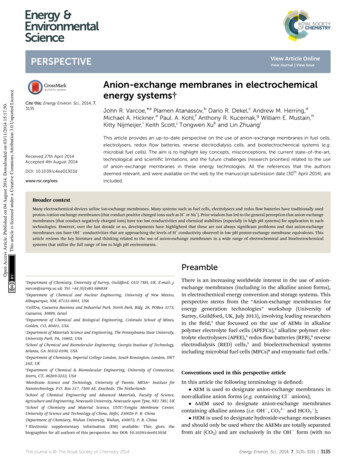
Transcription
Energy &EnvironmentalScienceOpen Access Article. Published on 04 August 2014. Downloaded on 03/11/2014 15:17:50.This article is licensed under a Creative Commons Attribution 3.0 Unported Licence.PERSPECTIVEView Article OnlineView Journal View IssueAnion-exchange membranes in electrochemicalenergy systems†Cite this: Energy Environ. Sci., 2014, 7,3135John R. Varcoe,*a Plamen Atanassov,b Dario R. Dekel,c Andrew M. Herring,dMichael A. Hickner,e Paul. A. Kohl,f Anthony R. Kucernak,g William E. Mustain,hKitty Nijmeijer,i Keith Scott,j Tongwen Xuk and Lin ZhuanglThis article provides an up-to-date perspective on the use of anion-exchange membranes in fuel cells,electrolysers, redox flow batteries, reverse electrodialysis cells, and bioelectrochemical systems (e.g.microbial fuel cells). The aim is to highlight key concepts, misconceptions, the current state-of-the-art,Received 27th April 2014Accepted 4th August 2014technological and scientific limitations, and the future challenges (research priorities) related to the useof anion-exchange membranes in these energy technologies. All the references that the authorsDOI: 10.1039/c4ee01303ddeemed relevant, and were available on the web by the manuscript submission date (30th April 2014), arewww.rsc.org/eesincluded.Broader contextMany electrochemical devices utilise ion-exchange membranes. Many systems such as fuel cells, electrolysers and redox ow batteries have traditionally usedproton-/cation-exchange membranes (that conduct positive charged ions such as H or Na ). Prior wisdom has led to the general perception that anion-exchangemembranes (that conduct negatively charged ions) have too low conductivities and chemical stabilities (especially in high pH systems) for application in suchtechnologies. However, over the last decade or so, developments have highlighted that these are not always signi cant problems and that anion-exchangemembranes can have OH conductivities that are approaching the levels of H conductivity observed in low pH proton-exchange membrane equivalents. Thisarticle reviews the key literature and thinking related to the use of anion-exchange membranes in a wide range of electrochemical and bioelectrochemicalsystems that utilise the full range of low to high pH environments.PreambleaDepartment of Chemistry, University of Surrey, Guildford, GU2 7XH, UK. E-mail: j.varcoe@surrey.ac.uk; Tel: 44 (0)1483 686838bDepartment of Chemical and Nuclear Engineering, University of New Mexico,Albuquerque, NM, 87131-0001, USAcCellEra, Caesarea Business and Industrial Park, North Park, Bldg. 28, POBox 3173,Caesarea, 30889, IsraeldDepartment of Chemical and Biological Engineering, Colorado School of Mines,Golden, CO, 80401, USAeDepartment of Materials Science and Engineering, The Pennsylvania State University,University Park, PA, 16802, USAfSchool of Chemical and Biomolecular Engineering, Georgia Institute of Technology,Atlanta, GA 30332-0100, USAThere is an increasing worldwide interest in the use of anionexchange membranes (including in the alkaline anion forms),in electrochemical energy conversion and storage systems. Thisperspective stems from the “Anion-exchange membranes forenergy generation technologies” workshop (University ofSurrey, Guildford, UK, July 2013), involving leading researchersin the eld,1 that focussed on the use of AEMs in alkalinepolymer electrolyte fuel cells (APEFCs),2 alkaline polymer electrolyte electrolysers (APEE),3 redox ow batteries (RFB),4 reverseelectrodialysis (RED) cells,5 and bioelectrochemical systemsincluding microbial fuel cells (MFCs)6 and enzymatic fuel cells.7gDepartment of Chemistry, Imperial College London, South Kensington, London, SW72AZ, UKhDepartment of Chemical & Biomolecular Engineering, University of Connecticut,Storrs, CT, 06269-3222, USAiMembrane Science and Technology, University of Twente, MESA Institute forNanotechnology, P.O. Box 217, 7500 AE, Enschede, The NetherlandsjSchool of Chemical Engineering and Advanced Materials, Faculty of Science,Agriculture and Engineering, Newcastle University, Newcastle upon Tyne, NE1 7RU, UKkSchool of Chemistry and Material Science, USTC-Yongjia Membrane Center,University of Science and Technology of China, Hefei, 230026 P. R. ChinalDepartment of Chemistry, Wuhan University, Wuhan, 430072, P. R. China† Electronic supplementary information (ESI) available: This gives thebiographies for all authors of this perspective. See DOI: 10.1039/c4ee01303dThis journal is The Royal Society of Chemistry 2014Conventions used in this perspective articleIn this article the following terminology is de ned: AEM is used to designate anion-exchange membranes innon-alkaline anion forms (e.g. containing Cl anions); AAEM used to designate anion-exchange membranescontaining alkaline anions (i.e. OH , CO32 and HCO3 ); HEM is used to designate hydroxide-exchange membranesand should only be used where the AAEMs are totally separatedfrom air (CO2) and are exclusively in the OH form (with noEnergy Environ. Sci., 2014, 7, 3135–3191 3135
View Article OnlineOpen Access Article. Published on 04 August 2014. Downloaded on 03/11/2014 15:17:50.This article is licensed under a Creative Commons Attribution 3.0 Unported Licence.Energy & Environmental Sciencetraces of other alkaline anions such as CO32 ); this is not thecase in most of the technologies discussed in the article (apossible exception being APEEs); AEI is used to designate an anion-exchange ionomer whichare anion-exchange polymer electrolytes in either solution ordispersion form: i.e. anion-exchange analogues to the protonexchange ionomers (e.g. Na on D-52x series) used in protonexchange membrane fuel cells (PEMFCs). AEIs are used aspolymer binders to introduce anion conductivity in the electrodes (catalyst layers). CEM is used to designate cation-exchange membranes innon-acidic form (e.g. containing Na cations); PEM is used to designate proton-exchange membranes (i.e.CEMs speci cally in the acidic H cation form);Perspective IEM is used to designate a generic ion-exchange membrane(can be either CEM or AEM).Note that in this review, all electrode potentials (E) are givenas reduction potentials even if a reaction is written as anoxidation.AEMs and AEIs for electrochemicalsystemsSummary of AEM chemistries used in such systemsAEMs and AEIs are polymer electrolytes that conduct anions,such as OH and Cl , as they contain positively charged[cationic] groups (typically) bound covalently to a polymerbackbone. These cationic functional groups can be boundProfessor John Varcoe (Department of Chemistry, University ofSurrey, UK) obtained both his1st class BSc Chemistry degree(1995) and his Materials Chemistry PhD (1999) at the University of Exeter (UK). He was apostdoctoral researcher at theUniversity of Surrey (1999–2006) before appointment asLecturer (2006), Reader (2011)and Professor (2013). He isrecipient of an UK EPSRC Leadership Fellowship (2010). His research interests are focused onpolymer electrolytes for clean energy and water systems: morespeci cally, the development of chemically stable, conductiveanion-exchange polymer electrolytes. He is also involved in theUniversity's efforts on biological fuel cells.ProfessorMichaelHickner(Associate Professor, Department of Materials Science andEngineering, Pennsylvania StateUniversity, USA) focuses hisresearch on the relationshipsbetween chemical compositionand materials performance infunctional polymers to addressneeds in new energy and waterpuri cation applications. Hisresearch group has ongoingprojects in polymer synthesis,fuel cells, batteries, water treatment membranes, and organicelectronic materials. His work has been recognized by a Presidential Early Career Award for Scientists and Engineers fromPresident Obama (2009). He has co-authored seven US andinternational patents and over 100 peer-reviewed publicationswith 5400 citations.Dr Dario Dekel (Co-Founder andVP for R&D and Engineering,CellEra, Israel) received hisMBA, MSc and PhD in ChemicalEngineering from the Technion,Israel Institute of Technology.He was the chief scientist andtop manager at Rafael AdvancedDefense Systems, Israel, wherehe led the world's second largestThermal Battery Plant. He le Rafael in 2007 to co-found CellEra, leading today a selectedgroup of 14 scientists and engineers, developing the novel AlkalineMembrane Fuel Cell technology. He currently holds 3M government research grants from Israel, USA and Europe. Dr Dekel holds14 battery and fuel cell patents.Professor Paul Kohl (HerculesInc./Thomas L. Gossage Chair,Regents' Professor, GeorgiaInstitute of Technology, USA)received a Chemistry PhD(University of Texas, 1978). Hewas then involved in new chemical processes for silicon andcompoundsemiconductordevices at AT&T Bell Laboratories (1978–89). In 1989, hejoined Georgia Tech.'s School ofChemical and BiomolecularEngineering. His research includes ionic conducting polymers, highenergy density batteries, and new materials and processes foradvanced interconnects for integrated circuits. He has 250 papers,is past Editor of JES and ESSL, past Director MARCO InterconnectFocus Center, and President of the Electrochemical Society(2014–15).3136 Energy Environ. Sci., 2014, 7, 3135–3191This journal is The Royal Society of Chemistry 2014
View Article OnlineOpen Access Article. Published on 04 August 2014. Downloaded on 03/11/2014 15:17:50.This article is licensed under a Creative Commons Attribution 3.0 Unported Licence.Perspectiveeither via extended side chains (alkyl or aromatic types ofvarying lengths) or directly onto the backbone (o en via CH2bridges); they can even be an integral part of the backbone.The most common, technologically relevant backbones are:poly(arylene ethers) of various chemistries8 such as polysulfones [including cardo, phthalazinone, uorenyl, andorganic–inorganic hybrid types],9 poly(ether ketones),10,11poly(ether imides),12 poly(ether oxadiazoles),13 and poly(phenylene oxides) [PPO];14 polyphenylenes,15 per uorinatedtypes,16,17 polybenzimidazole (PBI) types including where thecationic groups are an intrinsic part of the polymer backbones,18 poly(epichlorohydrins) [PECH],19 unsaturated polypropylene20 and polyethylene21 types [including those formedusing ring opening metathesis polymerisation (ROMP)],22 thosebased on polystyrene and poly(vinylbenzyl chloride),23 polyphosphazenes,24 radiation-gra ed types,25 those synthesisedusing plasma techniques,26 pore- lled types,27 electrospun bretypes,28 PTFE-reinforced types,26g,29 and those based on poly(vinyl alcohol) [PVA].19a,30The cationic head-group chemistries that have been studied(Scheme 1), most of which involve N-based groups, include:(a) Quaternary ammoniums (QA) such as benzyltrialkylammoniums [benzyltrimethylammonium will be treated asthe benchmark chemistry throughout this report],2,31 alkyl-bound(benzene-ring-free) QAs,21a,b and QAs based on bicyclic ammonium systems synthesised using 1,4-diazabicyclo[2.2.2]octane(DABCO) and 1-azabicyclo[2.2.2]octane (quinuclidine, ABCO)(to yield 2 and 1azoniabicyclo[2.2.2]octane {quinuclidinium}19c,33 functionalgroups, zolium,10a,13,23a,25g,31,34 benzimidazoliums,35 PBI systems where thepositive charges are on the backbone (with or without positivecharges on the side-chains),18b,d,f,h,36 and pyridinium types (canonly be used in electrochemical systems that do not involvehigh pH environments);26h,i,30i,37Professor Tongwen Xu (University of Science and Technology ofChina) received his BSc (1989)and MSc (1992) from HefeiUniversity of Technology and hisChemical Engineering PhD(1995) from Tianjin University.He then studied polymer scienceat Nankai University (1997). Hewas visiting scientist at University of Tokyo (2000), TokyoInstitute of Technology (2001)and Gwangju Institute of Scienceand Technology (Brain Pool Program Korea award recipient). Hehas received a “New Century Excellent Talent” (2004) and an“Outstanding Youth Foundation” (2010) Chinese awards. Hisresearch interests cover membranes and related processes,particularly ion exchange membranes and controlled release.This journal is The Royal Society of Chemistry 2014Energy & Environmental Science(c) Guanidinium systems;16c,38(d) P-based systems types including stabilised phosphoniums [e.g. ,39and P–N systems such as phosphatranium16d and tetrakis(dialkylamino)phosphonium40 systems;(e) Sulfonium types;41(f) Metal-based systems where an attraction is the ability tohave multiple positive charges per cationic group.42General comments on the characterisation of AEMsGiven that OH forms of AAEMs quickly convert to the lessconductive CO32 and even less conductive HCO3 forms whenexposed to air (containing CO2 – see eqn (1) and (2)), even forvery short periods of time,25d,43 it is essential that CO2 is totallyexcluded from experiments that are investigating the propertiesof AAEMs in the OH forms. This includes the determination ofwater uptakes, dimensional swelling on hydration, long-termstabilities, and conductivities [see speci c comments in thebelow sections].OH CO2 # HCO3 (1)OH HCO3 # CO32 H2O(2)Additionally, when converting an AEM or AEI into a singleanion form, it is vital to ensure complete ion-exchange. An IEMcannot be fully exchanged to the desired single ion form a eronly 1 immersion in a solution containing the target ion, evenif a concentrated solution containing excess target ion is used:the use of only 1 immersion will leave a small amount of theoriginal ion(s) in the material (ion-exchange involves partitionequilibria). IEMs must be ion-exchange by immersion inmultiple (at least 3) consecutive fresh replacements of thesolution containing an excess of the desired ion. Traces of theoriginal (or other contaminant) ions can have implicationsregarding the properties being measured.44Professor Lin Zhuang
Albuquerque, NM, 87131-0001, USA cCellEra, Caesarea Business and Industrial Park, North Park . energy density batteries, and new materials and processes for advanced interconnects for integrated circuits. He has 250 papers, is past Editor of JES and ESSL, past Director MARCO Interconnect Focus Center, and President of the Electrochemical Society (2014–15). 3136 Energy Environ. Sci.,2014 .