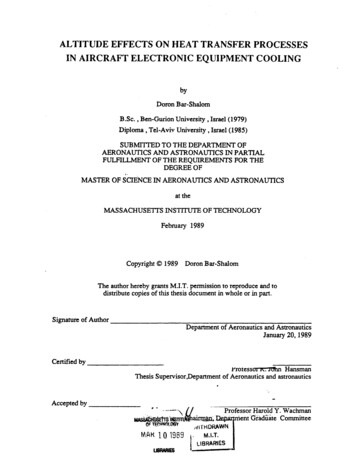
Transcription
ALTITUDE EFFECTS ON HEAT TRANSFER PROCESSESIN AIRCRAFT ELECTRONIC EQUIPMENT COOLINGbyDoron Bar-ShalomB.Sc., Ben-Gurion University, Israel (1979)Diploma , Tel-Aviv University , Israel (1985)SUBMITTED TO THE DEPARTMENT OFAERONAUTICS AND ASTRONAUTICS IN PARTIALFULFILLMENT OF THE REQUIREMENTS FOR THEDEGREE OFMASTER OF SCIENCE IN AERONAUTICS AND ASTRONAUTICSat theMASSACHUSETTS INSTITUTE OF TECHNOLOGYFebruary 1989Copyright 1989 Doron Bar-ShalomThe author hereby grants M.I.T. permission to reproduce and todistribute copies of this thesis document in whole or in part.Signature of AuthorDepartment of Aeronautics and AstronauticsJanuary 20, 1989Certified by'rotessorux-,.n HansmanThesis Supervisor,Department of Aeronautics and astronauticsAccepted byProfessor Harold Y. Wachman"" M " Ib(OlaientOF TE.H.N.,V1THDRAWNMIAR 10 1989UBRMIESA.-.M.I.T.I LIBRARIESGradiulate Committee
ALTITUDE EFFECTS ON HEAT TRANSFER PROCESSESIN AIRCRAFT ELECTRONIC EQUIPMENT COOLINGbyDoron Bar-ShalomSubmitted to the Department of Aeronautics and Astronautics onJanuary 20, 1989 in partial fulfillment of the requirements for the degree ofMaster of Science in Aeronautics and Astronautics.AbstractAltitude dependent changes of aircraft heat transfer processes in electronic equipmentboxes in equipment bays were investigated to examine the compatibility of currentspecifications for avionics thermal design with the thermal environment encountered inhigh performance aircraft.Steady state equipment and bay air temperature were analyzed as a function ofaltitude based on known sea level thermal conditions and design parameters, by usingstandard atmospheric models and aircraft altitude Mach number flight envelope. Thisanalysis was used to generate temperature-altitude envelopes which give the temperature ofthe equipment and the bay air as a function of altitude, based on typical altitude profiles ofthe bay wall temperature. Analysis of an unconditioned bay, containing ambient cooledequipment, was conducted. The optimal temperature difference between the equipment andthe bay wall was identified. Analysis of a conditioned bay, containing both ambient andforced-air cooled avionics, showed a tendency towards isothermal bay air temperaturealtitude profiles as the fraction of forced-air cooling was increased. The results showed thatthe temperature difference between the equipment and the bay wall grows exponentiallywith altitude in natural convective cooling and can be approximated as a function of theexternal pressure only. Radiation heat transfer was shown to serve as a "thermal pressurerelief valve" and to improve the thermal performance of the system at high altitude.The isothermal tendency of the bay air in a conditioned bay implies that ambientcooled equipment designed in accordance with MIL-E-5400 would not be compatible withthe bay environment and additional cooling would be required. The results of this thesisprovide guidance in determining the thermal design parameters which improve altitudeperformance of the avionics cooling system and in identifying the flight conditions resultingin critical thermal conditions.Thesis Supervisor:Title:Professor R. John HansmanAssociate Professor of Aeronautics and Astronautics
AcknowledgementsFirst, I would like to thank Professor RJ. Hansman, my advisor, for providing"guidance and control" over the past eighteen months, and for offering valuableadvice and criticism, as well as a warm working atmosphere despite the surroundingicing conditions. I would also like to thank Mr. Jerry Hall of McAir for the valuabledata he has provided, and for the time we spent discussing and sharing his extensiveexperience in the field of avionics cooling. I would also like to thank Mr. CharlesLeonard of Boeing for sharing his ideas and test data in the area of passive cooling,introducing an additional important point of view. To the friends in the AeronauticalSystems Lab, who have provided lots of friendship and fun: may your effort fly upand away.I would like to express my appreciation to my parents, Rachel and Avigdor,who have had their fingers crossed for the past several months while waiting "in thedark" across the sea. To my wife Edna: there is not enough space to express thegratitude you deserve for being so patient, understanding, and most of all for caring.Last but certainly not least, to our children Liron and Zohar, who have left their littlefriends behind and come to see the big world: I hope that the huge pile of scrap paperwill give you a chance to express your artistic talents.
Table of ContentsAbstractAcknowledgementsTable of ContentsList of FiguresNomenclature234691. Introduction1.1 Overview12122. Avionics Cooling2.1 Background2.2 The Importance of Avionics Thermal Control2.3 The Optimization Problem2.4 The Cooling Problem2.5 The Avionics Bay Environment2.5.1 The External Atmospheric Environment2.5.1.1 Atmospheric Temperature Model2.5.1.2 Atmospheric Pressure Model2.5.1.3 Atmospheric Humidity Model2.5.2 Aerodynamic Heating2.5.3 Aircraft Thermal Zones2.6 Avionics Cooling Techniques2.6.1 Ambient Cooled Equipment2.6.1.1 Cooling Requirements2.6.2 Forced-Air Cooled Equipment2.6.2.1 Cooling Requirements2.7 Environmental Control System1414181920222425252529313336384141433. Unconditioned Bay Configuration - Ambient Cooled Avionics3.1 Introduction3.2 Modes of Heat Transfer3.2.1 Dependence of Radiation and Convection Parameters on Altitudeand Configuration3.2.1.1 Convective Heat Transfer Coefficient3.2.1.2 Radiative Heat Transfer Coefficient3.3 Results3.3.1 Equipment Temperature Versus Altitude For a Single SegmentConvection Path System Configuration3.3.2 Equipment Temperature Versus Altitude for a Single SegmentConvection and Radiation Paths System Configuration3.3.3 Equipment Temperature Versus Altitude for a Double SegmentConvection and Radiation Paths System Configuration464646524. Conditioned Bay Configuration - Ambient and Forced-Air CooledAvionics4.1 Introduction92525456566881
-5-4.24.34.44.5Thermal Configuration of a Conditioned Avionics BayControl Volume Analysis For a Conditioned BayAnalysis of Altitude Dependent Effects on Bay TemperatureResults5. Summary and Conclusions5.1 Summary5.2 Conclusions and Implications9297101101108108110
List of FiguresFigure 2-1: Aircraft growth curve magnifies effects of weight increments[16]Figure 2-2: Trends in cabin and avionics heat load and aircraft mass [14].Figure 2-3: Illustration of order of aircraft penalty of an E.C.S. designedto cool 30 KW [14]Figure 2-4: Thermal acceleration factor for bipolar digital devices [1].Figure 2-5: Example - The influence of temperature on componentreliability (PNP Silicon transistor)Figure 2-6: Electronic component temperature buildupFigure 2-7: Illustration of responsibilities of aircraft and avionicsdesigners for thermal designFigure 2-8: Hot and cold atmosphere models - Temperature vs. altitude[23]Figure 2-9: Atmospheric model - Pressure vs. altitude [23]Figure 2-10: Atmospheric model - Design moisture conditions [23]Figure 2-11: Adiabatic wall air temperature versus Mach number andaltitudeFigure 2-12: Typical flight envelope of modem fighter aircraftFigure 2-13: Typical adiabatic wall temperature profile of modem fighteraircraftFigure 2-14: Typical aircraft thermal zones [7]Figure 2-15: Typical electronic equipment bay arrangements [10]Figure 2-16: Ambient cooled and forced-air cooled avionicsFigure 2-17: Temperature rise per unit heat flux vs. convective heattransfer coefficientFigure 2-18: Temperature-altitude operational requirements -- bay airtemperature versus altitude, MIL-E-5400 Class II [21]Figure 2-19: Forced-air cooled avionics cooling requirements - airflowvs. cooling air temperatureFigure 2-20: Environmental control system schematicFigure 2-21: Typical ECS cooling air temperature control scheduleFigure 3-1: Ambient cooled equipment: modes of heat transfer andthermal resistors modelFigure 3-2: Natural convection - avionics units vs transition to turbulentFigure 3-3: Single convection segment configuration schematicFigure 3-4: Numerical analysis flow diagramFigure 3-5: Effects of bay wall temperature and temperature difference onthe changes of (W 86062with altitude in a single segmentconvection7 ath configuration)ew ,'versus altitude, as a function of pressure onlyFigure 3-6: (Figure 3-7: Equipment temperature simulation based on MIL-E-5400class II temperature altitude envelope for a single segment convectionpathFigure 3-8: Single convection segment and radiation configurationschematic646670
Figure 3-9: Numerical analysis flow diagram for single convection andradiation systemFigure 3-10: Effects of radiation factor a, on the changes of ( Tew)at'with altitudeFigure 3-11: Equipment temperature simulation based on MIL-E-5400class H temperature altitude envelope for a single segment convectionpath and radiation, radiation factor a 0.01Figure 3-12: Equipment temperature simulation based on MIL-E-5400class H temperature altitude envelope for a single segment convectionpath and radiation, radiation factor a O0.1Figure 3-13: Equipment temperature simulation based on MIL-E-5400class II temperature altitude envelope for a single segment convectionpath and radiation, radiation factor a z1.0Figure 3-14: Equipment temperature simulation based on MIL-E-5400class II temperature altitude envelope for a single segment convectionpath and radiation, radiation factor a 10.0Figure 3-15: Equipment temperature simulation based on MIL-E-5400class II temperature altitude envelope for a single segment convectionpath and radiation, radiation factor rc 100.0Figure 3-16: Optimal temperature difference versus radiation factor aFigure 3-17: Double convection segment and radiation configurationschematicFigure 3-18: Numerical analysis flow diagram for double convection andradiation systemFigure 3-19: Effects of convection balance factor, y, on the changes of( ( Tw)ak\7173757677787980828486with altitude (without radiation)y for the system considered87in Fig. 3-19Figure 3-21: Effects of convection balance factor, y, on the changes ofin a system with radiation factor a 0.0188Figure 3-20: The changes in(ATewa))P,1/4versuswith altitudeFigure 3-22: Effects of convection balance factor, y, on the changes offactor a 0.1( A(T )S with altitude in a system with radiationFigure 3-23: Effects of convection balance factor, y, on the changes ofu with altitude in a system with radiation factor a 1.0( &Tewa"89Figure 3-24: Effects of convection balance factor, y, on the changes of91(ATew)st )((ATew )j)90with altitude in a system with radiation factor a 10.0Figure 4-1: Thermal configuration of a conditioned bay -- Ambient andforced-air cooled avionicsFigure 4-2: Control volume analysis for a conditioned avionics bay.Figure 4-3: Numerical analysis flow diagram for conditioned bayconfigurationFigure 4-4: The changes of bay air temperature with altitude for constantwall temperatureFigure 4-5: Bay air temperature vs. altitude for a simple temperaturealtitude profile as the wall temperature9398102104105
-8Figure 4-6: Bay air temperature vs. altitude and forced-air cooled heatload ratio in comparison with MIL-E-5400106
NomenclatureaACCdPFrghch,H hAkLmmM u/anPpqq" q/ArRst, TyLocal velocity of sound, [m/sec]Surface area, [m2]ConstantSpecific heat at constant pressure, [J/Kg"K]Diameter,[m]Radiation heat transfer factor, defined by equation (3.13), [W/OK 4 ]Acceleration of gravity, [m/s2 ]Average convective heat transfer coefficient, [W/m2 C]Average radiative heat transfer coefficient, [W/m2 "C]Heat transfer conductance factor (1/R), [W/oC]Thermal conductivity, [W/moC]Length, [m]mass, [Kg]Mass flow rate, [Kg/sec]Mach numberExponent - used for Rayleigh number in free convection 1/4 for laminar flow 1/3 for turbulent flowPressure - absolute, [N/m2 ]Pressure - atmospheres, [dimensionless]Heat transfer rate, [W]Heat flux, [W/m2 ]Recovery factor, defined by equation (2.5), [dimensionless]Thermal resistance, [OC/W]A characteristic dimension (in conduction path), [m]Absolute temperature, [OK]Elevation - altitude, [Ft]Dimensionless GroupsBi hskBiot modulusGrx p2pgrpGrashof numberI2Gr. p2 q-"x4Modified Grashof number for uniform heat flux (q")N, -Forced-air cooling influence numberhxxNux -h"Local Nusselt numberhLLNuL -"kAverage Nusselt number
-10-Prandtl numberPr CPk3RaL - spaOLRayleigh numberRa , Ra.,vkModified Rayleigh number for uniform heat flux (q")Greeka Radiation factor, Eq.E (3.7)a kThermal diffusivity, [m 2/sec](3Volumetric expansion coefficient, [oK-'],( 1/T for ideal gasses)Convection balance factor, Eq. (3.7)Normalized convective heat transfer coefficient, Eq. (3.7).Y6, he lt)8.ATp.pvaThermal boundary layer thickness, [m]Temperature difference, ["C]Dynamic viscosity of air, [Nsec/m 2 ] [Kg/msec]Density of air, [Kg/m 3]Kinematic viscosity of air, [m2/sec]Stefan - Boltzmann constantSuperscripts(C)()"( )Per unit time, [sec- ']Per unit area, [m- 2]Normalized parameter, P Paameter at atitdreParameter at sea levelSubscripts()alt()a()ambEvaluated at altitudeRefers to ambient temperature(),()bAdiabatic wall conditionsEvaluated at bulk temperatureConductionConvectionBased on diameterRefers to equipment surface temperatureRefers to the external airEvaluated at the film temperature, given by equation utInSummation conventionBased on length of plateMean flow conditionsOut
-11-()r()so)w()x()o()o(LRadiationEvaluated at sea levelRefers to wall temperatureLocal valueDenotes reference conditions(usually ambient pressure and temperature at sea level)Denotes stagnation flow conditionsEvaluated at free stream conditions
Chapter 1Introduction1.1 OverviewThe objective of this thesis is to examine the compatibility of the currentspecifications for avionics thermal design with the thermal environment encounteredin modem high performance jet aircraft.A subsequent
temperature versus altitude, MIL-E-5400 Class II [21] Figure 2-19: Forced-air cooled avionics cooling requirements -airflow 42 vs. cooling air temperature Figure 2-20: Environmental control system schematic 44 Figure 2-21: Typical ECS cooling air temperature control schedule 45 Figure 3-1: Ambient cooled equipment: modes of heat transfer and 47 thermal resistors model Figure 3-2: Natural .