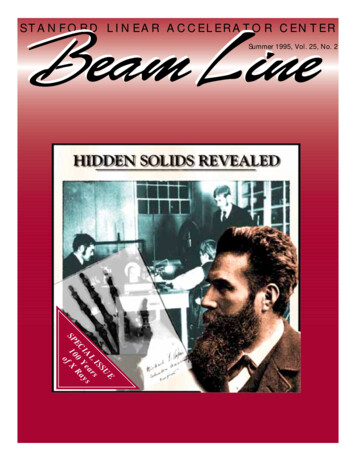
Transcription
STANFORD LINEAR ACCELERATOR CENTERSummer 1995, Vol. 25, No. 2SPESUISL arsIA Ye aysEC 0 R10 f Xo
A PERIODICAL OF PARTICLE PHYSICSSUMMER 1995VOL. 25, NUMBER 2page 4EditorsRENE DONALDSON, BILL KIRKEditorial Advisory BoardJAMES BJORKEN, ROBERT N. CAHN,DAVID HITLIN, JOEL PRIMACK,NATALIE ROE, ROBERT SIEMANN,HERMAN WINICKIllustrationsTERRY ANDERSONProduction EditorRAY ISLEDistributionCRYSTAL TILGHMANpage 10The Beam Line is published quarterly bythe Stanford Linear Accelerator Center,PO Box 4349, Stanford, CA 94309.Telephone: (415) 926-2585INTERNET: beamline@slac.stanford.eduBITNET: beamline@slacvmFAX: (415) 926-4500SLAC is operated by Stanford University under contractwith the U.S. Department of Energy. The opinions of theauthors do not necessarily reflect the policy of theStanford Linear Accelerator Center.Cover: A photo montage created electronically by graphicartist Terry Anderson for this issue commemorating the discovery of X rays 100 years ago.page 35Printed on recycled paper
CONTENTSFEATURES4page 25HOW THE TWENTIETH CENTURY STARTEDAHEAD OF TIMEThe centennial encounter of a physicist.Philip Morrison10EARLY HISTORY OF X RAYSThe discovery of X rays in 1895 was the beginningof a revolutionary change in our understandingof the physical world.Alexi Assmus25MEDICAL APPLICATIONS OF X RAYSA brief review of a century of radiology.Otha W. Linton35THE IMPACT OF SYNCHROTRON RADIATIONON MATERIALS RESEARCHSynchrotron radiation has transformed the roleof X rays as a mainline tool for probing the atomicand electronic structure of materials and theirsurfaces.Arthur Bienenstock & Arthur L. Robinson45THE X-RAY UNIVERSEX-ray images of the Universe are strikinglydifferent from the usual visible-light images.Wallace H. Tuckerpage 47DEPARTMENTS2FROM THE EDITORS’ DESK51THE UNIVERSE AT LARGEOn Beyond XVirginia Trimble60CONTRIBUTORS63DATES TO REMEMBERpage 51
FROM THE EDITORS’ DESKTHIS ISSUE of the SLAC Beam Line is unusual inseveral ways. First, at 64 pages it is the longest issuethat we have yet produced. Second, rather than acollage of different physics topics it concentrates on a singletheme: the centennial celebration of Wilhelm Roentgen’sdiscovery of X rays in 1895. Third.well, let’s back up a bitbefore we write down what comes third.This issue begins with an article by Philip Morrison, whotells us, from a physics perspective, “How the 20th centurystarted ahead of time” with the discoveries of X rays,radioactivity, and the electron. This issue ends with anarticle by Virginia Trimble, who tells us how astrophysicsmay go “On beyond X” into the next century to view theUniverse in the light of gamma rays, neutrinos, andgravitational radiation.Thus Morrison and Trimble are, in several senses, thebookends for this issue, and what a pair of bookends they are! It is probably not true thatPhilip Morrison and Virginia Trimble2SUMMER 1995
have read everything and know everything. It is probablyonly half true. But even the polymathic minds are not thefull story. To know it is a necessary but not sufficient condition of being able to say it or write it. If there is an exemplarof all that is best in English prose style, it is probably someone like Bertrand Russell, and reading Morrison and Trimblegives rise to much of the same pleasure that one takes in theclarity, grace, and wit of the prose.But enough already. Part three of the special character ofthis issue is to welcome Phil Morrison as a first-time contributor to our journal, and also to emphasize our continuingpleasure in the superb articles that Virginia Trimble produces for each issue of the Beam Line in “The Universe atLarge.”BEAM LINE3
How the Twentieth CenturyStarted Ahead of TimeThe Centennial Encounterof a Physicistby PHILIP MORRISONENRICO FERMI is said to have evaded somequery about a new particle with thisrebuff: “Young man, if I couldremember the names of allthose particles I wouldhave become abotanist.”(It is true thatthe first really newparticle, the neutrino,was first understood—and,in fact, was given its Italianname—by Fermi himself.)4SUMMER 1995
BEAM LINE5
X-ray photographof a snail shelltaken by SLACphysicist HobeyDeStaebler at theStanford PhysicsDepartment in1962 ( 2.4).If I could have recalled dates well,I might have become a historian!Many physicists share my inner needfor such approximation, and so it isnot really remarkable that twentiethcentury physics itself began a fewyears early, on New Year’s Day of1896. On that day Professor WilhelmRoentgen mailed from his university at Würzberg the preprints of hisforty-ninth paper. (His first fortyeight are less well known.) He included an X-ray photo of his ownhand, a piece of bone-hard evidencefor the new penetrating radiation. Somuch the books tell us.My own encounter with the dawnof twentieth century physics was personal, but of course second-hand.Even so veteran a member of the APSas myself doesn’t go all the way backto Gibbs and Helmholtz. But theanecdote makes vivid connectionwith Roentgen on this present occasion, the hundredth anniversary ofhis recognition of X rays.The story unfolds in an unexpected location in space-time, Oklahoma City, Oklahoma, about 1976.I found myself in Oklahomathrough the formidable persuasivepowers of Jerrold Zacharias, physicist and impresario at MIT. Zach hadalready drawn me to MIT yearsbefore. He was so energetic and effective as an organizer and standardsetter for science education at every6SUMMER 1995level that he was then a very fountain of opportunity to do good forphysics students anywhere. Thistime it was the Oklahoma City University that would provide me anaudience for a rousing popular talkon something new in physics. I cannot really recall my topic; pretty surely it was about quasars or supernovaeor the microwave background, something out of current astrophysics,presented for the interested butunspecialized student of physics.The details were elided by Zach;we always worked on mutual trust.There would be an evening publiclecture on the Oklahoma City campus to which I had acceded cheerfullylong before. But a luncheon meetingearlier in that day was my first encounter with my hosts. It wasmarked by experiences unique in allmy years of such little formalities.The luncheon setting was not atall novel; a lot of people sat at tablein some club or hotel dining room,whom I faced from my place at a longtable among a dozen or so who weresingled out for introduction. Whatwas novel was my luncheon partner,who was sitting beside me. He was aspry and articulate elder, and I soonlearned that this day was—at leastfor official purposes—his own hundredth birthday. Not only was he aman of unrivaled seniority, but hewas the focus of the whole event, my
X-ray photographof two springblooming daffodilstaken by HobeyDeStaebler ( 0.5).The interior ofRoentgen'slaboratory atWürzburg.lecture and all. A pillar of OklahomaCity life, he was a generous benefactor to the City University, and theowner, if I remember well, both ofthe city’s main newspaper and of itschief TV station. Plainly he was ruleron this day of all days.I was there, a visitor from MIT, tospeak about physics on campus at hisexpress request for a good lecture. Hehad very sound reason; the nowpowerful centenarian had been aphysics student while an undergraduate, and he still loved and admiredthe subject. He had drifted away intoa long career in journalism to reachan elevated level of achievement, buthe still wanted to talk physics whenhe could. At some point I came to askhim about his days as a student ofphysics so long ago, and he unfoldedthis wonderful narrative.He was then a student at ColoradoCollege in Colorado Springs. Onemorning in January 1896 he came tothe physics lecture room as usual.But the lecturer was filled with uncommon excitement. (Here I canonly paraphrase what I recall frommy hundred-year-old companion .)“Gentlemen,” began the lecturer,“something so unusual has happenedthat I want to seek your help. If youconsent, we will not simply go aheadwith the planned lecture. Instead wewill all work together in the lab toan amazing new purpose.“This morning’s newspaperbrought a reportthat a Germanprofessor has discovered an extraordinary newform of radiation, one so penetrating that for instance he is ableto photograph the bones within theliving hand, or a coin hidden insidethe pages of a thick book. The story is not very complete, but it saysenough about how it was done thatI think we could duplicate the resultswith apparatus we have right here inour college laboratory.“It would be wonderful to do that,and perhaps we might even be thefirst in all America to repeat his result, since we are getting started inthe first hours of the morning. Let’sget going; first we’ll collect what weneed and then set it up.“If we all work together we caneasily do the job by lunchtime. Willyou join me?”The delighted class set about thetask. The needed materials were allsoon found on the lab shelves: the bigsparky induction coil, the Crookestube, the fluorescent screens, thedarkroom materials, the filters ofblack paper.Soon it all came together. And it worked! By lunchtime my host recalled running overBEAM LINE7
Roentgen out walking later in life.to the ColoradoCollege chapel toborrow a largeBible in whosepages they couldhide a silver coin.The excitementwas unforgettable.Of course they were not to be thefirst in America to run the experiment. For the morning papers hadcarried the story very widely. Colorado had an irremediable handicap:its longitude. So far west, they werelate in starting, behind the manyphysics labs of the Eastern time zonea couple of hours as the earth turns.Many had had a similar idea, andsome of the Easterners would surelybe first. I have no real data on exacttimes or even dates, but I do knowthat Penn, Princeton, Columbia, Cornell, Harvard, Dartmouth, and others recall very prompt repetitionsof Roentgen’s wonderful result.This result came as though a seedcrystal had been dropped into a saturated solution! The new physicscrystallized out everywhere at once;the requisite apparatus was alreadythere in all serious labs around theworld. On the 20th of January, HenriPoincaré, who had received a NewYear preprint from Roentgen himself,showed the marvelous photos to thesession of the Paris Academy. HenriBecquerel was there, an expert onfluorescence like his father beforehim, and by March 2 Becquerel hadfound, largely by happy accident, thata uranium compound emitted somesuch active radiation spontaneously, without requiring exposure tolight or any other energizing input.Radioactivity had been discovered,8SUMMER 1995and the physics of the twentieth century had begun, for good and for ill.What a story! Yet it was to becapped that very evening. Of courseI could not fail to re-tell the physics student’s birthday story as a preface to my lecture. It went well, although certainly it was a digression.After my talk, a young man came upto speak to me. He was no undergraduate; he introduced himself asa physics postdoc at work for a yearor two in Oklahoma. His home wasGermany, where he had taken hisdoctor’s degree. What he told me wasa family story that he had first heardin his childhood, often told and retold in his presence. It was his elderlyuncle, a physician, who was the storyteller.That man had been a medical student in Würzberg in 1896. He tookphysics from Roentgen. One day theProfessor told his physics class of hisrecent work and demonstrated itbriefly. Now, it is an ancient customin the German universities for students to indicate high pleasure andapproval by remaining seated in placewhile beating their shoes smartly onthe floor. In the usual lecture theaterthere the seats rise up in rows stepafter step, to allow all to view the lecture table. The floor structure is thushollow and resonant, and the noiseof the footbeats is grand. The students that day approved mightily ofRoentgen’s miracle, and continuedtheir racket, so Uncle reported, forone full hour without stopping.Twentieth-century physics was madewelcome for the first time.It is curious that the best-knownfinding of twentieth century physicswas made in the same well-seeded
AP photocontext on the brink of WWII in January 1939. The celebrated Berlin experimenter Lise Meitner, newly exiled in Sweden, spent Christmas Dayin a park near Stockholm with a visitor from Copenhagen, her youngernephew, physicist Robert Frisch. Thetwo talked over the amazing new report from Meitner’s old lab thatdemonstrated that uranium upon irradiation by neutrons yielded radioactive products that included notonly the expected elements close touranium in atomic weight, but onethat was only about half as heavy. Atone point in the conversation theyboth came to an explanation andsoon mutually understood that uranium had fissioned into two heavyfragments, and that the fragmentsmust fly apart with unprecedentedenergy, to be detectable by the heavily ionized tracks they left in matter.Within two weeks Frisch had seenon the oscilloscope screen the unmistakable strong spikes of ionization they had expected.Their news came out even beforepublication, by word of mouth directfrom Niels Bohr, who had sailed offto a conference in Washington heldin the last week of January. Within days eager phone calls back tohome labs by the physicists who hadheard Bohr had induced the production of those very spikes in manyplaces (I saw them myself then atBerkeley); within weeks they werecertainly familiar all over the world.You had mainly to scrounge a smallamount of uranium compound in thechemistry storeroom. The fissionspikes were easy to find with thelittle ion chambers, oscilloscopes ofmodest gain, and weak neutron sour-ces that every serious nuclear physics lab then held, as forty-three yearsbefore every lab working with electrical discharges through gases already had its Crookes vacuum tubeand high voltage source on the shelf.History had repeated itself. Thefirst time the stunning discovery wasrather light-hearted, in those shadow photos through closed books andbony hands, but the second time itwas fateful. By the spring of 1940 sixgovernments, all of them already ator close to war, had each formed itsown initial organization to seeklarge-scale energy from uranium.For the last few years we havecome to share reason for hope thatthe hundredth anniversary of fission, when it arrives, will indeed becommemorated mainly among thephysicists, and not everywhere touniversal public dismay instead ofprolonged applause.This X ray image, taken in NorthProvidence, Rhode Island, Thursday,Feb. 16, 1995, shows a diamond ringthat was swallowed by a robber to foolpolice.BEAM LINE9
Early History of X Raysby ALEXI ASSMUSThe discovery of X raysin 1895 was thebeginning of arevolutionary changein our understandingof the physical world.IN THE WINTER of the year of his fiftieth birthday, and the yearfollowing his appointment to the leadership of the Universityof Würzburg, Rector Wilhelm Conrad Roentgen noticed a bariumplatinocyanide screen fluorescing in his laboratory as hegenerated cathode rays in a Crookes tube some distance away.Leaving aside for a time his duties to the university and to hisstudents, Rector Roentgen spent the next six weeks in his laboratory, working alone, and sharing nothing with his colleagues.10SUMMER 1995
Wilhelm Conrad Roentgen (1845–1923).(Courtesy of AIP Emilio Segré VisualArchives)that jolted the finde-siècle discipline out of itsmood of finality,of closing downthe books withever more precisemeasurements, oflosing itself in debates over statisticalmechanics, or of trying to ground allphysical phenomena inmathematically precisefluctuations of the ether.All three discoveries, X rays,uranium rays, and the electron, followed from one of themajor experimental traditions in thesecond half of the nineteenthcentury, the study of the dischargeof electricity in gases. All threecontributed to a profound transformation of physics. In the 20th century, the discipline has been grounded in the study of elementaryparticles.As with the invention of incandescent lightbulbs, the studyof electrical discharge throughgases was madepossible by thedevelopment ofimproved vacuum technologyin the 1850s. Early on, Englishscientists wereinvestigating thepatterns of lightand dark that appeared in sealedlead-glass tubes.The patterns inForms of tube used by Roentgenin 1895–1896 for the productionof X rays.German Museum, MunichThree days before Christmas hebrought his wife into his laboratory, and they emerged with a photograph of the bones in her hand and ofthe ring on her finger. The WürzburgPhysico-Medical Society was the firstto hear of the new rays that couldpenetrate the body and photographits bones. Roentgen delivered thenews on the 28th of December 1895.Emil Warburg relayed it to the BerlinPhysical Society on the 4th of January. The next day the Wiener Presscarried the news, and the day following word of Roentgen’s discoverybegan to spread by telegraph aroundthe world.On the 13th of January, Roentgenpresented himself to the Kaiser andwas awarded the Prussian Order ofthe Crown, Second Class. And on the16th of January the The New-YorkTimes announced the discovery asa new form of photography, whichrevealed hidden solids, penetratedwood, paper, and flesh, and exposedthe bones of the human frame. “Menof science in this city are awaitingwith the utmost impatience thearrival of English technical journalswhich will give them the full particulars of Professor Roentgen’s discovery of a method of photographingopaque bodies,” The New-YorkTimes began, and it concluded by predicting the “transformation of modern surgery by enabling the surgeonto detect the presence of foreignbodies.” (Jan. 16, 1896, p. 9)The public was enthralled by thisnew form of photography and curious to know the nature of the newrays. Physicians put it to immediateuse. Physicists sat up and took notice. The discovery of X rays was thefirst in a series of three discoveriesBEAM LINE11
German Museum, MunichRoentgen’s apparatus for studying theionization of air by X rays, 1906.Sir Joseph John Thomson, 1856–1940.(Courtesy of the AIP Niels Bohr Library)12SUMMER 1995these partially evacuated tubes werestimulated by a voltage drop betweena cathode and an anode: typicallythere was a dark space, calledCrookes’ dark space; then a glow,called negative light; then anotherdark space, this one called Faraday’s;and a final glow of positive light. Ifthe air in the tube was exhausted until the first dark space expanded tofill the entire tube and all glows disappeared, then the rays emitted fromthe cathode could be investigated.The rays cast shadows, and weredeflected by magnetic fields, butappeared to be immune to the effects of static electric forces.As was to be characteristic of thenew ray physics to come—the physics of cathode rays, X rays, alpha rays,beta rays, gamma rays, and N rays—the nature of the cathode rays was indispute, the British favoring a streamof particles, those on the Continentpreferring to think of them as somesort of disturbance of the ether. (TheBritish position, and the research program developed by J.J. Thomson atthe Cavendish Laboratory to studyionization in gases, would result inthe discovery of the electron. But ourstory does not take us that way).A strong reason for believing thatthe cathode rays were particles wasthe observation that they wouldnot pass through matter that wastransparent to ultra-violet light.When Heinrich Hertz found that hecould pass the rays through metalfoil, a fellow German scientist, PhilipLenard, began to study them morecarefully. Lenard designed a tubewith a thin aluminum windowthrough which the rays couldemerge, and he measured how farthey could travel and still inducefluorescence. Defined in this way,the range of the cathode rays was sixto eight centimeters. Lenard’s experiments inspired Roentgen to wonder if the rays in an attenuated formreally traveled farther, and heplanned experiments to see if asensitive electroscope would measure a discharge at four times thedistance Lenard had identified.This line of work was outsideRoentgen’s usual research pursuits,which had by this time gained himgreat stature in German science. Sonof a cloth manufacturer and merchant from the Rhine province,Roentgen was not a particularlydiligent student in his youth. Heeventually made his way to thePolytechnic in Zurich, where heobtained a diploma in mechanicalengineering in 1868 and a doctorate one year later. In Zurich hebecame an assistant to August Kundtand moved along with him to theUniversity of Würzburg, and then onto the Physical Institute at Strasbourg. His first move on his own wasto the chair of physics at Giessenin Hesse in 1879, from which hereceived many offers to go elsewhere.The path upward in the Germanuniversity system was to follow callsto universities of higher and higherstature, and finally to obtain aninstitute of one’s own. Roentgen
refused the calls until the University of Würzburg offered him theDirectorship of their Physical Institute. In 1894 he was elected Rectorat Würzburg. In his inaugural address, given the year before his discovery of X rays, Roentgen statedthat the “university is a nursery ofscientific research and mental education” and cautioned that “pride inone’s profession is demanded, but notprofessional conceit, snobbery, oracademic arrogance, all of whichgrow from false egoism.”*Roentgen’s pride could rest in theover forty papers he had publishedfrom Strasbourg, Giessen, andWürzburg. These early interestsranged widely—crystals, pyroelectrical and piezoelectrical phenomena,and the effects of pressure on liquidsand solids—but did not yet includeelectrical discharges in gases. He hadtaken his turn at measuring thespecific heat ratios of gases using asensitive thermometer of his ownmaking. He was an exact experimenter who often made his ownapparatus—a skill learned during histraining as an engineer in Zurich—and he was able to measure extremely small effects, surpassingeven Faraday’s measurement of therotation of polarized light in gases.Roentgen turned to a new interestin October of 1895: the study of cathode rays. In the course of repeatingthe experiments of Hertz and Lenard,he happened to notice a glowing fluorescent screen set off quite somedistance from the Crookes’ tube hewas operating. The screen sat muchfarther away than the six to eightcentimeters that Lenard had foundto be the maximum distance forwhich cathode rays maintain theirpower to induce fluorescence. Roentgen recognized the effect as worthy of his undivided attention anddevoted the next six weeks to itsuninterrupted study.Historians have speculated aboutwhy Roentgen was the first to recognize the significance of this effect.The equipment, a cathode ray tubeand a fluorescing screen, had been inuse for decades. In 1894 J.J. Thomsonhad seen fluorescence in Germanglass tubing several feet from thedischarge tube. Others had notedfogged photographic plates. Butbefore Lenard’s work, the object ofstudy was always the effects insidethe tube itself, and stray ultra-ultraviolet light could be used to explainthe fogging of photographic plates.Lenard’s great interest was in proving, in contradiction to the British,the ethereal nature of cathode rays,and he was the first to study thePhillip Lenard, 1862–1947. (Courtesy ofUllstein Bilderdienst and the AIP NielsBohr Library)Demonstration by Crookes that cathoderays travel in straight lines: a) cathode;b) aluminum cross and anode; d) darkshadow; c) fluorescent image.*Quoted in “Wilhelm Conrad Roentgen,”Dictionary of Scientific Biography(New York: Scribner’s, 1975), p. 531.BEAM LINE13
Heinrich Rudolf Hertz, 1857–1894.(Courtesy of Deutsches Museum andAIP Emilio Segrè Visual Archives)O, Röntgen, then the news is true,And not a trick of idle rumour,That bids us each beware of you,And of your grim and graveyard humour.We do not want, like Dr. Swift,To take our flesh off and to pose inOur bones, or show each little riftAnd joint for you to poke your nose in.We only crave to contemplateEach other’s usual full-dress photo;Your worse than “altogether” stateOf portraiture we bar in toto!The fondest swain would scarcely prizeA picture of his lady’s framework;To gaze on this with yearning eyesWould probably be voted tame work!No, keep them for your epitaph,these tombstone-souvenirs unpleasant;Or go away and photographMahatmas, spooks, and Mrs. B-s-nt!—Punch, January 25, 189614SUMMER 1995effects of the rays in air or in a second glass tube into which he directedthem.Roentgen, a meticulous and observant experimenter, made theobvious tests on the new X rays:Were they propagated in straightlines? Were they refracted? Were theyreflected? Were they distinct fromcathode rays? What were they? Likethe cathode rays, they moved instraight lines. Roentgen was unableto refract them with water and carbon bisulphide in mica prisms. Norcould he concentrate the rays withebonite or glass lenses. With eboniteand aluminum prisms he noted thepossibility of refracted rays on a photographic plate but could not observethis effect on a fluorescent screen.Testing further, he found that X rayscould pass freely through thick layers of finely powdered rock salt,electrolytic salt powder, and zincdust, unlike visible light which,because of refraction and reflection,is hardly passed at all. He concludedthat X rays were not susceptible toregular refraction or reflection.Roentgen found that the X raysoriginate from the bright fluorescence on the tube where the cathoderays strike the glass and spread out.The point of origin of the X raysmoves as the cathode rays are movedby a magnetic field, but the X raysthemselves are insensitive to themagnet. Roentgen concluded thatthey are distinct from cathode rays,since Lenard’s work had shown thatcathode rays passing through thetube maintained their directionbut were susceptible to magneticdeflection.Roentgen justified calling the newphenomena rays because of theshadowy pictures they produce:bones in a hand, a wire wrappedaround a bobbin, weights in a box,a compass card and needle hiddenaway in a metal case, the inhomogeneity of a metal. The ability of thenew rays to produce photographsgave them great popular appeal andbrought Roentgen fame. Many articles appeared in photography journals, and The New-York Times indexed the new discovery underphotography. Since the rays exposedphotographic plate, the public assumed they were some form of light.The physicist Roentgen concurred.Accepting Lenard’s claim that cathode rays were vibrations of the ether,Roentgen compared the new rays tothem and forwarded the opinion thatthe two were ethereal, although different from visible, infra-red andultra-violet light in that they did notreflect or refract. He suggested thatcathode rays and X rays were longitudinal vibrations of the ether ratherthan transverse ones.Now that their existence wasestablished, it was easy enough toexperiment with the new X rays.Roentgen himself published onlythree papers on the subject, but others jumped quickly into the field.And not just physicists. ThomasEdison used modified incandescentlight bulbs to produce the new rays.He boasted to reporters that anyone could make photographs ofskeleton hands; that was mere child’splay. Within a month of Roentgen’sannouncement doctors were usingthe X rays to locate bullets in humanflesh and photograph broken bones.Dr. Henry W. Cattell, Demonstratorof Morbid Anatomy at the University of Pennsylvania, confirmed their
Henri Poincaré, 1854–1912. (Courtesy ofAIP Emilio Segrè Visual Archives)importance for the diagnosis ofkidney stones and cirrhotic livers andcommented that “The surgicalimagination can pleasurably lose itself in devising endless applicationsof this wonderful process.” (TheNew-York Times, Feb. 15, 1896, p. 9).In the first six months after their discovery Viennese mummies were undressed, doctors claimed to have photographed their own brains, and thehuman heart was uncovered. By 1897the rays’ dangerous side began tobe reported: examples included lossof hair and skin burns of varyingseverity.Electricians and physicists speculated on the nature of these X rays.Albert Michelson thought theymight be vortices in the ether.Thomas Edison and Oliver Lodgesuggested acoustical or gravitational waves. But the rays ability to photograph was decisive, and seriousthinkers settled on three possibilities, all of them of electromagneticorigin: the waves were very high frequency light; they were longitudinalwaves (Roentgen’s initial suggestion);or they were transverse, discontinuous impulses of the ether.Quite early on the hypothesis thatthey were longitudinal waves wasdiscarded, despite the support ofHenri Poincaré and Lord Kelvin. Thecrux of the question was whether thewaves were polarizable. If so theycould not be longitudinal waves.Although the early experiments onpolarization were negative or unclear,with the discovery of another ray,Henri Bequerel’s uranium rays forwhich he claimed to have found polarization, those on the Continentset up a convincing typology. It wentfrom lower to higher frequencytransverse ethereal vibrations: light,uranium rays, X rays. Uranium rayswere given off by certain minerals,and they needed no apparatus to produce them, but they shared certainproperties with X rays. They exposedphotographic plates and they causedgases to conduct electricity.British physicists weighed in onthe side that X rays were impulses inthe ether rather than continuouswaves. Lucasian Professor of Mathematics at Cambridge, Sir GeorgeGabriel Stokes, and his colleague anddirector of the Cavendish Laboratory, J.J. Thomson, committed themselves to the impulse hypothesis in1896. It was consistent with theirconception of cathode rays as particles (Thomson was to announce thediscovery of the corpuscle or electronone year later.) The abrupt stop of acharged particle would result, after atiny delay, in the propagation outward of an electromagnet
physics students anywhere. This time it was the Oklahoma City Uni-versity that would provide me an audience for a rousing popular talk on something new in physics. I can-not really recall my topic; pretty sure-ly it was about quasars or supernovae or the microwave background, some-thing out of current astrophysics, presented for the interested but