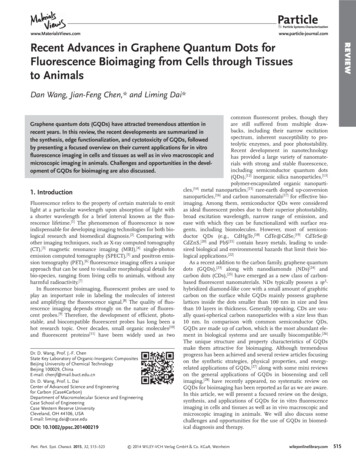
Transcription
EWRecent Advances in Graphene Quantum Dots forFluorescence Bioimaging from Cells through Tissuesto AnimalsDan Wang, Jian-Feng Chen,* and Liming Dai*common fluorescent probes, though theyare still suffered from multiple drawbacks, including their narrow excitationspectrum, inherent susceptibility to proteolytic enzymes, and poor photostability.Recent development in nanotechnologyhas provided a large variety of nanomaterials with strong and stable fluorescence,including semiconductor quantum dots(QDs),[12] inorganic silica nanoparticles,[13]polymer-encapsulated organic nanoparticles,[14] metal nanoparticles,[15] rare-earth doped up-conversionnanoparticles,[16] and carbon nanomaterials[17] for effective bioimaging. Among them, semiconductor QDs were consideredas ideal fluorescent probes due to their superior photostability,broad excitation wavelength, narrow range of emission, andease with which they can be functionalized with surface reagents, including biomolecules. However, most of semiconductor QDs (e.g., CdHgTe,[18] CdTe@CdSe,[19] CdTeSe@CdZnS,[20] and PbS[21] contain heavy metals, leading to undesired biological and environmental hazards that limit their biological applications.[22]As a recent addition to the carbon family, graphene quantumdots (GQDs),[23] along with nanodiamonds (NDs)[24] andcarbon dots (CDs),[25] have emerged as a new class of carbonbased fluorescent nanomaterials. NDs typically possess a sp3hybridized diamond-like core with a small amount of graphiticcarbon on the surface while GQDs mainly possess graphenelattices inside the dots smaller than 100 nm in size and lessthan 10 layers in thickness. Generally speaking, CDs are usually quasi-spherical carbon nanoparticles with a size less than10 nm. In comparison with common semiconductor QDs,GQDs are made up of carbon, which is the most abundant element in biological systems and are usually biocompatible.[26]The unique structure and property characteristics of GQDsmake them attractive for bioimaging. Although tremendousprogress has been achieved and several review articles focusingon the synthetic strategies, physical properties, and energyrelated applications of GQDs,[27] along with some mini reviewson the general applications of GQDs in biosensing and cellimaging,[28] have recently appeared, no systematic review onGQDs for bioimaging has been reported as far as we are aware.In this article, we will present a focused review on the design,synthesis, and applications of GQDs for in vitro fluorescenceimaging in cells and tissues as well as in vivo macroscopic andmicroscopic imaging in animals. We will also discuss somechallenges and opportunities for the use of GQDs in biomedical diagnosis and therapy.Graphene quantum dots (GQDs) have attracted tremendous attention inrecent years. In this review, the recent developments are summarized inthe synthesis, edge functionalization, and cyctotoxicity of GQDs, followedby presenting a focused overview on their current applications for in vitrofluorescence imaging in cells and tissues as well as in vivo macroscopic andmicroscopic imaging in animals. Challenges and opportunities in the development of GQDs for bioimaging are also discussed.1. IntroductionFluorescence refers to the property of certain materials to emitlight at a particular wavelength upon absorption of light witha shorter wavelength for a brief interval known as the fluorescence lifetime.[1] The phenomenon of fluorescence is nowindispensable for developing imaging technologies for both biological research and biomedical diagnosis.[2] Comparing withother imaging techniques, such as X-ray computed tomography(CT),[3] magnetic resonance imaging (MRI),[4] single-photonemission computed tomography (SPECT),[5] and positron emission tomography (PET),[6] fluorescence imaging offers a uniqueapproach that can be used to visualize morphological details forbio-species, ranging from living cells to animals, without anyharmful radioactivity.[7]In fluorescence bioimaging, fluorescent probes are used toplay an important role in labeling the molecules of interestand amplifying the fluorescence signal.[8] The quality of fluorescence imaging depends strongly on the nature of fluorescent probes.[9] Therefore, the development of efficient, photostable, and biocompatible fluorescent probes has long been ahot research topic. Over decades, small organic molecules[10]and fluorescent proteins[11] have been widely used as twoDr. D. Wang, Prof. J.-F. ChenState Key Laboratory of Organic-Inorganic CompositesBeijing University of Chemical TechnologyBeijing 100029, ChinaE-mail: chenjf@mail.buct.edu.cnDr. D. Wang, Prof. L. DaiCenter of Advanced Science and Engineeringfor Carbon (Case4Carbon)Department of Macromolecular Science and EngineeringCase School of EngineeringCase Western Reserve UniversityCleveland, OH 44106, USAE-mail: liming.dai@case.eduDOI: 10.1002/ppsc.201400219Part. Part. Syst. Charact. 2015, 32, 515–523 2014 WILEY-VCH Verlag GmbH & Co. KGaA, Weinheimwileyonlinelibrary.com515
om2. Synthesis and Edge Functionalization of GQDsfor Toxicology Studies2.1. SynthesisCommercial applications of GQDs will not be realized if thereis no facile and large-scale fabrication capability for GQDswith a well-defined size and shape. To date, various syntheticapproaches have been developed for the synthesis of GQDs,which can be classified into two strategies. One is the top-downapproach involving the cleavage of carbonaceous materials viaphysical, chemical, or electrochemical processes. For instance,various carbon materials, including graphite, graphene, graphene oxides, carbon nanotubes, carbon fibers, carbon black,and even coal have been used as starting materials for the synthesis of GQDs via acidic oxidation, hydrothermal treatment,electrochemical exfoliation, to name a few.[27] Alternatively,GQDs can be synthesized via a bottom-up approach, involvingsolution chemistry, cyclodehydrogenation of polyphenylene precursors, or carbonizing certain organic precursors.[28]The optical properties of GQDs are the basis of application in in vitro and in vivo imaging. Different synthetic oftenlead to GQDs with different sizes, functional groups, andedge defects, which play important roles in regulating opticalproperties. GQDs usually show typical absorption bands inthe UV region, with an absorption peak around 230 nm dueto the π–π* transition of aromatic sp2 domains and a long tailextending into the visible range.[29] However, another broadband over 270–390 nm associated with the n–π* transition ofC O could also appear.[30] More importantly, most of the GQDsreported in the literature exhibit photoluminescence emissions,which make them useful for fluorescent bioimaging. Till now,GQDs with a wide range of PL emission colors from ultravioletto near-infrared have been synthesized via various approaches(Figure 1). Some of the GQDs are reported to even possessupconversion photoluminescence properties[27,28,31] attractivefor multi-photon-induced bioimaging.[32] For detailed discussions on the various synthetic approaches to GQDs GQDs andtheir optical properties, the interested readers are referred tospecialized review articles.[27,28] Here, we give only a brief summary in Table1[33–46] while the photoluminescence of GQDs fordifferent bioimaging applications will be described in the subsequent sections.Dan Wang is a postdoctoralfellow in State Key Laboratory of Organic–InorganicComposites, at BeijingUniversity of Chemical Technology. He received a B.Eng.degree in Material Scienceand Engineering and aPh.D. degree in OpticalEngineering from ZhejiangUniversity, in 2008 and2013, respectively. Currently,he is a visiting scholar in Prof. Liming Dai’s group at CaseWestern Reserve University. His research interests focuson the synthesis, surface functionalization, and bio-relatedapplications of nanomaterials.Jian-Feng Chen is a CheungKong Distinguished Professorand Director of State Key Laboratory of Organic–InorganicComposites, Dean of College ofChemical Engineering, BeijingUniversity of Chemical Technology (China); He receiveda B.Eng. degree and a Ph.D.degree in Chemical Engineeringfrom Zhejiang University in1986 and 1992, respectively. Hewas a visiting full professor at Case Western Reserve University from 1997 to 1998. His research interests involveprocess intensification, nanomaterials, and nanodrugdelivery systems.Liming Dai is a Kent HaleSmith Professor in Department of Macromolecular Science and Engineering at CaseWestern Reserve University. Heis also director of the Centerof Advanced Science andEngineering for Carbon. Beforejoining the CWRU, he was anassociate professor of polymerengineering at University ofAkron and the Wright BrothersInstitute Endowed Chair Professor at University of Dayton.He is a Fellow of Royal Society of Chemistry and Fellow ofAmerican Institute for Medical and Biological Engineering.His expertise covers the synthesis, functionalization, andfabrication of conjugated polymers and carbon nanomaterials for energy- and biodevice applications.2.2. Edge Functionalization for Toxicology StudiesFigure 1. Different emission spectra of graphene quantum dots. Reproduced with permission.[37b] Copyright 2013, Royal Society of Chemistry.516wileyonlinelibrary.comGQDs synthesized by the above-mentioned approaches oftencontain various hydrophilic groups (e.g., carboxylic, hydroxy, 2014 WILEY-VCH Verlag GmbH & Co. KGaA, WeinheimPart. Part. Syst. Charact. 2015, 32, 515–523
EWTable 1. A brief summary of the emission of GQDs via typical syntheticstrategies.MethodsAcidic oxidationHydrothermalElectrochemistryStepwise solution chemistryPrecursor pyrolysisStarting materialsEmissionRefs.GraphiteBlue, green[33]GrapheneBlue[34]GOBlue, green, yellow[35]Carbon nanotubesblue[36]Carbon fibersBlue to near-IR[37]Carbon blackBlue, green, yellow[38]CoalBlue, green, yellow[38]GOBlue, green, nic precursorsRed[43]Citric acidBlue[44]GlucoseDUV, blue[45]L-glutamic acidBlue, green, yellow[46]and amino), and thus could be dispersed well in water. Theseedge functional groups could not only improve the biocompatibility of GQDs, but also allow GQDs for covalent bonding withbiomolecules for bio-recognition in bio-systems. In this context,Zheng et al.,[38] have synthesized insulin-conjugated GQDsfor specific labeling and dynamic tracking of insulin receptorsin 3T3-L1 adipocytes. On the other hand, Zhu et al.,[47] prepared various edge functionalized GQDs by connecting GQDswith alkylamines (m-GQDs) or reducing GQDs with NaBH4(r-GQDs) (Figure 2a), and found a very low toxicity to MC3T3cells for both GQDs and r-GQDs with a distinct dose-dependentcell viability for m-GQDs (Figure 2b). These results also supportthe notion that the edge functionalization plays a critical role inthe cytotoxicity of GQDs. In addition, Nurunnabi et al.,[26b] performed both in vitro and in vivo toxicity evaluations on carboxylated GQDs prepared from carbon fibers. In the in vitro toxicityevaluations, the GQDs (up to 500 µg mL 1) were introduced toKB, MDA-MB231, A549, and MDCK cells, and were found tonot impose considerable toxicity to the selected cell lines. Inthe in vivo toxicity evaluations, no obvious organ damage orlesions were observed for the mice after 21 d of administrationof GQDs at 5 mg kg 1 or 10 mg kg 1 dosages (Figure 3). By preparing nitrogen-doped GQDs (N-GQDs) from GO, Liu et al.,[48]performed co-incubation of HeLa cells with N-GQD for 12 and24 h, respectively, and found that different doses of N-GQDs(5–400 µg mL 1) did not weaken the cell activity compared withthe control group.Apart from cytotoxicity studies of cells and animals, the genotoxicity of GQDs is also very important to evaluate the potentialhazards of GQDs to biological systems because there is a closecorrelation of DNA damage with mutation and cancer. It hasbeen demonstrated that carbon nanotubes,[49] nanodimonds,[50]and graphene oxides[51] could cause DNA damage even whenthey showed a low toxicity to cells. However, no study on thegenotoxicity of GQDs has been reported so far. Therefore, morecomprehensive and thorough cytotoxicity and genotoxicitystudies are needed to exploit the full potentials of GQDs forPart. Part. Syst. Charact. 2015, 32, 515–523Figure 2. a) Scheme of preparation routes (suggested struct ures).m-GQDs are obtained by connecting with alkylamines, r-GQDs areobtained by reducing GQDs using NaBH4. b) Effect of GQDs, m-GQDs,and r-GQDs on MC3T3 cells viability. Reproduced with permission.[47]biomedical applications, though GQDs are more biocompatiblethan other fluorescence probes, such as semiconductor QDs.3. GQDs for In Vitro Cell ImagingThe photoluminescence is one of the fascinating featuresof GQDs that makes them attractive for bioimaging. Consequently, considerable research efforts have been dedicatedto exploit GQDs as fluorescent probes for in vitro and in vivoimaging. For instance, Zhu et al.,[52] have prepared GQDs withstrongly green fluorescence emission from graphene oxides viaone-step solvothermal method (Figure 4a). The resultant GQDswere demonstrated to exhibit a good biocompatibility, whichcould be used as excellent biolabeling agents for imaging cells(Figure 4b).Using polycyclic aromatic hydrocarbon as the precursor,Zhou et al.[53] synthesized GQDs via a bottom-up approach. Theresultant GQDs were then efficiently internalized by MCF-7 2014 WILEY-VCH Verlag GmbH & Co. KGaA, Weinheimwileyonlinelibrary.com517
omone-photon-excited fluorescence, the fluorescence emission is usually induced by UV orblue excitation, which are harmful to livingcells or bio-systems. Therefore, the multiphoton induced fluorescence of GQDs hasbeen studied for cellular imaging. In particular, Zhu et al.[47] performed two-photonfluorescence imaging of MC3T3-E1 cells,using GQDs as fluorescent probes and nearIR excitation (Figure 7). Using nitrogendoped GQDs with a two-photon absorptioncross-section as high as 48 000 GM as twophoton probes, Liu et al.,[48] have obtaineda large imaging depth of 1800 µm in tissuephantom (Figure 8).Figure 3. Histology study to measure toxicity. Histological evaluation of the major organs ofthe mice at 22 d after intravenous injection of the GQDs. No symptoms of inflammation and/or lesion were observed in the images. Tissues of 5 and 10 mg kg 1 GQDs treated mice aresimilar with that of tissues of saline-treated mice. Images were taken at 160 magnification withhematoxylin and eosin staining. Reproduced with permission.[26b] Copyright 2013, AmericanChemical Society.cells and accumulated in the cytoplasm (Figure 5a). Comparedwith Alexa fluo 488 and fluorescein that are commonly usedfluorescence probes for cellular imaging, these GQDs exhibiteda much higher photostability (Figure 5b).By conjugating insulin with GQDs, Zheng and co-workersrevealed insulin receptor dynamics in live cells using a totalinternal reflection fluorescence microscopy (TIRFM).[38] Theseauthors observed small discrete clusters of GQDs under TIRFMafter pre-incubating adipocytes with insulin-GQDs (Figure 6a)and tracked the constant lateral movement of GQD-enlightenedclusters to the cell membrane and vertical movement betweenthe inner cytosol and the plasmalemmal region by followingthe GQDs fluorescence. Based on the real-time tracking of individual clusters during the imaging period, four subpopulationswere identified (Figure 6b–e), demonstrating great potential ofthe edge functionalized GQDs in cell imaging, particularly forinvestigating dynamic cellular processes.The basis for the use of GQDs as fluorescent probes forin vitro imaging in above-mentioned and other studies[54] isthe one-photon-excited photoluminescence emission. In theFigure 4. a) PL (at 375 nm excitation) spectra of the GQDs aqueous solution (inset: photograph taken under UV light). b) Fluorescence imaging ofMG-63 cells treated with GQDs (at 405 nm excitation). Reproduced withpermission.[52] Copyright 2011, Royal Society of Chemistry.518wileyonlinelibrary.com4. GQDs for In Vivo Imagingof AnimalsIn most cases of fluorescence imaging foranimals, significant background signal associated with autofluorescence of tissues of theanimals is observed.[55] The short-wavelengthlight (either the excitation light or the emission light) couldalso be easily absorbed by water in the biological tissues andscattered due to the Rayleigh scattering effect, which is notdesirable for animal imaging. Thus, the quality of fluorescenceimaging for small animals depends strongly on the emissionwavelength of fluorescent probes. Following an intravenousinjection of carboxylated GQDs with green fluorescence, Nurunnabi et al.,[26b] performed in vivo fluorescence imaging of mice,in which the fluorescence signal of GQDs was observed at thetumor site at 12 h post-injection, indicating that GQDs could beused for superficial tissue imaging to detect, for example, skincancer (Figure 9). However, the fluorescence signal of GQDs inthe deep tissue or organs, such as heart, liver, or spleen, couldnot be detected in vivo due to the short-wavelength excitationand emission of the GQDs used in this particular case. WhenGQDs with near infra-red photoluminescence were used for invivo imaging, however, the near infra-red fluorescence signalFigure 5. a) Merged image of fluorescence imaging and bright-fieldimaging of MCF-7 cells after incubation with GQDs for 2 h. b) Timedependent fluorescence intensity ratio (I/I0) of P-GQDs, Alexa fluo 488,and fluorescein. I0 and I are the emission intensities of GQDs, Alexa fluo488, and fluorescein without and with laser illumination for different time,respectively. Reproduced with permission.[53] 2014 WILEY-VCH Verlag GmbH & Co. KGaA, WeinheimPart. Part. Syst. Charact. 2015, 32, 515–523
EWFigure 6. Tracking the dynamics of insulin receptors in living adipocytes using TIRFM. a) Typical TIRFM image of a 3T3-L1 adipocyte after 1 h incubation of insulin-GQDs. Scale bar 5 µm.b) Membrane patch consisting of insulin-GQD/insulin receptor clusters (type I). c) Endocytosisof fluorescent membrane patches into a vesicle (type II). d) Exocytosis of a vesicle containinginsulin-GQD/insulin receptor complexes (type III). e) Transient approaching and retrieval ofinsulin-GQD/insulin receptor containing vesicle (type IV). Scale bars 0.2 µm. Reproducedwith permission.[38] Copyright 2013, American Chemical Society.at 680 nm useful for in vivo bioimaging. Inaddition to bioimaging, these authors foundthat these GQDs could produce 1O2 via amultistate sensitization process (Figure 11a)with a quantum yield of about 1.3, which isthe highest yield reported for photodynamictherapy (PDT) agents. Consequently, theyperformed in vivo fluorescence imaging andPDT in mice models (Figure 11b).Along with the in vivo fluorescenceimaging for animals in macroscopic scale,GQDs also show promise for in vivoimaging of microcosmic structures in animals. By using two-photon scanning microscope and GQDs as fluorescent probes, Qianet al.,[32] monitored the flow distributionand clearance of GQDs in ear blood vessels of mice (Figure 12). It was found thatGQDs with a large two-photon absorptioncross-section and long-wavelength emissionhold great potentials for in vivo imaging ofmicrocosmic structures, such as brain bloodvessels.5. Conclusions and PerspectivesFigure 7. a) The up-conversion fluorescence spectra of GQDs undernear-IR fs laser excitation. b) Fluorescence cellular imaging of GQDs inMC3T3-E1 cells under 808 nm excitation. The detection wavelength wasin the 490–550 nm range. Reproduced with permission.[47]of GQDs could be detected around the heart, liver, spleen, andkidneys at 8 h post-injection (Figure 10).[37b]Ge and co-workers synthesized GQDs using a hydrothermalmethod with polythiophene derivatives (PT2) as the carbonsource.[41] The GQDs thus produced exhibited a broad absorption in the UV–vis region, along with a strong emission peakFigure 8. a) Schematic of the setup used for TPFI of N-GQDs in tissuephantom with different thickness. b) Penetration depth of NGQDs forTPFI (right panel) and OPFI (left panel) in tissue phantom (all scalebars: 100 µm). Reproduced with permission.[48] Copyright 2013. AmericanChemical Society.Part. Part. Syst. Charact. 2015, 32, 515–523GQDs have attracted tremendous attention in many fields,including chemistry, physics, and biomedicine. It was notedthat in many recent publications various carbon-based nanostructures were named as “GQDs,” however, a closer look atthe data often reveals that those particles were actually CDs. Inthis article, we focus on the applications of real GQDs, whichpossess graphene lattices inside the dots smaller than 100 nmin size and less than 10 layers in thickness. We have presented a focused review on the design, synthesis, and applications of GQDs for in vitro fluorescence imaging in cells andtissues and in vivo macroscopic and microscopic imaging inanimals. Even this short review article has revealed the versatility of GQDs for bioimaging, biomedical diagnosis andtherapy. With so many synthetic approaches already reported,and more to be developed, there will be many opportunitiesfor developing various new GQDs with tailor-made structuresand well-defined fluorescence properties desirable for bioimaging. However, the applications of GQDs for bioimaging arestill at its early stage with many important issues remained tobe addressed:1. Although GQDs with emission wavelengths ranging fromUV to near infra-red have been synthesized via variousbottom-up and top-down approaches, the quantum yieldsof GQDs reported so far are much lower than conventionalsemiconductor QDs. There is an urgent need to improvethe quantum yield of GQDs. GQDs with bright red/nearinfra-red emissions are considered as ideal nanoprobes forbioimaging.2. Targeted imaging of tumors based on GQDs has rarelybeen demonstrated in vivo. The next generation of cancerdiagnostic agents for small animals requires improvement 2014 WILEY-VCH Verlag GmbH & Co. KGaA, Weinheimwileyonlinelibrary.com519
omsimultaneous imaging and therapy.4. By optimizing the multi-photon excitationand emission properties of GQDs, their novelapplications in biomedical areas, such as neurobehavioral analysis, gene therapy in thebrain, and barrier penetration through brainblood capillaries, could be exploited.Figure 9. In vivo imaging of KB tumor- bearing mice after intravenous injection of GQDs (5and 10 mg kg 1). Reproduced with permission.[26b] Copyright 2013, American Chemical Society.in their accumulation efficiency in tumor tissues. Edgefunctionalization of GQDs with antibodies or peptides fortargeted imaging of specific cancers needs to be furtherexplored.3. Development of multifunctional nanoprobes to covera broad spectrum of imaging modalities is always aninteresting topic. It is still a major challenge to designGQDs-based nanoprobes for simultaneous optical imaging, MRI, and CT monitoring. In these cases, the opticaland radioactive properties of GQDs will be promising for5. Like many other new biomedical technologies, there are concerns about the possibleside effects derived from the use of GQDs.Cytotoxicity and genetoxicity studies of GQDswith different components, sizes, shapes, andsurface coatings need to be investigated. Biodistribution studies of GQDs in various animal models are also needed.Continued research and developmentefforts in this emerging field is of great value. This will revolutionize the way in which future biomedical tests and clinicaldiagnoses to be performed that could affect many aspects of ourlives.AcknowledgementsThe authors thank our colleagues for their contributions to the workcited. The authors are also grateful for financial support from NSF(CMMI-1400274, CMMI-1266295, AIR-IIP-1343270, DMR 1106160),Figure 10. a) Side- and b) front-view images of mice showing that the fluorescence intensity decreases slowly after injection. c) Ex vivo images weretaken after dissecting the GNP treated mice, which shows biodistribution and accumulation into the different organs. Reproduced with permission.[37b]Copyright 2013, Royal Society of Chemistry.520wileyonlinelibrary.com 2014 WILEY-VCH Verlag GmbH & Co. KGaA, WeinheimPart. Part. Syst. Charact. 2015, 32, 515–523
EWFigure 11. a) Schematic illustration of the 1O2 generation mechanisms by conventional PDT agents (left) and GQDs (right). b) Photographs of miceafter various treatments on the 1st, 9th, 17th, and 25th d. (PDT: GQDs light irradiation; C1: GQDs only; C2: light irradiation only.) Reproduced withpermission.[41] Copyright 2014, Macmillan Publishers Ltd.Figure 12. In vivo two-photon scanning and one-photon confocal luminescence imaging of intravenously injected GQDs in a blood vessel of a mouseear at various time points after sample treatment. Scale bar: 50 µm. Reproduced with permission.[32]Part. Part. Syst. Charact. 2015, 32, 515–523 2014 WILEY-VCH Verlag GmbH & Co. KGaA, Weinheimwileyonlinelibrary.com521
omAFOSR (FA9550–12–1–0069, FA9550–12–1–0037), DAGSI (RQ20CWRU-13–4), DOD-Army (W911NF-11–1–0209), and “Partial supportfrom the open-funding program of State Key Laboratory of OrganicInorganic Composites.”Received: October 15, 2014Revised: November 1, 2014Published online: November 29, 2014[1] B. Valeur, Molecular Fluorescence: Principles and Applications,Wiley-VCH, Weinheim 2002.[2] a) R. Weissleder, M. J. Pittet, Nature 2008, 452, 580; b) R. Weissleder,V. Ntziachristos, Nat. Med. 2003, 9, 123.[3] W. A. Kalender, Phys. Med. Biol. 2006, 51, R29.[4] E. M. B. Haacke, M. L. Thompson, R. Venkatesan, Magnetic Resonance Imaging: Physical Principles and Sequence Design, Wiley,New York 1999.[5] T. A. Holly, B. G. Abbott, M. Al-Mallah, D. A. Calnon, M. C. Cohen,F. P. DiFilippo, E. P. Ficaro, M. R. Freeman, R. C. Hendel, D. Jain,S. M. Leonard, K. J. Nichols, D. M. Polk, P. Soman, J. Nucl. Cardiol.2010, 17, 941.[6] B. L. Bailey, D. W. Townsend, P. E. Valk, M. N. Maisey, PositronEmission Tomography: Basic Science and Clinical Practise, Springer,London 2003.[7] a) V. Ntziachristos, J. Ripoll, L. H. V. Wang, R. Weissleder, Nat. Biotechnol. 2005, 23, 313; b) P. N. Prasad, Introduction to Biophotonics,Wiley, New York 2003; c) J. Xie, G. Liu, H. S. Eden, H. Ai, X. Chen,Acc. Chem. Res. 2011, 44, 883.[8] H. Kobayashi, M. Ogawa, R. Alford, P. L. Choyke, Y. Urano, Chem.Rev. 2010, 110, 2620.[9] J. Zhou, Z. Liu, F. Y. Li, Chem. Soc. Rev. 2012, 41, 1323.[10] a) C. Ricard, J. C. Vial, J. Douady, B. van der Sanden, J. Biomed.Opt. 2007, 12, 064017; b) C. Stosiek, O. Garaschuk, K. Holthoff,A. Konnerth, Proc. Natl. Acad. Sci. USA 2003, 100, 7319.[11] R. Y. Tsien, Annu. Rev. Biochem. 1998, 67, 509.[12] a)M. Bruchez Jr., M. Moronne, P. Gin, S. Weiss, A. P. Alivisatos,Science 1998, 281, 2013; b) W. C. W. Chan, S. M. Nie, Science 1998,281, 2016.[13] a)J. Qian, D. Wang, F. H. Cai, Q. Q. Zhan, Y. L. Wang, S. L. He, Biomaterials 2012, 33, 4851; b) S. Palantavida, N. V. Guz, I. Sokolov,Part. Part. Syst. Charact. 2013, 30, 804.[14] a) K. Li, B. Liu, Chem. Soc. Rev. 2014, 43, 6570; b) D. Wang, J. Qian,W. Qin, A. Qin, B. Z. Tang, S. He, Sci. Rep. 2014, 4, 4279; c) Z. Zhao,B. Chen, J. Geng, Z. Chang, L. Aparicio-Ixta, H. Nie, C. C. Goh,L. G. Ng, A. Qin, G. Ramos-Ortiz, B. Liu, B. Z. Tang, Part. Part.Syst. Charact. 2014, 31, 481; d) D. Wang, J. Qian, S. He, J. S. Park,K. S. Lee, S. Han, Y. Mu, Biomaterials 2011, 32, 5880.[15] a) L. Tong, W. He, Y. S. Zhang, W. Zheng, J. X. Cheng, Langmuir 2009,25, 12454; b) D. J. Bharali, I. Klejbor, E. K. Stachowiak, P. Dutta,I. Roy, N. Kaur, E. J. Bergey, P. N. Prasad, M. K. Stachowiak, Proc.Natl. Acad. Sci. USA 2005, 102, 11539; c) E. Oh, F. K. Fatemi,M. Currie, J. B. Delehanty, T. Pons, A. Fragola, S. Lévêque-Fort,R. Goswami, K. Susumu, A. L. Huston, I. L. Medintz, Part. Part.Syst. Charact. 2013, 30, 453.[16] a) G. Y. Chen, H. L. Qiu, P. N. Prasad, X. Y. Chen, Chem. Rev. 2014,114, 5161; b) H. J. Wang, R. Shrestha, Y. Zhang, Part. Part. Syst.Charact. 2014, 31, 228.[17] a) K. Welsher, Z. Liu, D. Daranciang, H. Dai, Nano Lett. 2008, 8,586; b) X. Sun, Z. Liu, K. Welsher, J. T. Robinson, A. Goodwin,S. Zaric, H. Dai, Nano Res. 2008, 1, 203.[18] H. Qian, C. Dong, J. Peng, X. Qiu, Y. Xu, J. Ren, J. Phys. Chem. C2007, 111, 16852.[19] S. Kim, B. Fisher, H. J. Eisler, M. Bawendi, J. Am. Chem. Soc. 2003,125, 11466.522wileyonlinelibrary.com[20] T. Pons, N. Lequeux, B. Mahler, S. Sasnouski, A. Fragola,B. Dubertret, Chem. Mater. 2009, 21, 1418.[21] D. Wang, J. Qian, F. Cai, S. He, S. Han, Y. Mu, Nanotechnology2012, 23, 245701.[22] K. T. Yong, W.-C. Law, R. Hu, L. Ye, L. Liu, M. T. Swihart,P. N. Prasad, Chem. Soc. Rev. 2013, 42, 1236.[23] a) C. O. Girit, J. C. Meyer, R. Erni, M. D. Rossell, C. Kisielowski,L. Yang, C. H. Park, M. F. Crommie, M. L. Cohen, S. G. Louie,A. Zettl, Science 2009, 323, 1705; b) K. A. Ritter, J. W. Lyding,Nat. Mater. 2009, 8, 235.[24] Y. Xing, L. Dai, Nanomedicine 2009, 4, 207.[25] S. N. Baker, G. A. Baker, Angew. Chem. Int. Ed. 2010, 49, 6726.[26] a) H. Zhang, G. Grüner, Y. Zhao, J. Mater. Chem. B 2013, 1, 2542;b) M. Nurunnabi, Z. Khatun, K. M. Huh, S. Y. Park, D. Y. Lee,K. J. Cho, Y. Lee, ACS Nano 2013, 7, 6858.[27] Z. P. Zhang, J. Zhang, N. Chen, L. T. Qu, Energy Environ. Sci. 2012,5, 8869.[28] a) J. Shen, Y. Zhu, X. Yang, C. Li, Chem. Commun. 2012, 48, 3686;b) L. Li, G. Wu, G. Yang, J. Peng, J. Zhao, J.-J. Zhu, Nanoscale 2013,5, 4015; c) M. Bacon, S. J. Bradley, T. Nann, Part. Part. Syst. Charact.2014, 31, 415.[29] a) K. S. Novoselov, A. K. Geim, S. V. Morozov, D. Jiang, Y. Zhang,S. V. Dubonos, I. V. Gri
approach that can be used to visualize morphological details for . small organic molecules [ 10 ] and fl uorescent proteins [ 11 ] have been widely used as two DOI: 10.1002/ppsc.201400219 . State Key Laboratory of Organic-Inorganic Composites Beijing University of Chemical Technology Beijing 100029 , China .