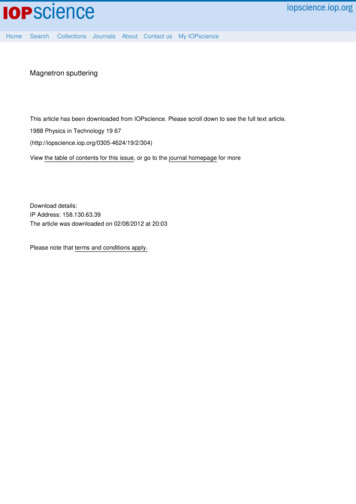
Transcription
HomeSearchCollectionsJournalsAboutContact usMy IOPscienceMagnetron sputteringThis article has been downloaded from IOPscience. Please scroll down to see the full text article.1988 Physics in Technology 19 ew the table of contents for this issue, or go to the journal homepage for moreDownload details:IP Address: 158.130.63.39The article was downloaded on 02/08/2012 at 20:03Please note that terms and conditions apply.
Phys Techno1 lS(1988l Printed in the UKMAGNETRON SPUTTERINGS SwannSputtering, once no more than a scientificcuriosity, is increasingly widely used toproduce thin coatings. Magnetronsputtering, in particular, shows how theapplication of simple physical principleshas led to a successful commercialtechnology.There are several physical vapour depositionmethods for producing coatings in a vacuum environment and these can be separated into two maingroups: (i) those involving thermal evaporationtechniques, where material is heated in vacuum untilits vapour pressure is greater than the ambientpressure, and (ii) those involving ionic sputteringmethods, where high-energy ions strike a solid andknock off atoms from the surface. Ionic sputteringtechniques include diode sputtering, ion-beam sputtering and magnetron sputtering.We are specifically concerned here with cathodicsputtering techniques where the ions are derivedfrom a plasma in a low-pressure gas between twoelectrodes. Sputtering as a phenomenon was firstobserved back in the 1850s but remained a scientificcuriosity until around the 1940s when diode sputtering was first used to any significant extent as acommercial coating process.However, diode sputtering suffers from very lowdeposition rates and in many applications was tooslow to make the process economic and was onlyused in applications where the special benefits ofsputtered films were justified. Then in the mid-03054624/88/020067 09 02 50 0 1988 IOP Publishing Ltd1970s a magnetically enhanced variant of diodesputtering emerged and this became known asmagnetron sputtering.Magnetron sputtering is a high-rate vacuum coating technique for depositing metals, alloys andcompounds onto a wide range of materials withthicknesses up to about 5 p m . It exhibits severalimportant advantages over other vacuum coatingtechniques and this has led to the developmentof a number of commercial applications rangingfrom microelectronics fabrication through to simpledecorative coatings.This article describes the sputtering process, andin particular the magnetron version: how it isachieved, its technological benefits and how theapplication of simple physical principles has led to asuccessful commercial process. The discussion begins with the special advantages of magnetron sputtering and is followed by a description of the basicdiode sputtering process, progressing to a description of the development of the magnetron principle.Why magnetron sputtering?Magnetron sputtering has emerged to complementother vacuum coating techniques such as thermalevaporation and electron-beam evaporation.However these techniques show certain disadvantages. In particular, alloys and refractory metalscause problems because of differences in alloy constituent vapour pressures and their high meltingpoints (the need to run sources very hot therebyaffecting your coated articles). In addition compounds can dissociate into their chemical constituents at the low evaporation pressures used.Magnetron sputtering overcomes these problemsand has many other advantages.The primary advantages are (1) high depositionrates, (2) ease of sputtering any metal, alloy orcompound, ( 3 ) high-purity films, (4) extremely highadhesion of films, ( 5 ) excellent coverage of steps andsmall features, (6) ability to coat heat-sensitive substrates, (7) ease of automation, and (8) excellentuniformity on large-area substrates, e.g. architectural glass. These points will be discussed later but it67
is immediately clear that sputtering is a very powerful technique which can be used in a wide range ofapplications.What is sputtering?Several terms may be met describing the sputteringprocess - cathodic sputtering. diode sputtering, K For DC sputtering. ion-beam sputtering, reactive sputtering - but all these are variants of the same physical phenomenon. Sputtering is the process wherebyatoms or molecules of a material are ejected from atarget by the bombardment of high-energy particles.More significantly. cathodic sputtering is the processdiscussed here and in this case the bombardment isby positive ions derived from an electrical dischargein a gas. Material is ejected from the target in such away as to obtain usable quantities of material whichcan be coated directly onto substrates.To obtain sputtering as a useful coating process anumber of criteria must be met. Firstly. ions ofsufficient energy must be created and directed towards the surface of a target to eject atoms from thematerial (figure 1). Secondly. ejected atoms must heable to move freely towards the object to be coatedwith little impedance to their movement. This is whysputter coating is a vacuum process: low pressuresare required ( i ) to maintain high ion energies and(ii) to prevent too many atom-gas collisions afterejection from the target. The concept of mean freepath (MFP)is useful here. This is the average distance that atoms can travel without colliding withanother gas atom.Figure 2 shows how M F P varies with pressure andit can be seen that in order to obtain relativelyunimpeded travel t h r o u a a gas it is necessary tooperate at pressures of 1 Pa (lO--'mbar) or better.Above this pressure material undergoes manygas collisions and deposition rates are very low.I-IHigh negative potentiolTorgetMaterial can also be deflected straight back onto thetarget and this reduces deposition rates further.Unfortunately the need to operate at low pressurecontrasts with the process required to produce thebombarding ions, namely that of plasma generation.All cathodic processes require a plasma or glowdischarge in order to work. The plasma may begenerated by DC.or K F power. The simplest systemuses tic. which will now be described ( K Fsystems willbe described later). Figure 3 shows the typical behaviour of conduction of electricity in a gas at lowpressure. Region A is the regime required for sputtering: here current densities are sufficiently high(-1 mAcm-') to make sputtering useful. This situation can relatively easily degenerate into an arcwhere voltage drops to very low values and thecurrent density rises rapidly. Sputtering power supplies must be specially designed to cope with thiseventuality.Considering a plasma in a low-pressure gas(figure 4) we find the tube appears to be divided intoseveral regions. The main regions of interest forsputtering use are the cathode dark space and thepositive glow. During stable conduction conditionselectrons are emitted from the cathode. These areaccelerated towards the positive electrode and whenthey have sufficient energy to ionise the gas molecules a glow appears characteristic of energy-leveltransitions in that gas. The positive gas ions formedthen move towards the cathode and impinge on itssurface. As a result of ion impact, secondary electrons and sputtered atoms are created. The electrons lead to further gas ionisation and atoms aresputtered away from the surface.As the accelerated electrons soon lose their energy in ionising collisions most of the applied voltagein a sputtering system appears across the cathodedark space. If the anode is moved closer to the4 Figure 1. Schematic diagram of i x d i o d e sputteringprocess chamber.Figure 2. Typical variation of mean free path withpressure.PlasmaregonterminaltPressure ( P a l-10-21026810010-210-4
IDark spaceGlass envelopeSputteringmmcCathode glowPositive column (striated)Figure 4. Charactcristic appearance of a g h discharge4 Figure 3. Voltage-currentcharacteristics of low-pressuregas.cathode until it reaches the dark space the plasmaextinguishes. ions cease to be produced and sputtering stops. This phenomenon is put to good use insputtering systems to prevent unwanted sputteringof components held at high voltage. e.g. the electrical connections to the target.As gas pressure is decreased with a glow dischargethe size of the dark space increases due to the largerM F P for electrons, and thus ions are produced faraway from the target material where they can beeasily lost to chamber walls. Similarly. primary electrons may reach the anode without producing gasionisation. Therefore the overall ionisation efficiency becomes low. Below approximately 1 Pa the plasma extinguishes and sputtering stops. Unfortunatelythis happens at pressures where mean free paths arejust becoming useful for a coating process.The solution to this problem is a method ofproducing more efficient ionisation at these lowerpressures and that solution is the magnetron design.Magnetron sourceThe magnetron uses the principle of applying aspecially shaped magnetic field to a diode sputteringtarget. The principle is that the cathode surface isimmersed in a magnetic field such that electron trapsare created so that E x B drift currents close in onthemselves. The principle was discovered as far backas the 1930s by Penning but has only been used inthe magnetron coating context for about fifteenyears.In essence the operation of a magnetron sourcerelies on the fact that primary and secondary electrons are trapped in a localised region close to thecathode into an endless ‘racetrack’. In this mannertheir chance of experiencing an ionising collisionwith a gas atom is vastly increased and so theionisation efficiency is increased too. This causes theimpedance of the plasma to drop and the magnetronsource operates at much lower voltages than diodesystems (500-600 V as compared with several k V ) .This greater ionisation efficiency leads directly to anincrease in ion current density onto the target whichis proportional to the erosion rate of the target.There are several types of magnetron designpossible. including post magnetrons. planar magnetrons, S guns etc. but these are only geometricvariants of the same physical principle. The commonfeature is that electron drift is controlled and electrons are trapped. The planar magnetron is a muchfavoured design because of its physical simplicityand the ability t o extend the cathode to virtually anysize required. Sources several metres in length canbe manufactured. Large cathodes are particularlysuited to continuous processing. However. sourcesare easy to fabricate into circular. rectangular or anycomplex shape: the only criterion is that an endlessracetrack must exist.It is most straightforward to consider the construction of planar magnetrons. Figure 5 shows aplanar magnetron in more detail and figure 6 showsthe appearance of ihree magnetron targets mountedin a vacuum system. Electromagnets or permanentmagnets may be used but for simplicity of designpermanent magnets are commonly used. These canbe of several different possible geometries but theessential feature is that the magnetic field linesform a tunnel shape in front of the target surface.I n order to achieve an efficient electron trap, fieldstrengths of a minimum of around 2OmT are usedbut are frequently much bigger than this.Figure 5 . Simplified schematic diagram of magnetronsource,Permanent magnetsMognetic f i e l d lines\1Water inwater out‘WatercooledCU\Negative69
The technological features of considerable interestto the process engineer are the target utilisation,the thickness of target material and film thicknessuniformity which may be obtained from the target.All these affect the economics of the process tosome degree and can make or break a design.Target utilisation is defined asv,,-v,x looo%v,,Figure 6 . View of three magnetron sources mounted inEdwards ESM100 system.Figure 7 shows several possible geometries whichcan be used and the choice will depend on the fieldstrength required and other factors such as efficiencyof target utilisation and simplicity of construction.The simplest form of magnet system employs asingle high-strength magnet with a ferromagneticpole-piece to extend the field into the correct shape.Because the field strength increases the efficiencyof electron trapping, high-magnetic-field systemswill generally operate at lower pressures, enablingoperation well down into th,e SOmPa region. Herethe process operates with characteristics more likevacuum evaporation, owing to the relatively highmean-free-path length.Considerations in magnetron designThere are several important features which must beconsidered in magnetron design. The first is that thecathode must conduct electricity efficiently. In addition a magnetron source is overall inefficient atconverting power into sputtered material. Perhaps80% (or worse) of the power consumed in plasmageneration is lost as heat, particularly at thecathode. Cathodes must therefore conduct heataway efficiently and are invariably water cooledto prevent overheating. Cathodes are often constructed of water-cooled solid copper for heat andelectricity conduction purposes.The source must also withstand the pressure differential stresses caused by vacuum operation and,in the case of large magnetrons, quite severe thermal stresses in the target material.70where V,, is the original volume of the target and V ,is its, volume when erosion grooves have just penetrated. In a magnetron design the plasma is localisedand concentrated into a region in front of the target.This region has the highest ion current density and itfollows that maximum ion bombardment occurs atthis point. A characteristic erosion groove is formedas the target wears away (figure 8). Clearly theshape of this groove will determine the target utilisation which can be obtained. The wider this grooveis, the better the final utilisation will be. This is ofimportance where expensive targets are used andalso in large machines where dissembly to changetargets must occur as infrequently as possible.The position of maximum erosion correspondsto near B, O (when the vertical component of themagnetic field is zero), so the position of this groovecan be tailored relatively easily. However, to obtaina wide erosion track it is necessary to have the fieldlines as parallel to the surface as is practical and yetstill maintain an electron trap to prevent loss ofelectrons at the edge of the target. The simplestmagnetic designs are not necessarily the best for thispurpose and quite complex systems of magnets andpole-pieces have been devised to improve thisbehaviour. Some designs have even used movingmagnets and variable magnetic fields to increaseutilisation. Even so, planar magnetrons seldom havegreater than 50% utilisation and figures of 20 to30% are not uncommon.Target thickness is significant because for continuous operation on industrial-scale equipment it isnecessary to be able to use thick targets to avoidFigure 7. Examples of possible magnet geometries inplanar magnetrons.
Surfuce after extensive erosim\'\IOriginat strface/11Figure S. Typical erosion prolilc of magnetron target (croshsection)changing targets too often. Magnetrons are oftenoperated in load-lock systems where the targets arekept under vacuum at all times in an oxidc-free stateand this too means that frequent target breakdownto atmosphere is undesirable. It is essential to have asufficiently strong magnetic field for efficient magnetron operation. As the target becomes thicker thetarget starts to behave more and more like a diodesputtering source with higher impedance.Also of major interest to the user is the filmthickness uniformity which may be obtained from asource. For a given size of source this will depend onpressure of operation. source-substrate distance.material being sputtered. substrate geometry andmotion, and source design. Figure Y shows the filmthickness which can be obtained at several sourcesubstrate distances from the same circular source.The magnetron is acting like a ring source and thebest film thickness region will occur at a specificdistance. It is of course possible to better this bymoving to much larger distances but the trade-off interms of deposition rate and film purity (due &excessive gas collisions) is too great. Most magnetrons have erosion track widths o f a maximum o f upto 100mm and so the source-substrate distancesused are typically in the region of 3(!-lOOmm. Thiscontrasts significantly with evaporative processeswhere source-substrate distances of 200mm o r moreare common.I n the case of large rectangular magnetrons thesubstrates are generally scanned past the targets sothe film thickness uniformity is improved by relativemotion. and source-substrate distance is less important. Working distances are then dictated by otherfactors such as heat load to substrates and deposition rate.Film thickness uniformity may also be improvedby using masks which are positioned between thesource and the substrate such that some material isintercepted. Masks may be used for static o r movingworkpieces and can improve distributions significantly.DCses because DC power supplies are simpler to manufacture than K F . In the rx mode the target is directlyconducting electricity and is subject to I'R lossesand may be operated up to currents of perhaps 70Wcm' averaged over the target. For IOOmm circularmagnetrons. power supplies will be capable of delivering up to 5 kW IIC' into the plasma. This canproduce deposition rates on static substrates ofseveral micrometres per minute making this verysuitable for high-production-rate processes. ThepowJer required is dependent on target size such thatlarge magnetrons may consume up to 5 0 kW.Examples of applications are ( I ) metallising formicroelectronic circuits and chip carriers. (2) electrical resistance films, e.g. Ni-Cr for strain gauges,( 3 ) magnetic films ( C O ,Fe, Co-Pt. Co-Cr, C w N ietc) for general magnetic storage devices, floppydiscs, tapes and thin-film magnetic heads, ( 3 ) optostorage devices. e.g. compact discs and video discs.( 5 ) corrosion-resistant films (Cr-Ni). (6) bondinglayers for various purposes. hermetic seals, brazingfor leadthroughs. glass fibres. and (7) gas sensors.In microelectronics fabrication processes sputtering has become the common method of coatingbecause of the ease with which alloys of materialsmay be processed and because of step coverage. Forexample it is necessary to produce conductive tracksin direct contact with the silicon wafer and on otherlayers of S i 0 2 and Si3N4 formed during the fabrication processes. Sputtering is particularly amenableto producing deposits of about l y m of aluminiumalloy containing a few per cent of silicon or copper.Figure 9. Variation of film thickness uniformity withsource-whatrate distance.%rce -substrate distancemagnetron sputtering processesIn the simplest of applications the magnetron is usedto deposit metallic materials by I)(' sputtering. The[xmagnetron is the cheapest of magnetron proces-IDtstonce across substrate lcm)71
Film/A-Rm. . . . . . . . . . . . . . . . . . . . . . . . . . . . . . . . . . . . .BiFigure 10. Illustration of step coverage on microcircuit: A .good coverage: B. poor coverage.This film must have good adhesion, uniformity and‘step coverage’. Step coverage is the ability to coatevenly over microscopic features on the silicon orGaAs wafer (figure LO) which are formed as partof the wafer manufacturing process by photolithographic techniques.Sputter sources give good coverage by virtue oftheir source size and because the process is occurring at relatively high pressure where some gaseousdiffusional transport of sputtered material occurs.This means that the vapour source does not propagate material in a rectilinear fashion and materialhas the ability to coat into more difficult geometrieswithout special substrate movement. I n electroplating terms the ‘throwing power’ is considerablyenhanced.Some microelectronic processes use substrateheating and/or biasing to alter the step coveragewhilst sputtering. Heating increases the surfacediffusion coefficient enabling reorganisation of thegrowing film and further improvements in stepcoverage.Biasing is the application of ii voltage to thesubstrate such that surface bombardment by positiveions occurs on the substrate as well as on the targetcathode. This causes resputtering o f the depositinglayer or at least an increase in surface energy. Theseeffects also control film growth and improve stepcoverage profile and can eliminate some commonlyobserved defects such as microcrack formation.Microelectronics fabrication machines usually employ a load-lock system where wafers are processedeither individually by a circular planar magnetron orscanned past rectangular magnetrons in batches.The choice of machine depends on throughputrequirements. The load-lock system ensures thatthe magnetron surface is not continually cycled upto atmospheric pressure giving longer pumpdown72cycles and contamination of the target surface by,e.g. oxygen.Presputtering or ‘conditioning’ of targets is required when a sputtering target is first pumpeddown. During this phase oxides and other surfacecontaminants such as grease are removed from thesurface and deposited onto shutters placed betweenthe target and substrate. This conditioning period isrequired every time a target sees a contaminatingreactive atmosphere and the load-lock system reduces or eliminates this requirement such thattargets are instantly ready to ‘fire’.In general. D(‘ magnetron sputtering is thereforeeminently suitable for microelectronics fabricationprocesses where compositional control. step coverage, film uniformity and ease of automation arerequired.Magnetic films are another important area formagnetron sputtering. Because the magnetron design relies on the presence of a magnetic field infront of the target i t is necessary to use very strongfields and/or thinner targets with magnetic materials. If this is not done the plasma impedanceincreases and the source operates like a diodesource.Magnetic thin films are used predominantly forreadiwrite storage of data for computer equipment.Storage may be by perpendicular recording. longitudinal recording or magneto-optical recording. Perpendicular recording materials such as Co-Cr have astrong perpendicular columnar isotropy which enables high information density and sharp cut-offbetween adjacent domain regions. Longitudinalmaterials have high coercivity and in-plane anisotropy. Ni-Co. CO-P. Cc-Pt. Co-Au are examplesproduced by sputtering.Magneto-optical discs rely on a reversal of magnetisation direction by heating the surface with alaser beam spot. Materials used have includedGd-Fe. T b F e - C o . Dy-Fe and Tb-Fe and there isconsiderable interest in this field at the moment.Magneto-optics could well be the major method ofinformation storage in the future and sputtering isthe favoured process.A classic example of the amenability of the sputtering process to large-scale production with entirelyautomatic machines is for optical storage discs.Video and compact discs have a thin layer (up to70nm) of aluminium sputtered onto the surface forreflectivity of the laser signal pick-up. This is donetypically in-line or in a single-disc-process system. Ineither case the target is kept under load-lock conditions such that it is immediately ready to ‘fire’. Asingle circular magnetron is a good configuration forcoating individual discs but large machines may haverectangular magnetrons with batches of discs scanning past o n linear workholders.
Figure 11. Characteristics of K Fplasma (after Butler and Kino 1963):see text below for explanation.I0Aluminium has the appropriate high reflectivity of 70% in the required wavelength band of 80(k830nm used to detect the optical pits on the discsurface. It also has good adhesion. minimum defectdensity and economy.A final example of the use of DC' sputtering is forbonding films and other metallurgical films. Sputtered films of Cr, Ti, Ni, Cu and Au have been usedsuccessfully to act as a basis for subsequent solderingand brazing treatments onto glass or ceramic substrates. Applications are, for example. bonding ofglass fibres into hermetic seals and special electricalleadthrough applications. The sputtered film is particularly suited to this purpose as a result of its veryhigh adhesion to the substrate as compared to evaporative processes. This enables subsequent bonding to be achieved without delamination or interfacefailure.I C magnetron sputtering can also be used forgeneral metallurgical films such as corrosionresistant or decorative coatings and any processwhere a metallic film is required with good adhesion.RFmagnetron sputteringDC' magnetrons will not work if an insulating target isused, because no current can flow through it. Thesolution to this problem is to use an alternating current at high frequency. K F sputtering wasdeveloped to enable the sputtering of dielectricmaterials and has the double advantage that it willsputter metals as well.When R F power is applied to a target it must becapacitively coupled such that a DC' sheath potentialis able to develop on the surface of the target. At thehigh frequency typically used for R F sputtering(-13.56 MHz) ions and electrons have vastly different mobilities in the fluctuating field. This meansthey physically move different distances during eachhalf cycle. The plasma I-V characteristics are asshown in figure 1 l ( a ) where an excess electron current is produced due to this difference in mobility.However in a capacitively coupled system no netcharge can be transferred so the electrode biases"negatively to compensate (figure 1 l(b)) such that ar x ' negative voltage is produced on the cathodesurface. Because it is capacitively coupled the electrode surface need not be a conductor and can be aninsulating material such as a ceramic or polymer.Because a [IC voltage is present. surface ion bombardment still occurs as with DC magnetron sputteringand so it is possible to sputter ceramic or insulatingmaterials as well as metallic materials. However R Fsputtering requires an impedance matching networkto ensure that the maximum power is absorbed intothe plasma. This. plus the higher complexity of R Fgenerators means that RF sputtering equipment ismore expensive to purchase than a i i c magnetron.Nevertheless KF. sputtering is essential for sputteringnonconducting materials so the increased cost istotally justified.The use of K F sputtering enables virtually anymaterial to be sputtered. Ionic and covalent compounds and even polymers may be sputtered away atrates depending o n their particular sputtering yield.There are certain limitations which must be considered however. Firstly dielectric materials are oftendifficult to fabricate and to obtain large targets canbe a problem. Secondly there are certain limitationsto sputtering of ceramics. Magnetron sputteringdoes put a significant heat load on the target surfaceand owing to the uneven nature of the ion bombardment the heat flow varies across the surface. Manyceramic materials have very low thermal conductivity and coupled with a low intrinsic shock resistancethis means that excessive heating causes catastrophicfailure. Hence when sputtering ceramics the powerlevels usually have to be much less than for I X .magnetron sputtering.Power levels of 10Wcm-' as opposed to70 W cm-' are a guide to the differences required.The difference is also noted in the sputtering yieldwhich for covalent and ionic compounds is generallymuch less than for metallic materials. The sputteringyield is defined as the number of atoms ejected froma target surface per incident ion. Dielectrics canhave sputtering yields only 10% of metals.This means that R F sputtering deposition rates for73
power ( K F or I X ) onto substrates may also be usedt o control film properties.Applications include cutting tools, drills etc. diesand punches, wire guides. tape heads and watchcases. Presently sputtering competes with cvr) (chemical vapour deposition) and PECL'D (plasmaenhanced .VI)) f o r some of these types of coatings.Passivating and insulating films and electrical resistance are another major area of application. Filmsof S i 0 2 . A l z O l . T a 2 0 i are used in microelectronicfabrication processes and in the fabrication of straingauges where an insulating sputtered film of Si02 orglass separates the resistance element from the basesupport. Films of Ni-Cr can be sputtered t o formhighly adherent strain-gauge elements.nonconductors are very much lower than for IXmagnetron sputtering thanks to power and yieldlimitations. However sputtering of compounds isstill an attractive process and has found favour inmany applications. Examples are superconductivefilms, dielectric films for semiconductors. passivating and insulating films. wear-resistant and hardcoatings, dielectric films for optics. polymeric films.transparent conducting films. integrated optic films.solar and energy-related films. T h e field of coatingswhich can be applied is so extenshe that it is difficultto describe them all. It is however clear that a n K Fsputtering system will enable the sputtering of bothmetallic and nonconducting materials which opensup a huge range of possibilities in coating researchand production.Superconducting materials exhibit a sudden transition t o virtually zero resistivity at a characteristictemperature called the critical temperature. Recentlyhuge interest in this field has been produced a sreported critical temperatures have 'soared' to aboveliquid-nitrogen temperatures. Superconducting filmssuch as NbN, Si3N4 and mixed oxide compositionscan be conveniently prepared b. sputtering fromfixed composition targets or by using sector-typecathodes to vary composition o r by co-sputteri
in a sputtering system appears across the cathode dark space. If the anode is moved closer to the 4 Figure 1. Schematic diagram of ixdiode sputtering process chamber. Figure 2. Typical variation of mean free path with pressure. Pressure (Pal -10-2 102 100 10-2 10-4 68