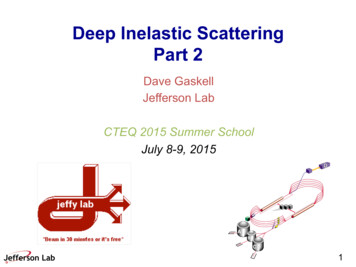
Transcription
Deep Inelastic ScatteringPart 2Dave GaskellJefferson LabCTEQ 2015 Summer SchoolJuly 8-9, 20151
OutlineDay 1:1. Introduction to DIS and the Quark Parton Model2. Formalismà Unpolarized DISà Polarized DIS3. Results and examplesDay 2:1. Nuclear Effects in DIS2. Beyond inclusive scatteringà Semi-inclusive reactions (SIDIS)2
Deep Inelastic Scatteringdσ4α 2 ( E / ) 2 /dΩdEQ4 22θ22θ W(ν,Q)cos 2W(ν,Q)sin1 222 Large Q2MW1 (ν ,Q 2 ) F1 (x)ν W2 (ν ,Q 2 ) F2 (x)Q2x 2MνF2 interpreted in the quark-parton model as thecharge-weighted sum over quark distributions:F2 ( x) ei2 xqi ( x)i3
Nuclear Effects in DISTypical nuclear binding energiesà MeV while DIS scales à GeV(F2Fe / ZF2p ( A Z ) F2n)(super) Naïve expectation:F2A ( x) ZF2p ( x) ( A Z ) F2n ( x)More sophisticated approachincludes effects from FermimotionF ( x) A2iM A / mNxdyfi ( y ) F2N ( x / y )Quark distributions in nuclei werenot expected to be significantlydifferent (below x 0.6)Figure from Bickerstaff and Thomas,J. Phys. G 15, 1523 (1989)Calculation: Bodek and Ritchie PRD23, 1070 (1981)4
Discovery of the EMC Effect First publishedmeasurement of nucleardependence of F2 by theEuropean MuonCollaboration in 1983 Observed 2 mysteriouseffects– Significantenhancement at small xà Nuclear Pions! (no)– Depletion at large x à the “EMC Effect” Enhancement at x 0.1 laterwent awayAubert et al, Phys. Lett. B123, 275 (1983)5
Confirmation of the EffectVOLUMEPHYSICAL51, NUMBER 7REVIEW LETTERSISLAC re-analysis of oldsolid target data usedfor measurements ofcryotarget wallbackgroundsR ochester-SLAC-(2&Q'&20lg — o SLACl. 2-Ml15 AUGUST 1 )IRochester- SLAG- MIT(E87)T(E49B)(g &Q(&20 (GeV/'c))0 FMC (9&Q &l70(GeV/c)(0.9&)SLAG (Cu/D)(0.9& Q'& l. 6 ( Bodek- RitchiSme Bodek- RitchieSmeori FeOplIIIà Effect for x 0.3confirmedà No large excess atvery low xllil0.9—0.8'&01.llPhoto production(Q 0, & l5 GeV)0.2II0.604x.(b)&& Photoproduction(Q O, v l5GeV),0.8l.000.20.40.60.8l.oerrors are shown. Point-to-point systematic errors havebeen added linearly (outer bars) where applicable. The normalization errors of 2.3% and 1.1% for cr A /oD (E49Bodek et50, All1431andPRL534 (1983)areal,not PRLfor W» 1.included.8 GeVand oF /aD (E87), respectively,data (1983)included.are 51,The data have been corforsmallrectedtheneutron excess and have not been corrected for Fermi-motion effects. The curve indicatesthe expected ratio if Fermi-motion effects were the only effects present (Ref. 11). High-Q2az, /oD data from EMO(Ref. 2), Iow-g o'Ay/ao and ac„/o'D data from Ref. 9, and photoproduction o'A /oD and oz, /oD data from Ref. 13 areshown for comparison. The systematic error in the EMC data is 1.5% at x 0.35 and increases to for thepoints at x 0. 05 and x 0.65.6FIG.(a)oA /O'Dand (b) or /aD vsOnly randomG%%uo
Subsequent MeasurementsA program of dedicatedmeasurements quicklyfollowedBCDMS (Fe)SLAC E139 (Fe)1.2EMC (Cu)A/DThe resulting data isremarkably consistent overa large range of beamenergies andmeasurement techniques10.800.1 0.2 0.3 0.4 0.5 0.6 0.7 0.8 0.91x7
Why is the EMC Effect Important? Neutron structure functions– Almost all the information we have on neutron structure functionscomes from deuterium data– Nuclear effects in deuterium relevant for extraction of neutroninformation – directly impacts PDFSNeutrino experiments– Neutrino experiments need nuclear targets– Extraction of information for nucleons requires understandingnuclear effectsUnderstanding QCD– Understanding the structure of the nucleon is obviously a keygoal– Understanding the force between nucleons and how nuclei areheld together also crucial– Why do “effective theories” work so well? At what point doquarks and gluons become relevant?8
Nuclear dependence of structurefunctionsExperimentally, we measure cross sections (and the ratios of cross sections)dσ4α 2 (E / ) 2 222θ22 θ F(ν,Q)cos F(ν,Q)sin21dΩdE /Q4ν 2 Mν2 σF M x R L 2 1 4 12 σ T 2xF1 Q 2AD2 F2 ( x) ei2 xqi ( x) i2Q 1 2 1 4M 2 x2F2A (1 RA )(1 RD ) DF2 (1 RA )(1 RD )In the limit RA RD or ε 1 2 tan21σA/σD F2A/F2DExperiments almost always display cross section ratios, σA/σDà Often these ratios are labeled or called F2A/F2Dà Sometimes there is an additional uncertainty estimated to account for the σà F2translation. Sometimes there is not.9
Properties of the EMC EffectGlobal properties of theEMC effectBCDMS (Fe)SLAC E139 (Fe)1.2EMC (Cu)A/D1. Universal x-dependence1Fermi motion0.800.1 0.2 0.3 0.4 0.5 0.6 0.7 0.8 0.91xanti-shadowingshadowingEMC-region10
x Dependence3A 3HeA 4HeD/1A/A41.2D1.21NMC0.80.8JLab E0310300.2SLAC E139HERMES0.40.6JLab E031030.8100.20.4x1.2Be0.81x1.2A 12 (14)C (N)DA 9D/1A/A0.61NMC (Be/C x C/D)0.8NMC0.8SLAC E139SLAC E139JLab E0310300.2JLab E031030.40.6x0.8100.2HERMES (N)0.40.60.81x11
x Dependence1.2A 271.2A 40CaD/1AA/DAl1NMC (Al/C x C/D)0.8NMC0.8SLAC E13900.20.40.60.81SLAC E13900.20.4x1.21.21A 197/208Au/PbDD/1A/A0.8xA 108/119Ag/Sn0.61NMC (Sn/C x C/D)0.8NMC (Pb/C x C/D)0.8SLAC E139 (Ag)SLAC E139 (Au)EMC (Sn)00.20.40.6x0.8100.20.40.60.81x12
Properties of the EMC EffectGlobal properties of theEMC effectBCDMS (Fe)SLAC E139 (Fe)1.2EMC (Cu)A/D1. Universal x-dependence2. Little Q2 dependence*10.800.1 0.2 0.3 0.4 0.5 0.6 0.7 0.8 0.91x13
x,the linear form C, (1 C& Q ). Figure 13 shows C& as afunction of x and indicates quantitatively that there is nosignificant Q dependence. Also shown for Fe and Ca isthe slope obtained combining our data with that ofBCDMS [3] and the New Muon Collaboration (NMC)781respectively, which also show no Q dependence.[6],Q2 Dependence of the EMC EffectAubert et a L / Nucleon structure functionsQJ.J.2 10-200GeV2EMC1.2x 0.05''' ' '"i; -1.0 , i, ,1 ,j ,x : 0.05SLAC E139 Q2 1-10 GeV2,EMC-0.8I .0 1.0"1.0 —"—" —"—x 0.081.21.0 0.004.1.0, , , ,, * x-- 0.1j50.81.2 '- -- * - , - e - e1.0 x : 0.25« .- ---*- Cx 03s0.0 - , - ----0.8 0.6 x 0.55 IZ Illlli6l102010.65 'Iz,OO.z ( G e V 2)lI100200 « «« « IA 0« \ « '««I 40.4001kate I \««« a«s«e«iI \ ««««II0.500 J «««0.300«««Lh« « iI1III1I1I0.700IiI0.600 iI0.400 I0.600iI0.700 I20I40 020QI-40 0iI2040(GeV/c )FIG. 12. Solid circles show (cr "/o );, as a function of Q forx Gomezvalues foretFeAu Rev.targetsD forexperiment.al,andPhys.49, this4348(1994)differentFerrors are statistical and point-to-point systematic added inafAubert et al, Nucl. Phys. B293, 740 (1987)quadrature.The ratio is for a hypothetical isoscalar rokenlinesbinsystematic errors were calculated as follows. The assumption was made thatFig. 13. The ratio F2(Fe)/F2(D ) as a function of Q2 at fixed values of x. The iron data are from ref.[2].TheThe0.500«««I01.00.8«««I0.220 090.8—1.00.6«««««« 0 10 x 0./,,50.8I0.90.8:1.0%FIiI 1.0 I09 as ———0.8—0.81.2'««I0.300x 0.220x 0.125I1.2 II0.9—0.80.6I«L1.2x 0.65IIarecorteralsnucove
(*) Q2 Dependence of Sn/CNMC Collaboration/Nuclear Physics B 481 (1996) 23-39lllrl]1.20.01 i iii1,11i i i i i i i i ] ' "1 x 0.015' ''"'"1' ''"'"10.015 lll. Ii ,i,,J. I35 ''l""l "ll'''"'"l'I ITII'I x 0.02 j,LL0.90.81.2I,0.04: :l,,,,,j.II-IIHJl2""1 x 0.05i t lHnl."1i illllnl' . I-IH.IJ ""10.05 x 0.06i l i,H.I.Ii ,lllllll."l3-,,,.I-''"q0.06 x 0.08, ,,,.,,i.I',', ,,,.I.INMC measured non-zero Q2dependence in Sn/C ratio atsmall x0 . 0 8 x 0.11.11-- 0.9 0.8'"q1.2 ''"'"1' '''""1-''"10.1 x 0 . 1 5.'"'1-'""1' '''""10.15 x 0.2' '"'"'1' ''"'"1-'""10.2 x 0.3I :',',110.3 x 0.41.11k.0.90.81.2à This result is in sometension with other NMC C/Dand HERMES Kr/D results-I nt*l-I '" 1i llllnl. Ii lHml . I0.4 x 0.5-liHdJ'"lL I Illtlll0.5 x I.L Ll llklo.6 x o.80.6-1( 2(Gev ) 10 21.110.90.85m.I1.I.IQ,(GeV ) 10 2-,,,,,I1. 1.I (GeV ) 10 2.I.1,,Q2(GeV ) 112Arneodo et al, Nucl. Phys. B 481, 23 (1996)Fig. 4. Structure function ratios FSnllff2 as a function of Q2 in different x bins. The error bars give thestatistical uncertainty. The solid lines represent the result of fits of the function FSn/lff2 a b In Q2 in eachx bin,Our results are consistent with those of previous measurements of the Q2 dependence ofFA 1 / F a , which however had uncertainties larger than the size of the presently observed15
Q2 Dependence at Large xJLab Results from Hall CQ2 2.3 GeV2W2 1.9 GeV2Q2 3.6 GeV2W2 2.4 GeV2Small angle, low Q2 à clear scaling violations for x 0.7, butsurprisingly good at lower x16
Scaling at Large xC/ DJLab Results from Hall C1.2JLab data from Hall Cà C/D ratio constanteven at large x for1.1W 2 GeVà The nuclear wavefunction smears the1cross section enoughto mimic “local duality”à Need to avoid theDelta resonance2Q 4.06Q2 4.50Q2 4.83W2 2.5 to 3.34 GeV2Q2 5.33Q2 6.050.90.20.30.40.50.60.70.80.9x17
Quark-Hadron Duality in Nuclei Free nucleon– average over resonanceregion DIS scaling limitBound nucleon– Fermi motion does theaveraging for us– Resonances much lessprominent in nuclearstructure functionsNuclear structure functionsappear to “scale” to lower Q2than their free nucleoncounterparts with no explicitresonance averagingJ. Arrington, et al., PRC73:035205 (2006)18
More detailed look at scalingC/D ratios at fixed xare Q2 independentforW2 2 GeV2 andQ2 3 GeV2JLab 6 GeV EMCdata scale up tox 0.85W2 2 GeV2W2 4 GeV2E03-103SLAC e13919
Properties of the EMC EffectGlobal properties of theEMC effectBCDMS (Fe)SLAC E139 (Fe)1.2EMC (Cu)A/D1. Universal x-dependence2. Little Q2 dependence3. EMC effect increaseswith Aà Anti-shadowing regionshows little nucleardependence10.800.1 0.2 0.3 0.4 0.5 0.6 0.7 0.8 0.91x20
A-Dependence of EMC Effect8N M C Collaboration Nuclear Physics B 481 (1996) 3-22.Y.C.F.NMC Collaboration Nuclear Physics B 481 (1996) 3-2219I'1.1i,''i,,,i, ,i ,,]1.10.9e DHeFeLi Be CAI ' . I PbI II tIIIItISYlilllDLiBeC HeCoFeSn P -'-- -0.9"-3x 0.01250.8Snx 0.0125II' I'p'I ' I'I 'I''II1.11.1DHeLi Be CAI Co FeSnPb0.9iD0.9 i-LiBeC HeAICoFeSn P BeC HeAICoFeSn PbIx 0.0450.8I:I t I I Ill IJHeLiBe CAI Co FeSn0.9PbDx 0.175IiIiiLi0.9x 0.1750.80.045 1.11.1DxiI I I I II {il l , i Ii10iiii0.02lllll100 nucl./fmi0.143]Structurefunction ratios versus n u c l e a r density p at x 0.0125, x 0 . 0 4 5 a n d x 0.175. The solidg. 8. Structure function ratios versus atomic weight A at x 0.0125, x 0.045 andFig.x 9.0.175.The lineslinesares hstatisticalo w the resultow the results o f fits to the data with the function F2A/lff2 cA (' -1) . The errors shownonly. o f a fit to the data with the function FA/Ff2 [3 p(A). The errors s h o w n arestatistical only.NMC: Arneodo et al, Nucl. Phys. B 481, 3 (1996)the data using the function FA/F2c cA C"-]) was performed in each x bin. Thecontinuous lines in Fig. 8 show the results of the fits for three x bins.The fitsa higherorder term proportional to A -2/3 yields a significant improvement of thedescribe the data satisfactorily.fit quality: the dashed line in Fig. 10 shows the result of the fit using the function21
(tr "/tr ),, d(x)[1 p(x}p(A)]. The average nuclearwasgivendensitybyp( A ) 3 & /4 &„&, 5(r )/3. The quantity (r ) is the rms electronscattering radius of the nucleus [48]. The values of p( & )J. GOMEZnucleons/fm ) used in the fits were 0.024 forof al.(in units etA-Dependence of EMC Effect4366IIIIIIIIIIIIIlllllx 0.22z Ab1.0 2}. TheIIIIIIIIvalues(1.Inparamempirical parametrizationIIIIII0.60(b)1.00.8—497. 237x 1013.129x 775. 767x —205. 872x0.600(b) 145.291x—1208. 393x0 0.90The1.0 fit values of C are close to unity everywheregood empirical parametrization0.9—is0.9 lnC(x} 0.017 0.018 lnx 0. 005(lnxIIIIIIIIIIIII10Nuclear Weight AIII100thistracteandmodeThby eof a(x) and C(x) are listed heavyin TIX, and the former is plotted in Fig. 19. The y Beperax 0.220(a)haveisshownin Fig. 19Alsoisgree of freedom(A1.0 a(x) —0. 070 2. 189x —24. 667xo 0.9Ft/FanZOU)These parametrizations also characterize the NMCon0.8He, C, andvalid in the ra0.05Ca [6]0.and10 are 0.only150.01(x &0. 88.NuclearDensityThe cross-section ratios can also be examinedFIG. function20. Ratiosof(cr"/crversus nuclearat and(a) Table2indensitynuclearFig.3)- densityFIG. 18. Ratios (o "/0");, versus atomic weight A at x(a) 0.pe2asRlines 5⟨r⟩/3the20ρ 3A/4πR220Someand (b) x 0.600.FIThesolidparepresentedescribed below, predict that the probabx 0.220 and (b) x 0.600. The solid lines are a parametriza-rametrization models,(cr"/od) d( )[1xP( )p(xA)]. The errorsaveragof nucleons systematic,within thenucleus(whicoverlap point-to-pointty of statistical,tion of the data in terms of (cr "/cr );, C(x) A '"'. The errorsshown includetarget(12).and r2 RMSscatteringradiusto thenuclearis relatedEtoelectronshown include statistical, point-to-point systematic, and targetto-target proportionalerrors.The overallfunctiuncertaintydensity)due to thedeuteriumratiosTheat the Qs-averaged(rr "1'o );,shownwto-target errors. The overall uncertainty due to the deuteriumincluded onlytarget is efFect.A 2 point.is includedat the A 2targetin terms of average nuclear densitySLACE139:onlyGomezet point.al, PRD 49, 4348 (1992)parametrizedTABLE IX. Fit coeScients versus x. The coefBcients are from the fits (0 "/cr );, C(x) A '"' and(o "iver d);, d(x) [1 p(x)p( A )] are shown for both coarse and fine x bins. The fits include A 2.22
JLab E03103REMC (FA2/FD2)/(A/2)E03103 in Hall C at Jefferson Lab ran Fall 2004à Measured EMC ratios for light nuclei (3He, 4He, Be, and C)à Results consistent with previous world dataà Examined nuclear dependence a la E139New definition of “size” of theEMC effectà Slope of line fit fromx 0.35 to 0.71.1 dREMC/dx 0.280 /- 0.02810.9Normalization (1.6%)0.30.40.50.60.70.80.9xDefinition assumes shape ofthe EMC effect is universalfor nucleià Data consistent with thisassumptionà Normalization errors meanwe can only confirm this at1-1.5% level23
EMC Effect and Local Nuclear Density9Behas low average densityà Large component of structure is2α nà Most nucleons in tight, α-likeconfigurationsEMC effect driven by local ratherthan average nuclear density“Local density” is appealing inthat it makes sense intuitively –can we make this morequantitative?24
Local Density à Short Range CorrelationsWhat drives high “local” density in the nucleus?More complex calculations start from realistic NN potentialsTensor interaction and short range repulsive core lead to highmomentum tail in nuclear wave function à correlated nucleons25
Measuring Short Range CorrelationsTo measure the (relative) probability of finding a correlated pair, ratios ofheavy to light nuclei are taken at x 1 à QE scatteringIf high momentum nucleons in nuclei come from correlated pairs, ratio of A/Dshould show a plateau (assumes FSIs cancel, etc.)2N SRC3N SRC2 σA a2 (A)A σD1.4 x 2 2 nucleon correlation2.4 x 3 3 nucleon correlation26
Weinstein et al firstobserved linear correlationbetween size of EMCeffect and Short RangeCorrelation “plateau”Correlation strengthenedwith addition of Berylliumdata-dREMC/dxEMC Effect and SRC0.520.4/ ndfp04.895 / 5197Au-0.08426 0.0038695612C0.3Fe9Be4He0.230.10.0HedO. Hen et al, Phys.Rev. C85 (2012) 047301-0.10123456a2(A/d)This result provides a quantitative test of level of correlationbetween the two effects27
Origin of the EMC Effect Observation of correlation between size of EMC effectand SRCs interesting – but still does not explain theorigin of the EMC effect Seems odd that an effect observed in QE scatteringperhaps has common origin with modification of quarkdistributions Nonetheless, this correlation can be used to gleaninformation about nucleon structure28
EMC-SRC correlationused by Weinstein et al toextract the “in-mediumcorrection” byextrapolating to a2 0-dREMC/dxEMC Effect in Deuteron from SRCs0.520.4/ ndfp04.895 / 5IMC à 5612C0.3Fe9Be4He0.2AnAu-0.08426 0.00386930.12A1970.0HedO. Hen et al, Phys.Rev. C85 (2012) 047301p-0.10123IMC(D) EMC(N) – EMC(D)456a2(A/d)dRIM C 0.079 0.006dx29
EMC Effect in DeuteriumFrom BONUS: F2n/F2pUse world data from FD2and F2pCJ12systematic errorsKulagin and PettiW 1.4 GeV; 4 GeV data24 5 GeV; W 1.4 GeV; Q 1 GeV2-0.10(5)x 1.03(2)1.061.04F2d/(F2p F2n)EMC effect in deuteriumalso recently extractedusing data from BONUSexperiment (seeyesterday)1.0210.980.960.940.92Size of EMC “slope” extractedfrom this analysis consistentwith that extracted from EMCSRC correlation0.90.10.20.30.40.50.60.70.8xGriffioen et al, arXiv:1506.00871 [hep-ph]30
Future of the EMC Effect A key question moving forward is exploration of the roleof SRCs in the EMC effect New observables may be needed to gain further insight– Flavor dependence of the EMC effect (valence andsea quarks)– EMC effect in polarized quark distributions? Quark-meson inspired coupling models of the EMCeffect seem to bridge the gap between quark andnucleon degrees of freedom– These models do not include or give rise to SRCs31
Semi-inclusive DISSIDIS à production of one ormore hadrons in DIS reactionSimple picture:1. Electron scatters from quarkin nucleon2. Quark is kicked out à subsequently hadronizes,ending up in bound stateIn this simple picture, SIDIS canbe used to “tag” the flavor of thestruck quark in DIS process(E,’ p’ )e(E, p)qγ*Nuudhhπ πFragmentation function32
Semi-inclusive DISIn principle SIDIS can also beused to gain information aboutspatial distributions of quarks innucleonsà This requires measurements/observation of transversedegrees of freedom(E,’ p’ )e(E, p)qγ*Nuudhhπ π33
SIDIS KinematicsUseful kinematics related to outgoing hadron:q·pEhz q·Pp·qp q cos Fraction of virtual photon energy transferred to hadronpT (p( q qk) · ( qk qp )22ph )ph 12Components of hadron momentumrelative to qAzimuthal angle between electronscattering plane and hadron reactionplane34
SIDIS Cross SectionGeneral form of unpolarized cross section:2dy2 FT FL 22dxdydzdpT dxyQ 2(1)2 (1 ) cos FLT cos 2 FT TIntegrate over pT and ϕ, cross section can be expressed in terms of quarkparton model:d dxdydz1DISdDISdzd dzwhere22heq(x,Q)Df (z)f q f22)eq(x,Qf f f35
Fragmentation FunctionsDqh(z,Q2) à probability to form hadron (h) after quarkof flavor (q) is struckIn Quark-Parton Model, fragmentation functions Q2 independentà In reality, evolve with Q2 like quark PDFsFragmentation functions can be measured in e e- reactions à nocomplication due to hadron structureà Only average FF accessible – need other information for flavordependenceFor pion production, charge and isospin symmetry reduce number of FF’s needed D Du Dd D Du Dd Dū Dd Dū Dd 36
à Assumes leading orderfactorizationà Do not need knowledge ofabsolute fragmentation functions,but do need FF ratio: D-/D D favored fragmentationfunction (uà π )D- unfavored fragmentationfunction (uà π-)0.60.50.40.30.20.10 -2101.41.210.80.60.40.20-0.2 -21010-1d-uLight quark sea flavor asymmetrycan be extracted using semiinclusive pion production(d-u)/(u-d)SIDIS Examples1x22HERMES Q 2.3 GeV22E866 Q 54.0 GeV10-11x37
Strange quarkdistributions from(K K-) productionxS(x)SIDIS Examples22〈Q 〉 2.5 GeV0.42HERMES with DKS(z,Q )dz 1.27FitCTEQ6L––x(u(x) d(x))S(x) s(x) s̄(x)CTEQ6.5S-0NNPDF2.30.200.020.1Phys.Rev. D89 (2014) 9, 097101S(x)DSK (z, Q2 )dzK2dN(x,Q)2Q(x, Q ) 5dN DIS (x, Q20.6xKDQ(z, Q2 )dz38
SIDIS Examplesx u0.2Polarized quark distributionsd0hx d1 32(2)dxdQ2 dze2q q ( ) Dqh (z, Q2 )-0.2qq(x) q (x)0q (x)0–-0.2HERMES used a “purity” analysis sothey wouldn’t have to measure theabsolute magnitude of fragmentationfunctionx u–x d0-0.20-0.2x s0.030.10.6xHERMES, Phys.Rev. D71 (2005) 01200339
SIDIS with Transverse Degrees ofFreedomIn the more general case, allowing fortarget and beam polarizations, the crosssection is a bit more complicatedà Measurement of the transversemomentum of the hadron also allowsfor access to information regarding theinitial transverse momentum of thequarkà Transverse momentum dependentdistributions – TMDsà Azimuthal asymmetries key toaccessing TMDsSTk′kSPh qPhExample: Transversely polarized target, unpolarized beam2dy2sin ( hsin ( hS) S [sin()F FunpolhSU T,TU T,Ldxdyd S dzd h dp2hxyQ2 2(1)sin ( )sin (3 hS)S sin ( h S )FU T h S sin (3 h2 (1 ) sin S FUsin S )FU TTsin (2 hS)2 (1 ) sin (2 h]S )FU TS)40
TMDsIn the inclusive/forward hadron production case, we sample onedimensional parton distribution functionsTMDs allows us to explore the distributions of partons in the transversedirectionq(x)q(x, kT )Transverse momentumalso generated duringfragmentationD(z)D(z, pT )41
TMDsq(x)q(x, kT )D(z)D(z, pT )Probability of producing hadron withtransverse momentum PT comesfrom a convolution of kT dependentparton distribution and pT dependentfragmentation functionPT pT zkT 22O(kT /Q )42
SIDIS and gmentationfunctionBeam polarization 3FU Ucos (2 h )FU Usin (2 h )FU LFLLsin ( hFU T,TS)sin ( h S )FU TTarget polarizationsin (3 hS)FU Tcos ( hS)FLTf1 D1h1 H 1h1L H1g1L D1f1T D1Unpolarized TMDBoer-Mulders TMDWorm gear TMDHelicityTMDSivers TMDh1 H 1Transversity TMDh1T H1Pretzelosity TMDg1T D1Worm gear TMD43
Distribution FunctionsN/qULTquarksUh1f1U unpolarizedL long. polarizedg1h1LLT trans. polarizedTf1T g1T h1 h1TnucleonDiagonal elements usual PDFsOff-diagonal elements transverse momentum distributions, requirenon-zero angular momentumf1Tà Sivers function, describes unpolarized quark in trans. pol. nucleonh1 , h1L , h1Tà Boer-Mulders functions describe transversely polarizedquarks in un/long./trans./polarized nucleon44
Unpolarized SIDISHall C @ JLAB: E00-108Measured PTdependence ofunpolarized SIDIScross sections for:π and π- fromH and D45
Model PT dependence of SIDISGaussian distributions for PT dependence, no sea quarks, and leading order in (kT/q)Inverse of total width for each combination of quark flavorand fragmentation function given by:Simple model, with several assumptions:à factorization validà fragmentation functions do not depend on quark flavorà transverse momentum widths of quark and fragmentationfunctions are Gaussian and can be added in quadratureà more 46
Unpolarized SIDISµ2u 0.07 0.03 (GeV/c)2µu µdµ2d 0.01 0.05 (GeV/c)2µ µ-47
A1 PT-Dependence in SIDISm02 0.25GeV2mD2 0.2GeV2M.Anselmino et alhep-ph/0608048In perturbative limit predicted to be constantNew eg1dvcs data allowmultidimensional binning to studykT-dependence for fixed xπ ALL can be explained in terms of broader kTdistributions for f1 compared to g148
Proton Single-Spin Asymmetries with CLASTransversely polarizedquarks in the longitudinallypolarized nucleonW2 4 GeV2Q2 1.1 GeV2y 0.850.4 z 0.7MX 1.4 GeVPT 1 GeV0.12 x 0.48ep e’pX 10% ofE05-113 dataMuch higher statistics from 2009 run49
Transverse Target AsymmetriesCollins asymmetrysin (h S)h1 H 1Provides access to “transversity” distribution à linked to tensor charge ofthe proton1q 0hq1 (x)Sivers asymmetryFundamental property of nucleon,sin (hS)f1T D1Quark distributions in a transversely polarized nucleon50
Transverse Target asymmetries fromCOMPASSCollins asymmetriesPLB 744 (2015) 25051
Transverse Target asymmetries fromCOMPASS and HERMESSiversasymmetriesPLB 744 (2015) 25052
SIDIS Summary Semi-inclusive DIS a powerful tool for exploring howquarks are distributed in the nucleon– Flavor tagging for polarized and unpolarized PDFs– TMDs allow exploration of transverse structure à linkto orbital angular momentum Most SIDIS data has been acquired at fixed targetfacilities à HERMES, JLab, COMPASS– JLab has a large SIDIS program planned for Halls A,B, and C as part of 12 GeV Upgrade– A future EIC would provide a huge amount of data inthe “sea-quark” regime53
EXTRA54
DIS Cross SectionReminder: Inclusive cased4 2 22 yxF(x,Q) (1122dxdQxQy)F2 (x, Q2 )Quark parton-modelF2 2xF1F2 (x) xd2 2 [1 (124dxdQxQd2 2 xs [1 (14dxdyQe2f qf (x)fy)2 ]e2f qf (x)fy)2 ]e2f qf (x)f55
CJ12 PDFsNuclear effects in deuteron lead to significant uncertainties in quark PDFs atlarge xà This has been studied in some depth by the CTEQ-JLAB collaborationJ. F. Owens, A. Accardi and W. Melnitchouk, Phys. Rev. D 87, 094012 (2013)56
1 Deep Inelastic Scattering Part 2 Dave Gas