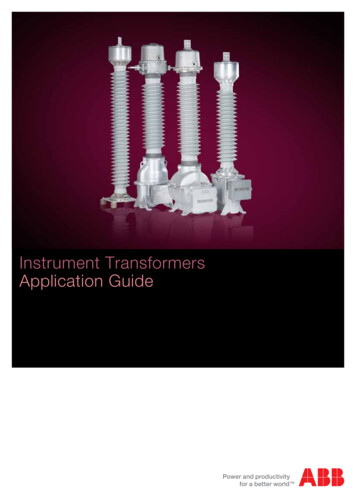
Transcription
Instrument TransformersApplication Guide
Installations withABB Outdoor Instrument TransformersLTB 420 E2 with current transformer IMB 420. Installation in Denmark.Substation in Oman with dessert climate. ABB equipment with IMB 145.Substation in Sweden with cold climate. ABB equipment with IMB 145.Inductive voltage transformers EMF 145. Installed in Sweden.Substation in Oman with dessert climate. ABB equipment with IMB 245.Current transformer IMB 420. Installation in Sweden.2 Application Guide ABB Instrument Transformers
ABB Instrument Transformers Application Guide 3
Table of contents1. General principles of measuring current and voltage81.1Instrument Transformers81.2Current transformers operating principles81.2.1Measuring errors1.2.2Calculation of errors9111.2.3Variation of errors with current131.2.4Saturation factor141.2.5Core dimensions151.3Voltage transformers operating principles161.3.1Measuring errors161.3.2Determination of errors181.3.3Calculation of the short-circuit impedance Z k201.3.4Variation of errors with voltage211.3.5Winding dimensions221.3.6Accuracy and burden capability231.4Capacitor Voltage Transformer (CVT)231.4.1Characteristics of a CVT241.4.2The CVT at no-load261.4.3The CVT at load271.4.4Calculation of internal resistance and load point for other burden than rated281.4.5Inclination of the load line301.4.6Frequency dependence of a CVT301.4.7Error changes for voltage variations311.4.8Different power factor of the burden311.4.9Error variation for temperature changes1.4.10 Quality factor31331.4.11 Leakage currents and stray capacitance341.4.12 Transient behavior341.4.13 Transient response341.4.14 Ferro-resonance352. How to specify current transformers362.1Rated insulation level362.1.1Altitude above sea level362.2Rated primary current382.3Rated continuous thermal current402.4Rated secondary current402.5402.6Short-time thermal current (Ith ) and dynamic current (Idyn)Burdens and accuracy classes422.6.1Measurement of current422.6.2Metering cores422.6.3Relay cores452.6.4Excitation curve462.6.5Accuracy classes according to IEC 61869-2482.6.6Accuracy classes according to IEEE C57.13502.7Pollution levels524 Application Guide ABB Instrument Transformers
3. Measurement of current during transient conditions533.1Background533.2The network533.3Important parameters553.3.1Rated primary current (I pn)553.3.2Rated secondary current (I sn)563.3.3Rated primary symmetrical short-circuit current (I psc)563.3.4Secondary burden (R b)563.3.5Specified duty cycle (C-O and C-O-C-O)563.3.6Secondary-loop time constant (Ts)573.3.7Rated symmetrical short-circuit current factor (K ssc)573.3.8Rated transient dimensioning factor (K td)583.3.9Flux overcurrent factor (n f)583.3.10 Maximum instantaneous error583.4Air gapped cores593.4.1Remanent flux (Ψ r)593.4.2Remanence factor (K r)593.5Accuracy classes for transient cores according to IEC 61869-2603.5.1Error limits for TPX, TPY and TPZ current transformers603.6How to specify current transformers for transient performance614. How to specify voltage transformers634.1Type of voltage transformer634.2Rated insulation level644.2.1Rated insulation levels according to IEC644.2.2Basic insulation levels according to IEEE/ANSI65654.3Rated primary and secondary voltage4.4Rated voltage factor (F V)664.5Burdens and accuracy classes664.6Pollution levels694.7Transient response for capacitor voltage transformers694.8Transient response for inductive voltage nce in capacitor voltage transformers71724.11Ferro-resonance in inductive voltage transformers4.12Fuses734.13Voltage drops in the secondary circuits734.14Coupling capacitors744.15CVTs as coupling capacitors754.16Measure harmonics with VTs765. Design of current transformers795.1General795.2Hair-pin type (Tank type)805.3Cascade/Eye-bolt type815.4Top core type815.5Combined current-voltage type82ABB Instrument Transformers Application Guide 5
Table of contents5.6Mechanical stress on current transformers835.6.1Forces on the primary terminals835.6.2Wind load835.6.3Seismic withstand846. Design of inductive voltage transformers856.1General856.2Mechanical stress on inductive voltage transformers866.2.1Forces on the primary terminals866.2.2Seismic withstand and wind load867. Design of capacitor voltage transformers877.1General877.2External disturbances on capacitor voltage transformers897.3Mechanical stress on capacitor voltage transformers907.4Seismic properties of ABB’s capacitor voltage transformers928. Instrument transformers in the system938.1Terminal designations for current transformers938.2Terminal designations for voltage transformers948.3Terminal designations for capacitor voltage transformers958.4Secondary grounding of current transformers968.5Secondary grounding of voltage transformers978.6Connection to obtain the residual voltage988.7Fusing of voltage transformer secondary circuits1008.8Location of current and voltage transformers in substations1008.8.1Location of current transformers1028.8.2Transformer and reactor bushing current transformers1038.8.3Physical order of cores in a current transformer1048.8.4Location of voltage transformers1049. Protective relays1059.1Current transformer classification1059.2Conditions1069.3Fault current1069.4Secondary wire resistance and additional load1079.5General current transformer requirements1079.6Rated equivalent secondary e.m.f. requirements1079.6.1Line distance protection REL 670 and REL 6501089.6.2Line differential protection RED6701099.6.2.1 Line differential function1099.6.2.2 Line distance function1109.6.3111Transformer protection RET670 and RET6509.6.3.1 Transformer differential function1119.6.3.2 Restricted earth fault protection (low impedance differential)1129.6.4Busbar protection REB6701159.6.4.1 Busbar differential function1159.6.4.2 Breaker failure protection1169.6.4.3 Non-directional instantaneous and definitive time, phase overcurrent protection1169.6.4.4 Non-directional inverse time delayed phase overcurrent protection1176 Application Guide ABB Instrument Transformers
9.6.5Bay control REC670 and REC6501189.6.5.1 Circuit breaker failure protection1189.6.5.2 Non-directional instantaneous and definitive time, phase and residual118overcurrent protection9.6.5.3 Non-directional inverse time delayed phase and residual overcurrent protection1199.6.5.4 Directional phase and residual overcurrent protection1209.6.6120Line distance protection REL670 (REL501, REL511, REL521, REL531)9.6.6.1 Line distance function1209.6.7121Line differential protection RED670 (REL551, REL561)9.6.7.1 Line differential function1219.6.7.2 Line distance function, additional for RED670 (REL561)1239.6.8Transformer protection RET670 (RET521) and transformer differential protection RADSB1249.6.9Busbar protection REB670 (RED521)1259.6.10High impedance differential protection RADHA1269.6.11Pilot-wire differential relay RADHL1269.7Current transformer requirements for CTs according to other standards1279.7.1Current transformers according to IEC 61869-2, class P, PR1279.7.2Current transformers according to IEC 61869-2 class PX, TPS1279.7.3Current transformers according to ANSI/IEEE12810. Non Coventional Instrument Transformers12910.1Fiber Optic Current Sensor (FOCS)12910.2DOIT — Technical description132ABB Instrument Transformers Application Guide 7
1. General principles of measuring current and voltage1.1 Instrument TransformersThe main tasks of instrument transformers are: To transform currents or voltages from a usually high value to a value easy to handlefor relays and instruments. To insulate the metering circuit from the primary high voltage system. To provide possibilities of standardizing the instruments and relays to a few ratedcurrents and voltages.Instrument transformers are special types of transformers intended to measure currents and voltages. The common laws for transformers are valid.Current transformersFor a short-circuited transformer the following is valid:1122This equation gives current transformation in proportion to the primary and secondary turns.A current transformer is ideally a short-circuited transformer where the secondary terminal voltage is zero and the magnetizing current is negligible.Voltage transformersFor a transformer in no load the following is valid:1122This equation gives voltage transformation in proportion to the primary and secondary turns.A voltage transformer is ideally a transformer under no-load conditions where the loadcurrent is zero and the voltage drop is only caused by the magnetizing current and isthus negligible.1.2 Current transformers operating principlesA current transformer is, in many respects, different from other transformers. Theprimary is connected in series with the network, which means that the primary andsecondary currents are stiff and completely unaffected by the secondary burden. Thecurrents are the prime quantities and the voltage drops are only of interest regardingexcitation current and measuring cores.8 Application Guide ABB Instrument Transformers
1.2.1 Measuring errorsI1I2BurdenFigure 1.1If the excitation current could be neglected the transformer should reproduce theprimary current without errors and the following equation should apply to the primary and secondary currents:In reality, however, it is not possible to neglect the excitation current.Figure 1.2 shows a simplified equivalent current transformer diagram converted to thesecondary side.(N1 / N2) x I1IeI2ExcitationImpedanceBurdenFigure 1.2The diagram shows that not all the primary current passes through the secondarycircuit. Part of it is consumed by the core, which means that the primary current is notreproduced exactly. The relation between the currents will in this case be:ABB Instrument Transformers Application Guide 9
1. General principles of measuring current and voltageThe error in the reproduction will appear both in amplitude and phase. The error inamplitude is called current or ratio error and the error in phase is called phase error orphase displacement.IeI2(N1 / N2) x I1δFigure 1.3εεδ(Ie / I2) x 100%I2 100%(I1 / I2) x (N1 / N2) x 100%Figure 1.4Figure 1.3 shows a vector representation of the three currents in the equivalent diagram. Figure 1.4 shows the area within the dashed lines on an enlarged scale.In Figure 1.4 the secondary current has been chosen as the reference vector and giventhe dimension of 100%. Moreover, a system of coordinates with the axles divided intopercent has been constructed with the origin of coordinates on the top of the referencevector. Since d is a very small angle, the current error e and the phase error d could bedirectly read in percent on the axis (d 1% 1 centiradian 34.4 minutes).10 Application Guide ABB Instrument Transformers
According to the definition, the current error is positive if the secondary current is toohigh, and the phase error is positive if the secondary current is leading the primary.Consequently, in Figure 1.4, the positive direction will be downwards on the e axis andto the right on the d axis.1.2.2 Calculation of errors(N1 / N2) x I1I2IfIμRiRbEsiXbFigure 1.5ε(If / I2) x 100%ϕ(Iμ / I2) x 100%δIsϕEsiFigure 1.6The equivalent diagram in Figure 1.5 comprises all quantities necessary for error calculations. The primary internal voltage drop does not affect the excitation current, andthe errors — and therefore the primary internal impedance — are not indicated in thediagram. The secondary internal impedance, however, must be taken into account, butonly the winding resistance Ri. The leakage reactance is negligible where continuousring cores and uniformly distributed secondary windings are concerned. The excitationimpedance is represented by an inductive reactance in parallel with a resistance.I m and If are the reactive and loss components of the excitation current.ABB Instrument Transformers Application Guide 11
1. General principles of measuring current and voltageThe error calculation is performed in the following four steps:1. The secondary induced voltage Esi can be calculated from2whereZThe total secondary impedance2. The inductive flux density necessary for inducing the voltage Esi can be calculated from2wherefFrequency in HzAjCore area in mm2N2Number of secondary turnsBMagnetic flux Tesla (T)3. The excitation current, Im and If, is necessary for producing the magnetic flux B.The magnetic data for the core material in question must be known. This information is obtained from an excitation curve showing the flux density in Gaussversus the magnetizing force H in ampere-turns/cm core length.Both the reactive component Hm and the loss component H f must be given.When Hm and H f are obtained from the curve, Im and If can be calculated from:2whereLjMagnetic path length in cmN2Number of secondary turns12 Application Guide ABB Instrument Transformers
4. The vector diagram in Figure 1.4 is used for determining the errors. The vectors Imand If, expressed as a percent of the secondary current I2, are constructed in thediagram shown in Figure 1.6. The directions of the two vectors are given by thephase angle between the induced voltage vector Esi and the reference vector I2.The reactive component Im is 90 degrees out of phase with Esi and the loss component If is in phase with Esi.1.2.3 Variation of errors with currentIf the errors are calculated at two different currents and with the same burden it willappear that the errors are different for the two currents. The reason for this is thenon-linear characteristic of the excitation curve. If a linear characteristic had beensupposed, the errors would have remained constant. This is illustrated in Figure 1.7and Figure 1.8. The dashed lines apply to the linear case.BBsBnHFigure 1.7Figure 1.8 on next page, shows that the error decreases when the current increases. This goes on until the current and the flux have reached a value (3) where thecore starts to saturate. A further inc
A current transformer is ideally a short-circuited transformer where the secondary ter-minal voltage is zero and the magnetizing current is negligible. Voltage transformers For a transformer in no load the following is valid: 1 2 1 2 This equation gives voltage transformation in proportion to the primary and secondary turns. A voltage transformer is ideally a transformer under no-load .