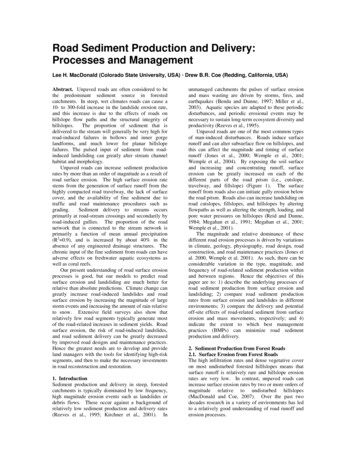
Transcription
Road Sediment Production and Delivery:Processes and ManagementLee H. MacDonald (Colorado State University, USA) Drew B.R. Coe (Redding, California, USA)Abstract. Unpaved roads are often considered to bethe predominant sediment source in forestedcatchments. In steep, wet climates roads can cause a10- to 300-fold increase in the landslide erosion rate,and this increase is due to the effects of roads onhillslope flow paths and the structural integrity ofhillslopes.The proportion of sediment that isdelivered to the stream will generally be very high forroad-induced failures in hollows and inner gorgelandforms, and much lower for planar hillslopefailures. The pulsed input of sediment from roadinduced landsliding can greatly alter stream channelhabitat and morphology.Unpaved roads can increase sediment productionrates by more than an order of magnitude as a result ofroad surface erosion. The high surface erosion ratestems from the generation of surface runoff from thehighly compacted road travelway, the lack of surfacecover, and the availability of fine sediment due totraffic and road maintenance procedures such asgrading.Sediment delivery to streams occursprimarily at road-stream crossings and secondarily byroad-induced gullies. The proportion of the roadnetwork that is connected to the stream network isprimarily a function of mean annual precipitation(R2 0.9), and is increased by about 40% in theabsence of any engineered drainage structures. Thechronic input of the fine sediment from roads can haveadverse effects on freshwater aquatic ecosystems aswell as coral reefs.Our present understanding of road surface erosionprocesses is good, but our models to predict roadsurface erosion and landsliding are much better forrelative than absolute predictions. Climate change cangreatly increase road-induced landslides and roadsurface erosion by increasing the magnitude of largestorm events and increasing the amount of rain relativeto snow. Extensive field surveys also show thatrelatively few road segments typically generate mostof the road-related increases in sediment yields. Roadsurface erosion, the risk of road-induced landslides,and road sediment delivery can be greatly decreasedby improved road designs and maintenance practices.Hence the greatest needs are to develop and provideland managers with the tools for identifying high-risksegments, and then to make the necessary investmentsin road reconstruction and restoration.1. IntroductionSediment production and delivery in steep, forestedcatchments is typically dominated by low frequency,high magnitude erosion events such as landslides ordebris flows. These occur against a background ofrelatively low sediment production and delivery rates(Reeves et al., 1995; Kirchner et al., 2001). Inunmanaged catchments the pulses of surface erosionand mass wasting are driven by storms, fires, andearthquakes (Benda and Dunne, 1997; Miller et al.,2003). Aquatic species are adapted to these periodicdisturbances, and periodic erosional events may benecessary to sustain long-term ecosystem diversity andproductivity (Reeves et al., 1995).Unpaved roads are one of the most common typesof man-induced disturbances. Roads induce surfacerunoff and can alter subsurface flow on hillslopes, andthis can affect the magnitude and timing of surfacerunoff (Jones et al., 2000; Wemple et al., 2001;Wemple et al., 2004). By exposing the soil surfaceand increasing and concentrating runoff, surfaceerosion can be greatly increased on each of thedifferent parts of the road prism (i.e., cutslope,travelway, and fillslope) (Figure 1). The surfacerunoff from roads also can initiate gully erosion belowthe road prism. Roads also can increase landsliding onroad cutslopes, fillslopes, and hillslopes by alteringflowpaths as well as altering the strength, loading, andpore water pressures on hillslopes (Reid and Dunne,1984; Megahan et al., 1991; Megahan et al., 2001;Wemple et al., 2001).The magnitude and relative dominance of thesedifferent road erosion processes is driven by variationsin climate, geology, physiography, road design, roadconstruction, and road maintenance practices (Jones etal. 2000, Wemple et al. 2001). As such, there can beconsiderable variation in the type, magnitude, andfrequency of road-related sediment production withinand between regions. Hence the objectives of thispaper are to: 1) describe the underlying processes ofroad sediment production from surface erosion andlandsliding; 2) compare road sediment productionrates from surface erosion and landslides in differentenvironments; 3) compare the delivery and potentialoff-site effects of road-related sediment from surfaceerosion and mass movements, respectively; and 4)indicate the extent to which best managementpractices (BMPs) can minimize road sedimentproduction and delivery.2. Sediment Production from Forest Roads2.1. Surface Erosion from Forest RoadsThe high infiltration rates and dense vegetative coveron most undisturbed forested hilllslopes means thatsurface runoff is relatively rare and hillslope erosionrates are very low. In contrast, unpaved roads canincrease surface erosion rates by two or more orders ofmagnitude relative to undisturbed hillslopes(MacDonald and Coe, 2007). Over the past twodecades research in a variety of environments has ledto a relatively good understanding of road runoff anderosion processes.
The first key point is that road travelways arehighly compacted and have very low infiltration rates(typically less than 5.0 mm hr-1) (Reid and Dunne,1984; Luce and Cundy, 1994; Loague and Kyriakidis,1997; Luce, 1997; Ziegler and Giambelluca, 1997).This results in the generation of infiltration-excess(Horton) overland flow even during small rainfallevents (Ziegler and Giambelluca, 1997). In addition,road cutslopes can intercept transient hillslopegroundwater (i.e., subsurface stormflow) when theheight of the cutslope exceeds the depth to the watertable (Ziegler et al., 2001b) (Figure 2).Theinterception of subsurface stormflow (SSF) isthreshold dominated, as SSF only occurs whenprecipitation exceeds 25-50 mm under wet antecedentconditions (Weiler et al., 2005). In some cases theinterception of SSF can account for more than 90% ofthe road surface runoff (LaMarche and Lettenmaier,2001; Wemple and Jones, 2003).The amount and energy of surface runoffdetermines the erosive force applied to the road prismby overland flow (Luce and Black, 1999). The roadprism can be broken into different process domains forsurface erosion based on the interaction of flowpathlength (L), which largely controls the amount ofrunoff, and slope (S), which is the primary control onthe energy of the runoff. On road cutslopes and roadfillslopes the slope can be very steep (Figure 1), butthe limited slope length limits the amount of flowaccumulation and hence the potential for hydraulicerosion.As a result, road cutslope and fillslopeerosion is primarily through rainsplash (if there is notmuch cover), sheetwash, and rill erosion if the slopelength allows sufficient runoff accumulation. Thelimited data suggests that cutslope erosion is usuallymuch less than the erosion from the road travelway(Ramos-Scharrón and MacDonald, 2007).Figure 1. A picture of a reconstructed outslopednative surface road on a highly erodible, weatheredgranodioritic hillslope in northern California, USA.The road prism is comprised of the cutslope,travelway, and fillslope, and the arrows show thepotential length of overland flow for each of thesepathways.Note how the rill networks on thetravelway concentrate the road surface runoff before itis discharged onto the fillslope. The extensive rillingis due to poor compaction during road reconstruction.Interceptionof gure 2.Schematic showing how subsurfacestormflow (SSF) along the soil-bedrock interface canbe intercepted by a road cutslope to create overlandflow (modified from Ziegler et al., 2001b).The slope of the travelway is usually limited toabout 10-12% in order to facilitate traffic andmaximize safety, but runoff can accumulate along thetravelway unless it is strongly outsloped or insloped(Figure 1). Detailed road surveys indicate that theaverage road segment length is about 50-70 m forforested areas in the western U.S. In many cases roadrunoff is prevented from running off the travelway bywheel ruts, and this can result in extensive rill or gullyerosion on the road surface. Inboard ditches alsocollect and concentrate runoff with a resulting risk ofditch incision and widening. Road fillslopes belowroad drainage outlets (i.e., relief culverts, rolling dips,and waterbars) are subject to the greatest erosiveforces because they are steep and the potentially largevolume of runoff draining to that point (Figure 2).The large volumes of water from longer road segmentsalso can induce gully erosion below drainage outlets(Montgomery, 1994; Wemple et al., 1996). Gullyerosion can be particularly severe when roads divertstream channels at road-stream crossings, and routethe streamflow down the road or onto hillslopes.The erodibility of the road prism varies as result oftime since construction, maintenance activites (i.e.,grading), soil texture, ground cover, and traffic (Luceand Black, 2001a; Ramos-Scharrón and MacDonald,2005; Ziegler et al., 2001a). Rainsplash erosion onroads is common due to the relative lack of vegetativecover, and can account for up to 38-48% of totalsediment production on freshly disturbed roadtravelways (Ziegler et al., 2000). Rainsplash erosionis highest on the road travelway, since this portion ofthe road prism is most frequently disturbed by trafficand typically has less vegetative cover than theadjacent cutslopes and fillslopes (Figure 1).Sediment production rates for cutslopes,travelways, and fillslopes are highest immediatelyafter road construction, with erosion rates decliningrapidly within 1-2 years (Megahan, 1974). Finetextured soils are the most susceptible to surfaceerosion, with siltier soils producing 4-9 times moresediment than soils dominated by sand or gravel (Luceand Black, 1999; Sugden and Woods, 2007). Soilswith higher rock content are more resistant to erosionand these soils typically have lower erosion rates(Sugden and Woods, 2007).
Table 1. Surface erosion rates for the travelway, cutslope, and fillslope for different study locations in megagrams (106grams) per hectare of road per year. Assuming an average road density of 4 km km-2 and an average road width of 6 m,these rates would apply to 2.4% of the catchment area. On this basis, multiplying these sediment production rates by0.024 allows a direct comparison with the sediment production rates from road-induced landslides in Table 2. Datacompiled by Carlos Ramos-Scharrón.SedimentStudyPortion ofproduction ratelocationroad prism(Mg ha-1 yr-1)North Carolina, USATravelway1143Lieberman & Hoover, 1948North Carolina, USATravelway7110Hoover, 1952Idaho Batholith, USATravelway73Megahan & Kidd, 1972Idaho Batholith, USATravelway20Megahan, 1975Washington, USATravelway4.8 – 66Southeast, USATravelway8 -120North Carolina, USATravelway37Northeast Oregon, USATravelway0–7Northwest Washington, USATravelway1 – 1010ReferenceWald, 1975Dissmeyer, 1976Simons et al., 1978Buckhouse & Gaither, 1982Reid & Dunne, 1984North Carolina, USATravelway0.3 - 52.4Swift, 1984Western Washington, USATravelway52Bilby, 1985Idaho Batholith, USATravelway23 - 76Vincent, 1985New ZealandTravelway0 – 113Fransen et al., 2001PolandTravelway98AustraliaTravelway50 – 90Grayson et al., 1993Oregon Coast Range, USATravelway1.8 – 37Luce and Black, 1999U.S. Virgin IslandsTravelway0.46 – 74MacDonald et al., 2001Froehlich, 1991U.S. Virgin IslandsTravelway74Sierra Nevada CA, USATravelway0.002 - 40Ramos-Scharrón & MacDonald, 2005North Coast CA, USATravelway0.5 – 46Barrett & Tomberlin, 2008Georgia, USACutslopes26 – 108Diseker & Richardson, 1962Coe, 2006Oregon, USACutslopes153 – 370Oregon, USACutslopes75 - 105Dyrness, 1970; 1975Wilson, 1963Idaho Batholith, USACutslopes150 - 165Megahan, 1980New GuineaCutslopes1050Blong & Humphreys, 1982New South Wales, AustraliaCutslopes36 - 58Riley, 1988South Island, New ZealandCutslopes52 - 152Fahey & Coker, 1989; 1992Idaho Batholith, USACutslopes0.1 - 248Megahan et al., 2001Idaho Batholith, USAFillslopes107Bethlahmy & Kidd, 1966Idaho Batholith, USAFillslopes12Megahan, 1978South Island, New ZealandFillslopes1 - 12.0Vegetative cover can protect the soil against surfaceerosion, and erosion from cutslopes and fillslopesdecline over time as they revegetate. Road travelwaysand inboard ditches are subjected to maintenanceactivities such as grading, and this removes the surfacecover and can greatly increase the supply of easily-Fahey & Coker, 1989; 1992erodible sediment. Recent studies have shown thatgrading can increase erosion rates from 70% to morethan an order of magnitude relative to ungraded roads(Luce and Black, 2001b; Ramos-Scharrón andMacDonald, 2005). Surface erosion rates declineexponentially to a baseline erosion rate following
initial construction or grading, and this rapid decline isdue to the rapid depletion of the readily erodiblematerial and the subsequent armoring of the roadprism (Megahan, 1974). (Megahan, 1974; Ziegleret al., 2001). Higher traffic levels increase the supplyof fine material, and this is a major reason why trafficcan increase sediment production rates by 2-1000times (Reid and Dunne, 1984; Ramos-Scharrón andMacDonald, 2005). Dry ravel from steep cutslopescan provide sediment to an inside ditch and the roadtravelway and thereby sustain higher surface erosionrates.The variations in rainfall, soil texture, traffic, andother controlling factors mean that road surfaceerosion rates vary over several orders of magnitude(Table 1). Both empirical and physically-based roadsurface erosion models have been developed, andthese typically include key variables such asprecipitation or rainfall erosivity, road slope, road areaor length, road surface slope, soil texture, time sincegrading, and traffic. Unfortunately it is still verydifficult to accurate predict road surface erosion forseveral reasons. First, many of these variables interact(e.g., traffic simulataneously affects infiltration rates,road surface cover, and the amount of erodiblematerial on the road surface). Second, the roadsurface characteristics and drainage patterns can beverydynamic as wheel ruts develop or waterbars breakdown. Third, most road erosion models only accountfor erosion due to infiltration-excess overland flow,even though the interception of SSF can be animportant source of road surface runoff (e.g., Wempleand Jones, 2003). Fourth, detailed road survey dataneed to be collected to predict surface erosion rates foreach road segment. Finally, the paucity of validationstudies for road surface erosion models means that themodels are most useful for predicting relative ratherthan absolute road surface erosion rates.2.2. Landslide Erosion from Forest RoadsForest roads increase landsliding by disrupting thebalance of driving and resisting forces acting upon andwithin hillslopes. As shown in Figure 3, road-relatedincreases in landsliding are commonly attributed to: 1)oversteepening and/or overloading of downslope areasby road fills; 2) removing support for unstablehillslopes by undercutting road cutslopes; and 3) andconcentrating road surface runoff onto potentiallyunstable portions of the road fillslope and lowerhillslopes (Benda et al., 1998; Sidle and Ochiai, 2006).Landsliding from roads can exceed naturallandsliding rates by one to two orders of magnitude(Table 2). Sediment production rates from roadinduced landslides are also an order of magnitudehigher than from clearcut hillslopes (Sidle and Ochiai,2006).Road-induced landsliding is generally only an issuein relatively steep terrain, with most road-initiatedfailures occurring on hillslopes greater than 31-39 (i.e., 60-80%) (Chatwin, 1994; Montgomery, 1994;Benda et al., 1998; Veldhuisen and Russell, 1999).Landslides initiated from fillslopes are typically larger2. Cutslope undercuttingsupport of upper hillslope3. Concentration ofrunoff ontounconsolidatedoversteepenedfill materialFillSlip surface1. Overloading thehead of slump withfill materialSlip surfaceFigure 3. Schematic showing how a road increasesthe likelihood of landsliding (modified from Benda etal., 1998).than those initiated from cutslopes (Wemple et a.,2001). Fill material is particularly unstable when it isplaced on slopes greater than 35 and on unstablelandforms such as colluvial hollows and inner gorges(Chatwin, 1994; Benda et al., 1998). Fillslope failuresare more likely on cut-and-fill roads and can belargely eliminated by the more costly approach of fullbench construction (Figure 4). This design excavatesa bench into the hillslope that is equal to the entirewidth of the travelway (Figure 4), but the trade-off isthat this generates a much higher cutslope.Cutslope failures are a common occurrence in steepareas as a result of the oversteepened hillslopes(Figure 3). By reducing the support at the toe ofunstable features (i.e., undercutting), cutslopes canincrease the likelihood of rotational sliding. Thepotential for oversteepening, undercutting unstablefeatures, and intercepting subsurface stormflow isgreatest on fully benched roads because of theincreased cutslope height (Figure 4). Cutslopes xcavatedhillslopeFullbenchedroadFigure 4. Schematic showing how different roaddesigns affect slope stability. (a) A cut-and-fill roadattempts to balance the amount of excavation with theamount of fill necessary to create the desired roadwidth. (b) A full benched road requires moreextensive excavation and a higher cutslope, but theexcavated material is removed rather than beingplaced on the hillslope.
Table 2. Sediment production rates from road-induced landslides in different forested areas (modified from Sidle andOchiai, 2006).SedimentIncrease overproduction ratenatural rate(Mg ha-1 yr-1)(times)Coastal SW British Columbia, Canada3.827O'Loughlin, 1972Western Oregon Cascades, USA3430Swanson and Dryness, 1975Study LocationReferenceWestern Oregon Cascades, USA202337Morrison, 1975Oregon Coast Range, USA2150Swanson et al., 1977South Island, New Zealand28Western Oregon Cascades, USAMosely, 198021.244Marion, 1981Oregon Klamath Mountains, USA3664Amaranthus et al., 1985North Coast California, USA64Weaver et al., 1995North Coast California, USA15Rice, 1999expose the hillslope to weathering, which canprogressively decrease the strength of the hillslopematerials. A downslope or fillslope failure also can beinitiated if a cutslope slide plugs the inside ditch andthe road runoff is then directed onto a fillslope orhillslope (Wemple et al., 2001).In many cases the increase in landsliding due toroads is a result of the hydrological changes ratherthan just the overloading, steepening, or undercuttingof hillslopes (Sidle and Ochiai, 2006). Roads increasethe amount of surface runoff and concentrate thisflow. When this water is routed onto fillslopes orhillslopes this can greatly decrease their stability as aresult of both the additional weight and the increase inpore water pressures. The decrease in permeabilitybetween the cutslope and the compacted road surfacealso can decrease the stability of the cutslope byincreasing pore water pressures at the base of thecutslope (Dutton et al., 2005).In the Pacific Northwest (USA), landslides canoccur on steep slopes (i.e., 31 ) when road lengths of60-130 m discharge overland flow below the outlets ofdrainage structures (Montgomery, 1994).Roadscrossing steep midslopes have a high likelihood ofintercepting subsurface stormflow, and cutslope andfillslope landslides are particularly common alongmidslope roads (Figure 5) (Wemple et al., 2001; Sidleand Ochiai, 2006). Midslopes are also commonlocations for unstable landforms such as colluvialhollows (Dietrich et al., 1993), and road drainagerouted into colluvial hollows increases their likelihoodof failure. Culverts at road-channel crossings can plugor overtop during storms, leading to catastrophicfailure of the road fill and the initiation of debris flows(Furniss et al., 1998).The prediction of road-related landsliding isdifficult given the stochastic nature of landslideinitiation, variability in road design and construction,and the inability to represent many of the causalprocesses for road-landslide interactions.Slopestability models such as SHALSTAB and SINMAPare useful for predicting the relative risk of failure andas landscape stratification tools. For managementpurposes these spatially-explicit estimates must befollowed by field-based slope stability assessments tobetter identify the risk for a specific area anddetermine the best way to minimize the risk of roadrelated landslides.Figure 5. A translational fillslope failure directlybelow a colluvial hollow.Colluvial hollowsconcentrate SSF, so placing fill material in theselandforms can increase the likelihood of landsliding.3. Sediment Delivery from Forest Roads3.1. Sediment Delivery from Road Surface ErosionThe delivery of road-related surface erosion is ofparticular concern because it is generally fine-grained(sand sized or smaller) (Ramos-Scharron andMacDonald, 2005), and this material is particularlydetrimental to many organisms (Waters 1995).Connectivity refers to the proportion of roads thatdrain directly to streams or other water bodies.Surveys indicate that the proportion of connectedroads is strongly controlled by road location, roaddesign, and the factors that control the amount of roadrunoff. In the western U.S. road-stream crossingsaccount for 30-75% of the connected road length(Wemple et al., 1996; Bowling and Lettenmaier, 2001;La Marche and Lettenmaier, 2001; Coe, 2006). Itfollows that road sediment delivery is highly
dependent on stream density, as this affects both thenumber of road-stream crossings and the proximity ofthe roads to the stream channel network.The delivery of road runoff and sediment tostreams generally decreases as the distance between aroad and a stream increases. The high infiltration ratesand high surface roughness of most forested hillslopesmeans that buffer strips can be quite effective attrapping road-related sediment. If the road runoff isdispersed, the sediment from road surface erosionrarely travels more than 30 m on vegetated hillslopes(Megahan and Ketcheson, 1996; Brake et al., 1999;Coe, 2006).However, if the road runoff isconcentrated into a single drainage outlet, the runoffand sediment can induce gullying and travel 3-4 timesfurther than when it is dispersed (Megahan andKetcheson, 1996; Coe, 2006).The development of gullies as a result ofconcentrated runoff is the second most importantmechanism for road-stream connectivity, as 9-35% ofthe total road length can be connected to the channelnetwork via this process (Wemple et al., 1996; Crokeand Mockler, 2001; Coe, 2006). Since longer roadsegments result in more runoff and more erosivepower below road drainage outlets, roads withinadequate drainage are much more likely to inducegullies and be connected to the stream channelnetwork that roads with dispersed or more frequentdrainage. Modeling studies have suggested that roadstream connectivity will increase with the amount ofinterceptedsubsurfaceflow(BowlingandLettenmaier, 2001; La Marche and Lettenmaier,2001), but there are not yet enough field studies toverify this relationship.A meta-analysis of the available data indicates thatroad-stream connectivity is a relatively simplefunction of annual precipitation and the presence ofengineered drainage structures (Coe, 2006). Theempirical predictive equation developed from 11studies in different parts of the world is:C 12.9 0.016P 39.5Mincrease in fine sediment deposition from roads will:decrease taxa richness and abundance; decrease theabundance and richness of sensitive taxa such asEphemeroptera, Plecoptera, and Tricoptera; andincrease the number of oligochaetes and burrowingchironomids (Waters, 1995). These macroinvertebratechanges will adversely affect the amount and type ofprey available to high-value fisheries. Large increasesin fine sediment and substrate embeddedness canadversely affect spawning and rearing habitat,decrease juvenile fish growth, and feeding efficiency(Everest et al., 1987; Suttle et al., 2004).3.2. Sediment Delivery from Road-RelatedLandslidesThe downstream delivery of road-induced landslides isdependent on their location relative to the channelnetwork, road design, and the travel distance of thefailure (MacDonald and Coe, 2007). Road-failuresinitiated in colluvial hollows have a higher likelihoodof delivering sediment to the channel network becausethese areas are located directly above first-orderchannels (Figure 6). Similarly, road-related failures ininner gorge landforms have a high probability ofdelivering sediment to streams because these areas aretypically very steep and the slopes feed directly intothe stream channels that carved these features(MacDonald and Coe, 2007). Landslides from roadscrossing steep midslopes also are likely to deliversediment to the channel network because hillslopes aresteep, roads frequently cross low-order channels, andthere is a high potential for intercepting subsurface(Wemple et al., 2001). Sediment delivery is also highwhen flood flows overtop road-channel crossings andinitiate landslides on the fillslopes at a crossing(Furniss et al., 1998) (Figure 7).(1)where C is the percent of road length or road segmentsthat are connected to the channel network, P is themean annual precipitation in millimeters, and M is abinary variable with 0 representing roads withdrainage structures, and 1 representing roads withoutdrainage structures (R2 0.92; p 0.0001).Thispredictive equation indicates the importance ofprecipitation in controlling both the amount of runoffand the density of the stream network. The binaryvariable indicates that well-designed roads withregular drainage will decrease road connectedness andhence road sediment delivery by at least 40%.The connectivity between roads and streams isimportant because any increase in fine sediment loadswill adversely affect water quality, macroinvertebratepopulations, fish habitat, salmonid populations, andthe health of coral reefs (Everest et al., 1987; Waters,1995; Suttle et al., 2004; Ramos-Scharron andMacDonald, 2007).For macroinvertebrates, anFigure 6. Road-induced debris flows in northwestWashington state, USA. The debris flows initiated inthe colluvial hollows on the upper road triggered thecatastrophic failures of the road-stream crossings onthe lower road. This sequence has been defined as a“disturbance cascade” (Wemple et al., 2001). Theroad was built prior to the implementation of bestmanagement practices and large fill volumes wereplaced within colluvial hollow and inner gorgelandforms (WA DNR, 1983).
Plugged culvertAbandonedchannelLandslidingNew channelFigure 7. Schematic showing how a plugged culvertor other crossing failure can cause severe erosion bydiverting water onto a road. When this water leavesthe road it can cause gullying and/or landslides.Culvert failures due to overtopping or plugging withsediment and woody debris are common when theculvert diameter is less than the active channel width,the culvert is not set to the stream grade, or the culvertis poorly aligned with the stream channel (taken fromKeller and Sherar, 2003).The delivery of sediment from road-relatedlandslides also depends on the road design. Sedimentfrom cutslope landslides is more likely to be deliveredto the stream network if the sediment is deposited intoan inside ditch it than on the road travelway (Wempleet al., 2001). Fillslope slides have a much higherlikelihood of delivering sediment to the channelnetwork, and in the western U.S. 50% of the fillslopeslides delivered sediment to the channel network aftera large flood event (30-100 year recurrence interval).Fillslope slides are also more likely to initiate debrisflows than cutslope slides (Wemple et al. 2001), anddebris flows almost always deliver sediment into thechannel network (MacDonald and Coe, 2007).Road-induced landslides deliver both fine andcoarse sediment (i.e., 2 mm) to the channel network.The episodic delivery of this sediment can inducedebris flows, debris fans, valley terrace formation,channel avulsion, increased bedload transport, channelaggradation, substrate fining, channel widening, andpool infilling (MacDonald and Coe, 2007). Thesesediment-induced changes in channel morphology canincrease downstream flooding and bank erosion byreducing the channel capacity, and also can adverselyaffect water quality and fish habitat (MacDonald andCoe, 2007).In summary, roads not only induce landslides at avery high rate relative to forests or clearcuts, but theyalso have a greater potential to deliver this sediment tothe stream network. In the Oregon Coast Range in thewestern USA, road-induced mass failures traveled onaverage three times farther than the mass failures in amature forest. The combination of a much highermass-failure rate and a higher sediment deliverymeans that road-induced mass failures can increase theamount of sediment being delivered to the channelnetwork by nearly five times relative to mature forests(May, 2002).4. Management ImplicationsThe effective mitigation of road-related sedimentproduction and delivery is dependent upon thedominant road erosion process and the properselection and implementation of best managementpractices (BMPs). Without sufficient knowledge ofthe relevant road erosion processes, managers aremore likely to treat the symptoms rather than theunderlying cause.Road surface sediment production can be reducedby improving road drainage, as this will decrease theamount of accumulated runoff and the erosive forceapplied to the road prism. Road drainage can beimproved by increasing the frequency of road
these rates would apply to 2.4% of the catchment area. On this basis, multiplying these sediment production rates by 0.024 allows a direct comparison with the sediment production rates from road-induced landslides in Table 2. Data compiled by Carlos Ramos-Scharrón. Sediment Study Portion of production rate