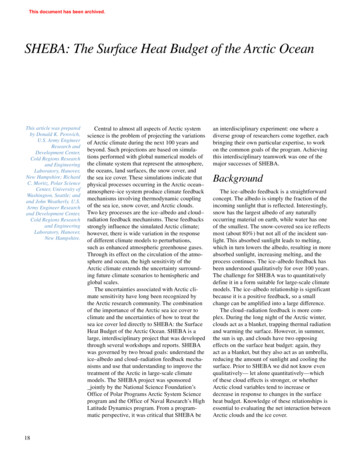
Transcription
This document has been archived.SHEBA: The Surface Heat Budget of the Arctic OceanThis article was preparedby Donald K. Perovich,U.S. Army EngineerResearch andDevelopment Center,Cold Regions Researchand EngineeringLaboratory, Hanover,New Hampshire; RichardC. Moritz, Polar ScienceCenter, University ofWashington, Seattle; andand John Weatherly, U.S.Army Engineer Researchand Development Center,Cold Regions Researchand EngineeringLaboratory, Hanover,New Hampshire.18Central to almost all aspects of Arctic systemscience is the problem of projecting the variationsof Arctic climate during the next 100 years andbeyond. Such projections are based on simulations performed with global numerical models ofthe climate system that represent the atmosphere,the oceans, land surfaces, the snow cover, andthe sea ice cover. These simulations indicate thatphysical processes occurring in the Arctic ocean–atmosphere–ice system produce climate feedbackmechanisms involving thermodynamic couplingof the sea ice, snow cover, and Arctic clouds.Two key processes are the ice–albedo and cloud–radiation feedback mechanisms. These feedbacksstrongly influence the simulated Arctic climate;however, there is wide variation in the responseof different climate models to perturbations,such as enhanced atmospheric greenhouse gases.Through its effect on the circulation of the atmosphere and ocean, the high sensitivity of theArctic climate extends the uncertainty surrounding future climate scenarios to hemispheric andglobal scales.The uncertainties associated with Arctic climate sensitivity have long been recognized bythe Arctic research community. The combinationof the importance of the Arctic sea ice cover toclimate and the uncertainties of how to treat thesea ice cover led directly to SHEBA: the SurfaceHeat Budget of the Arctic Ocean. SHEBA is alarge, interdisciplinary project that was developedthrough several workshops and reports. SHEBAwas governed by two broad goals: understand theice–albedo and cloud–radiation feedback mechanisms and use that understanding to improve thetreatment of the Arctic in large-scale climatemodels. The SHEBA project was sponsoredjointly by the National Science Foundation’sOffice of Polar Programs Arctic System Scienceprogram and the Office of Naval Research’s HighLatitude Dynamics program. From a programmatic perspective, it was critical that SHEBA bean interdisciplinary experiment: one where adiverse group of researchers come together, eachbringing their own particular expertise, to workon the common goals of the program. Achievingthis interdisciplinary teamwork was one of themajor successes of SHEBA.BackgroundThe ice–albedo feedback is a straightforwardconcept. The albedo is simply the fraction of theincoming sunlight that is reflected. Interestingly,snow has the largest albedo of any naturallyoccurring material on earth, while water has oneof the smallest. The snow-covered sea ice reflectsmost (about 80%) but not all of the incident sunlight. This absorbed sunlight leads to melting,which in turn lowers the albedo, resulting in moreabsorbed sunlight, increasing melting, and theprocess continues. The ice–albedo feedback hasbeen understood qualitatively for over 100 years.The challenge for SHEBA was to quantitativelydefine it in a form suitable for large-scale climatemodels. The ice–albedo relationship is significantbecause it is a positive feedback, so a smallchange can be amplified into a large difference.The cloud–radiation feedback is more complex. During the long night of the Arctic winter,clouds act as a blanket, trapping thermal radiationand warming the surface. However, in summer,the sun is up, and clouds have two opposingeffects on the surface heat budget: again, theyact as a blanket, but they also act as an umbrella,reducing the amount of sunlight and cooling thesurface. Prior to SHEBA we did not know evenqualitatively— let alone quantitatively—whichof these cloud effects is stronger, or whetherArctic cloud variables tend to increase ordecrease in response to changes in the surfaceheat budget. Knowledge of these relationships isessential to evaluating the net interaction betweenArctic clouds and the ice cover.
Ice Station SHEBA nearthe beginning of the drifton 28 October 1997. TheCanadian CoastGuard Icebreaker DesGroseilliers served as abase of operations forthe field experiment. Thehuts housed scientificequipment andlogistical supplies.The SHEBA program was divided into threephases. The first phase was directed towards analyzing existing data sets, formulating models, anddetermining the key knowledge gaps. DuringPhase 1 it became clear that the major obstacle tounderstanding the feedback mechanisms was alack of a comprehensive, integrated set of observations, and most importantly a set of observations that extended over an entire annual cycle.This identified need led directly to the centerpieceof Phase 2: the year-long drift of Ice StationSHEBA. Phase 3 is currently underway and isdirected towards analysis of the field results andmodel development.Ice Station SHEBAOn 2 October 1997, the Canadian Coast Guardicebreaker Des Groseilliers stopped in the middleof an ice floe in the Arctic Ocean, beginning theyear-long drift of Ice Station SHEBA. For thenext 12 months, until 11 October 1998, Ice Sta-tion SHEBA drifted with the pack ice from 75 N,142 W to 80 N, 162 W. At any given time, therewere 20–50 researchers at Ice Station SHEBA.During the year over 200 researchers participatedin the field campaign, spending anywhere fromjust a few days to the entire year.Conducting a year-long sea ice experimentprovided daunting scientific and logistic challenges: low temperatures, high winds, ice breakup, demanding instruments, and polar bears. Itwas truly a unique opportunity to observe withour eyes, as well as our instruments, the changesthat a sea ice cover undergoes over the course ofan annual cycle. For much of the year the ice wascovered by snow. The average snow depth wasabout 35 cm, and the surface was uniform andhad a large albedo. This was all changed by theonset of melt. The surface was transformed intoa highly variegated mixture of bare ice, meltponds, and open water, and the albedo decreasedsubstantially.Of course, the field program was much more19
than visual observations and personal impressions. There was an intense measurement program designed to obtain a complete, integratedtime series of every possible variable defining thestate of the “SHEBA column” over an entireannual cycle. This column is an imaginary cylinder stretching from the top of the atmospherethrough the ice into the upper ocean. Observations included longwave and shortwave radiativefluxes; the turbulent fluxes of latent and sensibleheat; cloud height, thickness, phase, and properties; energy exchange in the boundary layers ofthe atmosphere and ocean; snow depth and icethickness; and upper ocean salinity, temperature,and currents. This year-long, integrated data setprovides a test bed for exploring the feedbackmechanisms and for model development.ResultsViews from thebridge of the CCGC DesGroseilliers on 17 April1998 and 8 August 1998.20Cloud fraction and occurrence of liquid waterin the cloud were monitored using a combinationof radar and lidar. Clouds were pervasive atSHEBA. Even in midwinter the sky was overcastat least 40% of the time, and in the summer therewas almost continuous overcast. There was cloudliquid water present throughout the year, withliquid fractions of nearly 100% in summer andapproximately 20% in winter.The net surface radiation flux (that is, the netsurface longwave plus net surface shortwave irradiance) was negative during the winter. In winterthere was little to no incident solar radiation, andthe radiation flux was dominated by longwaveradiation. The large changes in the radiative fluxwere due to clouds. The net radiative flux waslarge and negative under clear-sky or high-cloudconditions. Under low clouds the net radiationwas much smaller in magnitude. By April theincreasing contribution of solar radiation resultedin the net radiative flux shifting from negative topositive. The net radiative flux reached a maximum of 130 W/m2 in mid-July, when incidentshortwave radiation was large, the surface albedowas relatively small, and there were low cloudspresent with warm air aloft. This confluence offactors resulted in both the net shortwave and thenet longwave fluxes being positive.The effect of changes in winter cloud conditions was also manifested in surface temperatures,with the low-cloud regime resulting in surfacetemperatures 10–20 C higher than the clear-skyor high-cloud regime. The annual average airtemperature at Ice Station SHEBA was only0.6 C lower than the regional climatological average temperature. There were, however, severaldifferences in the annual cycle of temperature.Comparing the climatological and SHEBA air
Selected annual cycle time series results from the SHEBA column: a) cloud fraction and occurrence of liquidwater in the cloud; b) daily averaged net radiation fluxes; c) Ice Station SHEBA and climatological air temperatures; d) snow depth (gray-shaded area), ice thickness (blue-red and red-white boundaries), and ice temperature(color contours); and e) elevation of ocean mixed layer temperature above the freezing point.21
temperature time series shows that the SHEBAyear was relatively cool in winter and warm inspring. Most pronounced was the difference inthe summer melt season. The SHEBA melt seasonwas quite long, lasting almost 80 days, comparedto an average of only 55 days. This long melt season had significant consequences for the ice massbalance.The mass balance was measured at more than100 sites. The snowpack accumulated slowly overnine months and then melted rapidly in only afew weeks in June. Rain on 29 May 1998 markedthe start of the surface melt season. The annualcycle of ice thickness was similar at all sites,though there was considerable spatial variabilityin the magnitude of the mass balance. The coldfront propagated down into the ice during fall,finally initiating bottom growth in November.There was a steady increase in ice thicknessthroughout the winter, with a gradual tapering inthe spring. In the summer the ice was isothermalat its melting point. On average at SHEBA therewere about 0.5 m of ice growth in winter and 0.64m of surface melt and 0.62 m of bottom melt insummer. There was a substantial net thinning ofthe ice at the SHEBA measurement sites of 0.75m during the SHEBA year.The upper oceanic mixed layer was close tothe freezing point for much of the year from fallthrough winter into late spring. With the onset ofsummer melt, the combination of a decrease in icealbedo and an increase in the area of open waterand ponded ice allowed significant amounts ofsunlight to be absorbed in the upper ocean. Thissolar energy resulted in warming that continuedthrough the summer, with the mixed layer reaching a peak temperature of 0.3 C in late July. Afterthis, a storm caused significant ice motion andmixing of the water. The storm-associated mixingresulted in the increased ice bottom melt and adecrease in water temperature.The analysis of the field data has providedmany insights into the ice–albedo and cloud–radiation feedbacks. The seasonal evolution ofareal surface albedo had five distinct phases, corresponding to the following surface conditions:dry snow, melting snow, pond formation, pondevolution, and fall freeze-up. To model the seasonal evolution of albedo accurately, it is necessary to accurately determine the timing of thesetransitions and to know the relative areas of ice,ponds, and open water. For the relatively low, wetcloud cover present at Ice Station SHEBA duringthe summer of 1998, the cloud–radiation feed-22back was positive. The net effect of the cloudswas warming and enhanced surface ablation.The SHEBA data set is fundamental to thelegacy of the SHEBA field experiment. Theanalysis of the Phase 2 field results has beencompleted, and the results are archived at http://www. joss.ucar.edu/cgi-bin/codiac/projs?SHEBA.These data are available for the use of all interested researchers.ModelingSome of the smallest-scale modeling isfocused on the interaction of radiation and seaice. A model of radiative transfer in sea ice hasbeen developed that uses the physical propertiesof ice measured at SHEBA and computes theradiation profile through an ice column. Radiationmeasurements through sea ice at SHEBA suggestthat the horizontal scattering of light in sea icecan play a significant role in the light transmittedaround and through melt ponds. The column radiation model can act as a tool to improve the treatment of radiation in large-scale models to accountfor melt ponds and impurities in ice such as sediment, brine, and bubbles.Modeling of the upper ocean has providedinsight and generated questions about some of theunique phenomena observed during SHEBA. Asteady warming of the upper ocean was measuredin June 1998, with temperatures elevated abovefreezing. The solar radiation is the dominant heatsource to the surface; it was not clear how thisenergy was reaching the upper ocean. The fraction of open water was below 5% in June, whichwould absorb a relatively small amount of energy.The diurnal cycle of the heating was synchronouswith the insolation, so a more remote heat sourcewas ruled out. A model of the upper ocean heatbalance suggests that 8% of the incoming solarradiation at the surface was absorbed in theocean, but it is not yet clear how this energywas transmitted through the ice cover.Parameterizing the atmospheric turbulentfluxes over sea ice has led to a new formulationof the drag coefficient appropriate for large-scalemodels. The high-frequency turbulence data fromSHEBA show that as the ice surface melts in summer, and ice concentration decreases, the surfacebecomes rougher and the drag coefficient increases,peaking at about 60% ice concentration. The vertical edge effects from melting floes and the disintegrating ice pack appear to dominate the drageffect in summer.
Modeling the vertical column of air, ice, andupper ocean following the SHEBA camp (theSHEBA column) has led to improvements in global climate models, such as the parameterizationof cloud liquid water content in the CommunityClimate System Model (CCSM). Using the lidarcloud measurements, the atmosphere profilesfrom balloonsonde data, and surface fluxes fromthe meteorology tower, the column model showeda bias of –20 W/m2 in the total longwave andshortwave radiation at the surface, resulting fromthe cloud-radiation scheme in the CCSM. Correcting the radiative path for the cloud liquidwater amounts found in SHEBA reduced this biassignificantly. The impact of this correction onglobal CCSM simulations has been a significantimprovement in the surface radiation in the Arctic, leading to more realistic ice and snow coverin the model.Sea ice models of both the thermodynamicsand dynamics observed during SHEBA haveshown the importance of processes that have yetto be incorporated into global climate models.The thermodynamic sea ice model used in theCCSM has been used to simulate the cycle ofgrowth and melt observed at SHEBA. Using themeteorological observations, including radiation,precipitation, and the upper ocean temperatures,the CCSM ice model simulates the growth andmelt of ice without melt ponds to within an average of 5 cm. The evolution of albedo that reflectsthe appearance and growth of melt ponds is notyet included in the CCSM ice model, so the modeled albedo shows a sharp transition to summermelt. The lateral melting and the reduction in iceconcentration during summer are highly dependent on the formulation of the ice–ocean heatflux and the model’s formulation of dynamic leadopening. The dynamic forcing on the ice in summer creates open water leads and allows greatersolar energy input to the upper ocean. This energy, in turn, creates greater lateral ice ablation andmore open water, which is the local-scale ice–albedo feedback. The ice models of the SHEBAcamp illustrate the interdependence of the dynamics and thermodynamics in the ice–albedo feedback and suggest that both must be representedappropriately in global climate models.ReferencesAndreas, E.L., C.W. Fairall, A.A. Grachev, P.S.Guest, T.W. Horst, R.E. Jordan, and P.O.G.Persson (2003) Turbulent transfer coefficientsand roughness lengths over sea ice: The SHEBA results. Seventh American MeteorologicalSociety Conference on Polar Meteorology andOceanography, Hyannis, MA.Intergovernment Panel on Climate Change (2001)Third Assessment Report, Climate Change 2001.Martin, S., and E.A. Munoz (1997) Properties ofthe Arctic 2-m air temperature for 1979–present derived from a new gridded data set.Journal of Climate, vol. 10, no. 6, p. 1428–1440.McPhee, M., G. A. Maykut, C.M. Bitz, and R.E.Moritz (2001) Early summer heating in theupper ocean in the vicinity of SHEBA. SixthAmerican Meteorological Society Conferenceon Polar Meteorology and Oceanography, SanDiego, CA.Moritz, R.E. (1990) Arctic System Science:Ocean–Atmosphere–Ice Interactions. JointOceanographic Institutions Incorporated,Washington, DC.Moritz, R.E., and D.K. Perovich (ed.) (1996) Surface Heat Budget of the Arctic Ocean, SciencePlan. ARCSS/OAII, Report No. 5, Universityof Washington, Seattle.Moritz, R.E., J.A. Curry, A.S. Thorndike, and N.Untersteiner (1993) SHEBA: A Research Program on the Surface Heat Budget of the ArcticOcean. ARCSS/OAII, Report No. 3.Perovich, D.K., et al. (1999) Year on ice gives climate insights. EOS, Transactions of the American Geophysical Union, vol. 80, p. 481, 485–486.Randall, D., et al. (1998) Status of and outlookfor large-scale modeling of atmosphere-iceocean interactions in the Arctic. Bulletin of theAmerican Meteorological Society, vol. 79, p.197–219.Rind, D., R. Healy, C. Parkinson, and D. Martinson (1995) The role of sea ice in 2 x CO2 climate model sensitivity. Part I: The total influence of sea-ice thickness and extent. Journalof Climate, vol. 8, p. 449–463.23
The Western Arctic Shelf–Basin Interactions ProjectThis article was preparedby Jackie Grebmeier,Director of the SBIProject Office and SBIProject Chief Scientist,Department of Ecologyand EvolutionaryBiology, The Universityof Tennessee, on behalfof all the SBI Phase IIparticipants, whoprovided many of theconcepts and results outlined in this article.Further information onthe overall SBI projectcan be found on the SBIweb site (http://sbi.utk.edu) or by contacting Jackie Grebmeier,Director of the SBIProject Office and SBIProject Chief Scientist,Marine Biogeochemistryand Ecology Group,Department of Ecologyand EvolutionaryBiology, The Universityof Tennessee, 10515Research Drive, Suite100, Bldg A, Knoxville,TN 37932;phone: 865-974-2592;fax: 865-974-7896;email: jgrebmei@utk.edu.24The National Science Foundation and theOffice of Naval Research are supporting an interdisciplinary global change research study knownas the Western Arctic Shelf–Basin Interactions(SBI) project. This project is part of the Ocean–Atmosphere–Ice Interactions component of NSF’sArctic System Science program. The goal of theSBI project is to improve our ability to assess theimpacts of global change on the physical and biogeochemical connections among the western Arctic shelves, slopes, and deep basins. The SBIproject focuses on shelf, shelf break, and upperslope water mass and ecosystem modifications,material fluxes, and biogeochemical cycles. Thegeographical focus is on the Chukchi and Beaufort Seas and adjacent upper slopes. An accumulated body of research indicates that climatechange will significantly impact the physical andbiological linkages between the Arctic shelvesand the adjacent ocean basins. SBI thereforefocuses on the outer shelf, shelf break, and upperslope, where it is believed that key processescontrol water mass exchange and biogeochemicalcycles and where the greatest responses to climatechange are expected to occur.The SBI project consists of three phases overa 10-year period. Phase I (1998–2001) involvedanalyses of historical data, opportunistic fieldinvestigations, and modeling of specific regionsand processes. SBI Phase II constitutes the fieldprogram taking place in the Bering Strait regionand over the outer shelf, shelf break, and slopeof the Chukchi and Beaufort Seas into the ArcticOcean. Phase III will focus on the development ofpan-Arctic models suitable for simulating scenarios of the impacts of climate change on shelf–basin interactions.The SBI field program has been developed tofocus on: Physical modifications of North Pacific andother waters on the Chukchi shelf and slope,and exchanges of these waters across theshelf and slope; Biogeochemical modifications of North Pacificand other waters over the Chukchi and Beaufort shelf and slope areas, with an emphasison carbon, nutrients, and key organisms thatrepresent the suite of trophic levels; and Comparative studies over the wide Chukchiand narrow Beaufort shelves and adjacentslopes to facilitate extrapolation and integration of the Western Arctic work to a panArctic perspective. Integrated process andmodeling studies of shelf–basin exchangeprocesses and their sensitivity to globalchange will be an important methodology inthis extrapolation. A physical–biological coupled model is being undertaken as part of theSBI study.Through integrated field and modeling efforts,the SBI project is investigating the effects of glo-
Arctic clouds and the ice cover. SHEBA: The Surface Heat Budget of the Arctic Ocean This article was prepared by Donald K. Perovich, U.S. Army Engineer Research and Development Center, Cold Regions Research and Engineering Laboratory, Hanover, New Hampshire; Richard C. Moritz, Polar Science Center, University of Washington, Seattle; and and .