTechnicalDetails,TechnicalInput Group,Activities,composed of members of theandSeismiccommittees of theConnectionPrestressedConcrete Institute.The Technical Input Group was asked to propose examples for study.It was agreed that a candidate structure should represent a type of construc-tion which provides large public exposure to structural precast concrete,considering building size, occupancy, and frequency of occurrence across thecountry.Further, the structure should be of a type which offers significantmarket potential for precast concrete in seismically active regions if presentdesign, performance, and acceptance limitations are overcome.Using these criteria, a seven-story precast concrete parking structure based on the Metro-Space building system and a hypothetical 17-story1.7
bearing wall apartment building designed by the PCI Bearing Wall Committeewere selected.1. 7INDUSTRY AWARENESS AND PARTICIPATION ARE VITALSome of the most important information concerning the adequacy ofseismic design and analysis methods is gained by surveying the performanceof structures which have been subjected to earthquakes.Damaging earth-quakes, however, are few and far between, so this information accumulatesslowly.Further, these "experiments" performed in the "real-world labora-tory" seldom are planned to maximize the value of the information gained.Seismic design details tend to receive attention in proportion to a structure'ssize,cost, importance, and seismic zone.Because the number of "minor"structures greatly exceeds the number of "major" structures constructed ofprecast concrete, the typical earthquake victim tends to be a "minor" structure not designed for seismic resistance.Although precast structures seemto have fared well in earthquakes in the past, these observations suggestthat the seismic behavior of precast structures in future earthquakes mayconvey little information on the adequacy of state-of-the-art technology forearthquake-resistant connection proach and attentiontoseismic-resistant detailing in the design of precast concrete structures, largeand small.This will increase the opportunities for observing and evaluatingstate-of-the-art connection performance, enhance the credibility of precast inseismic regions, and accelerate the evolution of connection design technology.These steps are appropriate given the current state of knowledge,but it is important to see the present effort as a waypoint on a longerjourney.Many important questions remain unanswered:oWhat is the effect of elastic nonlinearities, due to joints whichopen and close during an earthquake, on the seismic responseamplitude of a jointed precast structure?Do traditional seismicloading criteria, developed for monolithic structures, apply?oWhen assessing the fundamental period of a jointed precast structure for computing code seismic forces, is it necessary to model1.8
joint flexibility realistically / or is it sufficient to approximate theconnections as either fixed or pinned?If realistic modeling isessential/experimental data or reliable predictive techniques notpresently available must be obtained.oCurrent seismicdesigncodespresume monolithic behavior andenergy dissipation involving large volumes of material.Is it validto use code forces for jointed precast construction in which relatively small volumes of material yield?Precast buildingsarebeingconstructedin earthquake zones andthere is immediate need for seismic design guidelines.This means / for thetime being / that answers to some important questions must be assumed.questionswill arise astoday'squestions are answered but,Newin time / animproved understanding will evolve.Technological evolution is iterative / involving hypothesis / experimentation / evaluation / and deduction.In the case of seismic-resistant design /evolution is the product of effective communication between industry / government/ the research community / and private consulting practice, motivated bya shared commitment to earthquake safety.of technological evolution in progress.1.9This report is offered as evidence
CHAPTER 2PRECAST CONCRETE CONSTRUCTIONVERSUS EARTHQUAKES2.1INTRODUCTIONWhile the building design profession shows a growing awareness ofthe need for an effective and practical response to seismic hazardsIengi-neering seismology and structural dynamics are beyond the scope of mostundergraduate civil engineering programs; the majority of engineers are firstexposed to the questions of earthquake resistance only after entering professional practice.On the other handIof mathematics and physics.all engineers are familiar with the basic principlesWith these toolsIa solid conceptual understand-ing of building code seismic provisions and the structural requirements forearthquake safety can readily be achieved.Such understanding will signifi-cantly help many engineers responsible for the design of regular structuresin regions of low to moderate seismicity who want to know that their interpretations of code requirements are correctIand who seek greater confidencein their ability to select the design alternatives best suited to earthquakeresistance.This chapter is intended to provide a conceptual understanding of thesources of earthquake ground motions and the earthquake be)1.avior of buildings.Building code seismic provisions are explained.Important differencesin the seismic behcivior of "jointed" and "monolithic" structures are described.Specific considerations in the planningIanalysisIand detailing of seismic-resistant precast concrete structures are presented in the design methodologyof Chapter 3Iwhich is then applied to practical examples in Chapters 4 and5.Though the material presented here can improve the effectiveness ofbuilding designers who lack academic training and a depth of experience in2.1
earthquake engineering, it is neither precise nor complete.Formally trainedspecialists should be consulted in the planning and design of large, irregular,or unusual seismic-resisting structures.2.2THE ORIGINATION AND PROPAGATIONOF SEISMIC GROUND anbestbedescribed in terms of energy, its changes from one physical manifestation toanother, and its propagation through time and space.Energy may be con-sidered to occur in either of two conditions, active or passive.Energy in its passive state can be difficult to recognize.A frozenblanket of snow in the high mountains bears no outward resemblance to thesun's heat which drives the evaporative process and the storms, causing anupward migration of water against the gravitational field.Yet, a snowfieldhigh above sea level can be regarded as a passive reservoir of stored solarenergy.When this energy bank is unlocked by warmer temperatures in thespring, the energy is transformed to the active state; the waters rush downward, moving rocks and boulders in the streams, eroding river banks, andspinning the turbines in hydroelectric generating plants.Energy in the passive state is referred to as potential energy, or thestored capacity to perform work.The coiled spring in a clock, the altitudeof an airplane above the ground, and the fuel in its tanks are examples ofpotential energy.Ene
4.9.2 Connections Intended to Ride Along with Deformations of the Lateral Force Resisting System 4.9.3 Other Locations 4.10 Summary . EXAMPLE 2 --BEARING WALL APARTMENT BUILDING . . . . 5.1 Description of Structure. 5.2 Concept for Lateral Load Resistance . 4.18 4.18 4.18 4.19 4.30 4.34 4.