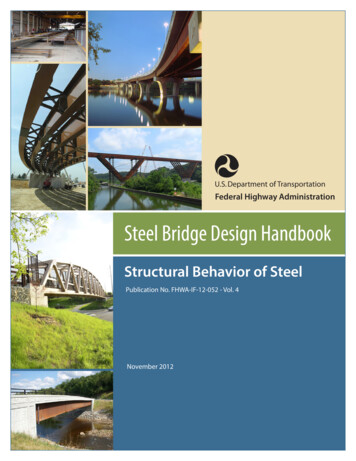
Transcription
U.S. Department of TransportationFederal Highway AdministrationSteel Bridge Design HandbookStructural Behavior of SteelPublication No. FHWA-IF-12-052 - Vol. 4November 2012
NoticeThis document is disseminated under the sponsorship of the U.S. Department of Transportation inthe interest of information exchange. The U.S. Government assumes no liability for use of theinformation contained in this document. This report does not constitute a standard, specification,or regulation.Quality Assurance StatementThe Federal Highway Administration provides high-quality information to serve Government,industry, and the public in a manner that promotes public understanding. Standards and policiesare used to ensure and maximize the quality, objectivity, utility, and integrity of its information.FHWA periodically reviews quality issues and adjusts its programs and processes to ensurecontinuous quality improvement.
Steel Bridge Design Handbook:Structural Behavior of SteelPublication No. FHWA-IF-12-052 - Vol. 4November 2012
Technical Report Documentation Page1. Report No.2. Government Accession No.FHWA-IF-12-052 – Vol. 44. Title and SubtitleSteel Bridge Design Handbook: Structural Behavior of Steel3. Recipient’s Catalog No.7. Author(s)Don White, Ph.D. (Georgia Institute of Technology)9. Performing Organization Name and AddressHDR Engineering, Inc.11 Stanwix StreetSuite 800Pittsburgh, PA 1522212. Sponsoring Agency Name and AddressOffice of Bridge TechnologyFederal Highway Administration1200 New Jersey Avenue, SEWashington, D.C. 205908. Performing Organization Report No.5. Report DateNovember 20126. Performing Organization Code10. Work Unit No.11. Contract or Grant No.13. Type of Report and Period CoveredTechnical ReportMarch 2011 – November 201214. Sponsoring Agency Code15. Supplementary NotesThis module was edited in 2012 by HDR Engineering, Inc., to be current with the AASHTO LRFD Bridge DesignSpecifications, 5th Edition with 2010 Interims.16. AbstractThe behavior of steel structures is an intricate and fascinating topic. This module is intended to serve as a guide to theAASHTO Load and Resistance Factor Design (LRFD) Specifications and their representation of the behavior of steelbridge systems and members. The module focuses on the structural form and function of bridge systems andmembers, with emphasis on strength limit states. Where relevant, recent advances in the AISC Specification forStructural Steel Buildings as well as findings from research developments are discussed in addition to the AASHTOLRFD Specfications. There are numerous areas where a broad understanding of the fundamental behavior ofstructures is key to the proper interpretation, application, and where necessary, extension of the AASHTO LRFDSpecfications. This module aims to aid the Engineer in reviewing and understanding the essential principles of steelsystem and member strength behavior and design.17. Key WordsSteel Bridge, Structural Behavior, Buckling, I-SectionMembers, Box-Section Members, Compression Members19. Security Classif. (of this report)UnclassifiedForm DOT F 1700.7 (8-72)18. Distribution StatementNo restrictions. This document is available to the public throughthe National Technical Information Service, Springfield, VA22161.20. Security Classif. (of this page)Unclassified21. No of Pages22. PriceReproduction of completed pages authorized
Steel Bridge Design Handbook:Structural Behavior of SteelTable of ContentsFOREWORD . 11.0 INTRODUCTION . 32.0BEHAVIOR AND STRUCTURE TYPES. 42.1 Rolled I-Section Stringer Systems . 42.2 General I-Section Stringer Systems . 52.2.1Overview . 52.2.2Fundamental Behavior of I-Section Stringer Systems . 92.2.3Integral Piers and Abutments . 162.2.4Temperature Movements . 182.3 Box-Section Stringer System . 212.4 Truss Bridges . 252.5 Arch Bridges . 282.6 Cable-Supported Bridges . 322.6.1 General . 323.02.6.2Suspension Bridges . 342.6.3Cable-Stayed Bridges. 36ELASTIC SYSTEM ANALYSIS, INELASTIC COMPONENT RESISTANCES. 414.0 OVERALL SYSTEM BUCKLING VERSUS INDIVIDUAL MEMBER BUCKLING . 464.1 Key Concepts . 464.2 Lean-On Bracing Systems . 494.3 General Consideration of System Stability Effects in Design . 515.0 MEMBER BEHAVIOR AND DESIGN STRENGTH . 535.1 Tension Members. 535.1.1Rolled or Built-Up Tension Members . 535.1.2Eyebars and Pin-Connected Plates. 58i
5.1.3 Strands. 615.2 Compression Members . 635.2.1Base Column Strength Equations . 635.2.2Flexural Buckling and Column Effective Length . 665.2.3Column Torsional and Torsional-Flexural Buckling . 685.2.3.1Torsional buckling of doubly-symmetric cross-sections . 685.2.3.2Flexural or torsional-flexural buckling of singly-symmetric cross-sections705.2.3.3Torsional-flexural buckling of general unsymmetric cross-sections . 715.2.3.4Special handling of double-angles and tees with non-slender elementsin AISC (2010) . 725.2.3.5Special handling of single angle compression members in AASHTO(2010) and AISC (2010) . 735.2.4Columns with Slender Elements . 775.2.4.1Width-to-thickness limits to prevent local buckling under uniformaxial compression. 775.2.4.2Compressive resistance of slender-element section members . 835.2.4.3Strength reduction Qs for members composed entirely of unstiffenedelements 845.2.4.4Strength reduction Q QaQs for members with stiffened elements . 845.2.4.5Axial capacity of hybrid slender-web girders . 895.2.4.6Local buckling criteria for solid-web arch ribs . 905.2.5Built-up Columns Composed of Two or More Shapes . 915.2.6Columns with Tapered and/or Stepped Sections and/or Nonuniform Internal AxialForce 955.2.7Composite Columns . 985.2.7.1AASHTO (2010) - AISC (1999) approach . 985.2.7.2AISC (2005) and (2010) approach . 1005.2.7.3Axial compression resistance of composite bridge girders. 1035.3 I-Section Flexural Members . 1065.3.1Introduction . 106ii
5.3.2 Proportioning Limits . 1075.3.3Compact Composite Sections in Positive Flexure . 1085.3.3.1Section classification . 1085.3.3.2Flexural resistance . 1095.3.3.3Handling of creep and shrinkage effects . 1115.3.4Noncompact Composite Sections in Positive Flexure . 1125.3.5Composite Sections in Negative Flexure and Noncomposite Sections . 1135.3.5.1Key concepts . 1135.3.5.2Maximum potential flexural resistance, Mmax or Fmax. 1175.3.5.2.1 Compact- and noncompact-web sections. 1175.3.5.2.2 Slender-web sections . 1185.3.5.2.3 Hybrid-web strength reduction factor . 1205.3.5.2.4 Other considerations . 1215.3.5.3Tension flange yielding (TFY) resistance. 1225.3.5.4Compact bracing limit, Lp . 1235.3.5.5Compact flange slenderness limit, pf . 1265.3.5.6Compression flange stress at the nominal onset of inelastic buckling,Fyr1265.3.5.7Elastic LTB stress, Fe.LTB . 1275.3.5.8Noncompact bracing limit, Lr . 1295.3.5.9Elastic FLB stress, Fe . 1305.3.5.10Noncompact flange slenderness limit, rf . 1305.3.5.11Moment gradient modifier, Cb . 1305.3.5.12Other considerations specific to composite I-section members innegative bending . 1395.3.5.13LTB effective lengths . 1415.3.5.14Inelastic redistribution of interior pier moments in continuous-spanbridges1445.3.6Stepped, Variable Web Depth and Other Nonprismatic I-Section Members . 1475.3.7Combined Major-Axis Bending, Minor-Axis Bending and Torsion . 1545.3.7.1General . 1545.3.7.2Calculation of flange lateral bending stresses . 157iii
5.3.7.35.3.85.3.9One-third rule concept . 158Shear Strength . 1605.3.8.1General . 1605.3.8.2Longitudinally-stiffened members . 1645.3.8.3Variable web depth members. 1645.3.8.4Web transverse stiffeners . 165Shear Connectors . 1685.3.10 Secondary Limit States . 1745.3.10.1Net section fracture . 1745.3.10.2Web bend buckling . 1755.3.10.3Longitudinal stiffeners . 1775.3.10.4Bearing stiffeners . 1825.3.10.5Web yielding and web crippling . 1825.4 Box-Section Flexural Members . 1835.4.1Introduction . 1835.4.2Categorization of Box-Girder Bridges in AASHTO (2010) . 1855.4.2.1Straight multiple-box-girder bridges satisfying the restrictions inArticle 6.11.2.3 and having fully effective flanges . 1855.4.2.2Box-girder bridges not satisfying one or more of the aboverequirements . 1875.4.35.4.4Other General Requirements Applicable to All Types of Box-Girder Bridges . 1895.4.3.1Diaphragm requirements at supports (Article 6.7.4.3) . 1895.4.3.2Bearing requirements (Article 6.11.1.2) . 1905.4.3.3Top lateral bracing requirements in tub girders (Article 6.7.5.3) . 190Additional Requirements for Specific Box-Girder Bridge Types . 1915.4.4.1Horizontally curved boxes (multiple or single) . 1925.4.4.2Single boxes . 1925.4.4.3Closed boxes . 1925.4.5Proportioning Limits . 1935.4.6Compact Composite Sections in Positive Flexure . 1935.4.7Noncompact Composite Sections in Positive Flexure . 194iv
5.4.8 Noncomposite Sections . 1965.4.9Composite Sections in Negative Flexure . 1975.4.10 Bottom Box Flange at Interior Pier Sections . 2015.4.11 Concrete Slab . 2025.4.12 Stepped, Variable Web Depth and Other Nonprismatic Box-Section Members 2025.4.13 Web Shear Strength . 2035.4.14 Shear Connectors . 2045.4.15 Comparison to the Wolchuk and Mayrbaurl (1980) Proposed Specifications forLong-Span Steel Box-Girder Bridges . 2055.4.15.1Box flange effective widths . 2055.4.15.2Resistance of unstiffened box flanges in compression . 2085.4.15.3Resistance of stiffened box flanges in compression . 2095.4.15.4Shear resistance . 2105.5 Miscellaneous Flexural Members . 2115.5.1Introduction . 2115.5.2I-Section Members in Weak-Axis Bending . 2115.5.3Noncomposite Box-Section Members . 2125.5.4Circular Tubes . 2155.5.5Tees and Double Angles in Strong-Axis Bending . 2165.5.6Channels in Strong- and Weak-Axis Bending . 2185.5.7Rectangular Bars and Rounds . 2195.5.8Single Angles . 2205.5.9Concrete-Encased and Filled Members . 2205.5.9.1AASHTO (2010) - AISC (1999) approach . 2205.5.9.2AISC (2010) Approach . 2235.6 Combined Flexure and Axial Load . 2235.6.1Introduction . 2235.6.2AASHTO (2010) and Primary AISC (2010) Beam-Column Interaction Equations2235.6.3Noncomposite Members . 225v
5.6.3.1In-plane resistance of doubly-symmetric I-section members subjectedto axial load and major- or minor-axis bending . 2255.6.3.1.1 Enhanced strength interaction curves for singly-symmetricmembers loaded in their plane of symmetry . 2345.6.3.1.2 Usage of the AASHTO (2010) and primary AISC (2010) bilinearinteraction equations with relaxed flange and/or web compactness limits . 2365.6.3.2Out-of-plane strength of doubly-symmetric rolled nonslender-elementI-section members with KLz KLy subjected to axial load and major-axis bending2375.6.3.35.6.45.6.5Other types of beam-columns, general loading conditions . 241Composite Members . 2425.6.4.1I- and box-section members with a composite concrete deck . 2425.6.4.2Concrete-encased sections and concrete-filled boxes and tubes. 243Summary Assessment of Beam-Column Strength Calculations . 2476.0 CONCLUDING REMARKS . 2487.0 REFERENCES . 249vi
List of FiguresFigure 1 Typical composite rolled I-beam or welded I-girder bridge cross-section. 5Figure 2 Effect of Shear lag. . 6Figure 3 Typical composite I-girder substringer system. . 7Figure 4 Two-girder system with floor beams and stringers. . 7Figure 5 Two-girder system with cross-girders. . 7Figure 6 Example complex plan geometry (courtesy of HDR Engineering, Inc.) . 9Figure 7 Plan view illustrating the required resultants for the reactions due to dead load at theends of a simply-supported horizontally-curved bridge with radial supports. 9Figure 8 Transfer of vertical shear forces due to torsion (V-loads) by the cross-frames and theslab in a curved bridge . 10Figure 9 Illustration of twisting and warping deformation of an I-section member. . 11Figure 10 Simplified calculation of flange lateral bending stresses on a curved I-girder subjectedto uniform bending moment. 12Figure 11 Use of lateral bracing within a few unbraced lengths adjacent to supports to reduce Igirder lateral bending stresses due to wind. . 13Figure 12 Plan view of deflections at the bottom and top of skewed diaphragms or cross-framesat a bearing line, forcing a coupling between major-axis bending and torsional rotation of thegirders. . 14Figure 13 Cross-frame, bridge cross-section, and girder torsional rotations due to differentialgirder displacements in skewed bridges (Coletti and Yadlosky 2005). . 15Figure 14 Use of staggered cross-frames to reduce the forces attracted to the cross-framemembers near the supports in skewed I-girder bridges. . 15Figure 15 Typical post-tensioned concrete integral bent cap with a single column pier(Wasserman 1997) (courtesy of Tennessee DOT and NSBA). . 17Figure 16 Straddle bents with integral steel pier caps (Abu-Hawash et al. 2005) (courtesy ofIowa DOT, HDR Engineering, and NSBA). 17Figure 17 Typical integral abutment detail (courtesy of Tennessee DOT). . 19Figure 18 Typical semi-integral abutment detail (courtesy of Tennessee DOT). 20Figure 19 Bearing orientation to accommodate thermal movement on a horizontally curvedalignment (NSBA 2004). . 20vii
Figure 20 Stresses in a single box girder subjected to an eccentric load. . 21Figure 21 Representative tub-girder bridge cross-section. . 23Figure 22 Shear center location and predominant torsional deformations for a tub girder sectionthat does not have a top-flange bracing system. . 23Figure 23 Costa-e-Silva Bridge in Rio de Janeiro, Brazil, second-longest box-girder span, 980 ft(courtesy of www.structurae.de) . 24Figure 24 Costa-e-Silva Bridge side elevation and cross-section (adapted from Ito et al. (1992)and Wolchuk (1997)) . 25Figure 25 Sfalassà Bridge in Calabria, Italy, longest box-girder span, 1230 ft (courtesy ofwww.structurae.de) . 25Figure 26 Ikitsuki Ohashi Bridge, Nagasaki, Japan, longest-span continuous truss bridge, 1300 ftmain span (www.sight-seeing.japan.com) . 27Figure 27 Quebec Bridge in Quebec, Canada, longest span cantilever truss bridge, 1800 ft mainspan (courtesy of www.structurae.de). . 27Figure 28 La Roize Bridge in France, triangular cross-section geometry using a single memberbottom chord and post-tensioning strand (courtesy of HNTB). . 28Figure 29 Slant-legged rigid-frame bridge (courtesy of HDR Engineering, Inc.) . 30Figure 30 LuPu Bridge in Shanghai, China, longest span steel arch, 1800 ft (courtesy ofwww.structurae.de). . 31Figure 31 LuPu Bridge deck system (courtesy of www.structurae.de). . 31Figure 32 New River Gorge Bridge in West Virginia, longest span steel arch in the UnitedStates, 1700 ft (courtesy of HDR Engineering, Inc.) . 31Figure 33 Cable suspended bridge systems, (a) suspension and (b) cable-stayed (reprinted withpermission from Podolny and Scalzi). . 32Figure 34 Akashi Kaikyo Bridge, longest suspension span (6530 ft) (courtesy ofwww.structurae.de). . 33Figure 35 Cooper River Bridge, longest cable-stayed span in the USA (1550 ft) (courtesy SouthCarolina DOT). . 34Figure 36 Sutong Bridge, longest cable-stayed span in the world (3570 ft) (courtesy of NewJersey DOT). . 35Figure 37 Representative two-span cable-stayed bridge system. . 37viii
Figure 38 Methods to avoid large tower bending moments in cable-stayed bridge systems withmore than three spans (Tang 2000). . 37Figure 39 Duisberg-Neuenkamp Bridge, Duisberg, Germany, 3-span cable-stayed bridgeutilizing a single plane of stay cables (1150 ft main span) (courtesy of www.structurae.de). . 38Figure 40 Alternative stay layouts, (a) harp pattern and (b) semi-harp pattern . 38Figure 41 Dual-girder subassembly composed of two equal-size doubly-symmetric I-girders . 47Figure 42 Example determination of whether system buckling or buckling of the girdersbetween the cross-frames governs for two equal-size doubly-symmetric I-girders. . 49Figure 43 A single cross-frame bridge cross-section showing multiple I-girders braced by asingle cross-frame . 50Figure 44 Plan view of a proof-of-concept skewed I-girder bridge utilizing lean-on bracing toalleviate large cross-frame forces and to reduce the number of required cross-frames (Herman etal. 2005) (reprinted with permission from Texas DOT). The x marks indicate the location ofcross-frames. . 51Figure 45 Dimensional requirements for eyebars specified to ensure good member performanceand development of the full yield capacity of an eyebar. . 59Figure 46 Requirements in addition to the checks of tension yielding, tension fracture and platebearing, for a specific pin connected plate with two equal size pin plates (w, a and e of pin plates w, a and e of main plate) bolted on each side of the main plate. . 61Figure 47 AASHTO (2010) and AISC (2010) column strength curve in terms of both KL/r and(Po/Pe)0.5 versus the SSRC multiple column curves 1P, 2P and 3P (Galambos 1998) and thetheoretical elastic buckling strength, steel columns with Fy 50 ksi . 65Figure 48 Torsional buckling of an I-section member. 69Figure 49 Single-angle cross-section and definition of geometric axes utilized by the AISC(2010) and AASHTO (2010) equivalent KL/r expressions. . 74Figure 50 Test end conditions associated with the recommended equivalent KL/r equations forsingle angle struts. . 74Figure 51 General form of AISC (2010)-AASHTO (2010) nominal strength curve for plate localbuckling. 78Figure 52 Theoretical kc values for elastic plate buckling (adapted from Salmon and Johnson(1996)). 82ix
Figure 53 Representative physical average (through thickness) stress distribution across thewidth of a postbuckled stif
Steel Bridge Design Handbook: Structural Behavior of Steel 5. Report Date November 2012 6. Performing Organization Code 7. Author(s) Don White, Ph.D. (Georgia Institute of Technology) 8. Performing Organization Report No. 9. Performing Organization Name and Address HDR Engineering, Inc. 11 Stanwix Street Suite 800 Pittsburgh, PA 15222 10. Work .Cited by: 1Publish Year: 2012