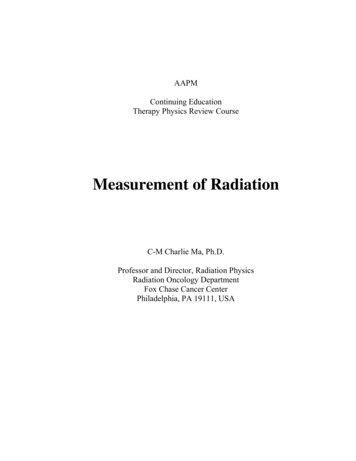
Transcription
AAPMContinuing EducationTherapy Physics Review CourseMeasurement of RadiationC-M Charlie Ma, Ph.D.Professor and Director, Radiation PhysicsRadiation Oncology DepartmentFox Chase Cancer CenterPhiladelphia, PA 19111, USA
Table of ContentsTABLE OF CONTENTS . 1DEFINITION . 2IONIZATION . 2IONIZING RADIATION . 2DIRECTLY AND INDIRECTLY IONIZING RADIATION . 2PARTICLE FLUENCE . 2ENERGY FLUENCE . 4MASS ENERGY TRANSFER COEFFICIENT . 4MASS ENERGY ABSORPTION COEFFICIENT . 4KERMA . 5COLLISION KERMA . 6ABSORBED DOSE . 7EXPOSURE . 8Measurement of Exposure . 10CHARGED PARTICLE EQUILIBRIUM . 14CPE for external photon sources . 14CPE, Dose and Collision Kerma . 16CPE: Dose and Kerma. 17Break Down of CPE . 17CAVITY THEORY . 18BRAGG -GRAY CAVITY THEORY . 18BRAGG -GRAY CAVITY THEORY . 19SPENCER-ATTIX THEORY . 21KILOVOLTAGE X-RAY CALIBRATION (TG-61) . 23ABSORBED DOSE DETERMINATION FOR LOW-ENERGY X-RAYS (UNDER 150 KV) . 23ABSORBED DOSE DETERMINATION FOR MEDIUM-ENERGY X-RAYS (100-300 KV). 23MEGAVOLTAGE PHOTON & ELECTRON CALIBRATION (TG-51) . 24EQUIPMENT NEEDED. 25BEAM SPECIFICATION . 25REFERENCE CONDITIONS . 27STEP-BY-STEP PROCEDURE TO DETERMINE DW IN A PHOTON BEAM . 28STEP-BY-STEP PROCEDURE TO DETERMINE DW IN AN ELECTRON BEAM . 28MEASUREMENT OF ABSORBED DOSE . 31CALORIMETRY . 31IONIZATION CHAMBER DOSIMETRY . 32Thimble Chambers . 32Parallel-Plate Chambers . 33Charge Measurement . 33CHEMICAL DOSIMETRY . 35Ferrous Sulfate (Fricke) Dosimeter. . 35SOLID STATE METHODS . 36Thermoluminescence dosimeters . 36Film Dosimetry . 39Semiconductor detectors. 39SAMPLE QUESTIONS . 40ACKNOWLEDGMENTS . 401
DefinitionIonizationIonization is a process in which one or more electrons are liberated from a parent atom ormolecule or other bound stateIonizing radiationIonizing radiation consists of charged particles (for example, positive or negative electrons,protons, or other heavy ions and/or uncharged particles (for example, protons or neutrons)capable of causing ionization by primary or secondary process.Directly and Indirectly Ionizing Radiation1. Directly ionizing radiation. Fast charged particles, which deliver their energy to matterdirectly, through many small coulomb-force interactions along the particle's track.2. Indirectly Ionizing Radiation. X- or γ-ray photons (i.e., uncharged particles), which firsttransfer their energy to charged particles in the matter through which they pass in arelatively few large interactions. The resulting fast charged particles then in turn deliver theenergy to the matter as above.It will be seen that the deposition of energy in matter by indirectly ionizing radiation is thus atwo-step process.Particle FluenceThe ICRU defines particle fluence as the quotient of dN by da, where dN is the number ofparticles incident on a sphere of cross-sectional area da. with units of M-2 (1)dNdaCare should be taken to distinguish fluence from planar fluence, which is the number ofparticles crossing a plane per unit area.2
In the two cases shown in the figure, the particle fluence is the same because the number ofparticles hitting the sphere is the same in both cases whereas the planar fluence decreases whenthe beam is not at the normal incident.An alternative formulation of particle fluence is that it is equal to the sum of the particle tracklengths in a volume, divided by the volume. This alternative formulation is equivalent to theformal definition and we have Tracklengt hs in volu me volume(2)Consider an electron passing through a slab of area dA cm2 and thickness t cm. For themoment, ignore the creation of secondary electrons. The path length in the volume is t/cos( ).The electron mass collision stopping power, (S/ )col, gives the energy lost to electrons in thematerial per unit path length (in g cm-2) the energy deposited in the slab is given by:E dep tcos (3) S / colIn this simple case, the particle fluence (path length per unit volume) is given by:t costdA 1dA cos Substituting this into equation (3) givesEdep dA t S / col(4)From this we findD Edepmass dA t S / col tdA S / col(5)This simple relationship between fluence and dose holds for arbitrary volume and fluence inany direction.The derivation assumes that radiative photons escape from the volume of interest andsecondary electrons are absorbed on the spot. This latter condition does not hold, but inconditions of charged particle equilibrium of secondary electrons, the result is still valid3
because energy transported out of the volume by knock-on electrons is replaced by similarones coming in.Energy FluenceThe energy fluence is the quotient of dR by da, where dR is the radiant energy incident on asphere of cross-sectional area da. dRda(6)Unit: JM-2Mass Energy Transfer CoefficientThe mass energy transfer coefficient, tr/ of a material for uncharged ionizing particles, is thequotient of dEtr /EN by PDL where E is the energy of each particle (excluding rest energy), Nis the number of particles, and dEtr/EN is the fraction of incident particles energy that istransferred to kinetic energy of charged particles by interactions in traversing a distance dl inthe material of density . tr1 dE tr EN dl(7)Unit: M2kg-1Mass Energy Absorption CoefficientThe mass energy absorption coefficient, of a material for uncharged ionizing particles is theproduct of the mass energy transfer coefficient, and (1-g), where g is the fraction of the energyof secondary charged particles that is lost to bremsstrahlung in the material. en tr 1 g (8)Unit: M2kg-1Energy Transferred, EtrThe energy transferred in a volume, V is by definitionE tr (R in ) u - (R out ) nonr Qu(9)where (Rin)u is the radiant energy of uncharged particles entering V, (and radiant energy is theparticles energy ignoring the rest mass); (Rout)unonr is the radiation energy of unchargedparticles leaving V, except that which originated from radiative losses of kinetic energy by4
charged particles while in V (i.e. except for the bremsstrahlung photons originating in V); and Q is the net energy derived from rest mass in V.Etr is just the kinetic energy received by charged particles in the volume V, regardless of howthey dissipate the energy.e T h h E tr (R in ) u - (R out ) nonr Qu hv1- hv2 0 TKermaThe Kerma K at a point of interest P in V is defined asK d E trdm(10)The kerma is the expectation value of the energy transferred to charge particles per unit mass ata point of interest.Kerma is defined for indirectly ionizing radiation only (i.e. photons and neutrons).For monoenergetic photons, the kerma is related to the energy fluence, by:Kwhere tr tr tr E is the mass energy-transfer coefficient. 5
Collision KermaLet the net energy transferred be defined for a volume V as:Etrn (Rin )u - (Rout )nonr- R ru Qu(11)where Rur is the radiant energy emitted as radiative losses by the charged particles, whichoriginated in V, regardless of where the radiative loss event occurs. Thus Etrn does not includeenergy going into radiative losses, where Etr does.The collision kerma, Kc is defined asKc dEntr(12)dmi.e. the collision kerma is the expectation value of the net energy transferred to chargedparticles per unit mass at the point of interest, excluding the radiative-loss energyFor monoenergetic photons,Kc en E en where en/ is the mass energy-absorption coefficient.Net Energy Transferrede Te' Te h h h h Etrn (R in ) u - (R out ) unonr -R ur Q6
h 1 - h 2 - (h 3 h 4 ) 0Absorbed DoseThe energy imparted by ionizing radiation to matter of mass m in a finite volume V is definedasE (Rin )u - (Rout )u (Rin )c - (Rout )c Q(13)where (Rin)u and (Rout)u are the radiant energy of all uncharged radiation entering and leavingV, (Rin)c and (Rout)c are the radiant energies of the charged particles entering and leaving V.The Absorbed dose, D, is the expectation value of the energy imparted to matter per unit massat a point.dE D(14)dmThus, the absorbed dose D is the expectation value of the energy imparted to matter per unitmass at a point.Energy ImpartedE (Rin )u - (Rout )u (Rin )c - (Rout )c Q h 1 - h 2 - (h 3 h 4 ) 0 Te 0'e Te' Te h h h h 7
ExposureExposure is symbolized as X and defined by the ICRU as the quotient of dQ by dm where thevalue of dQ is the absolute value of the total charge of the ions of one sign produced in (dry)air when all the electrons liberated by photons in air of mass dm are completely stopped in air,i.e.:dQX ( c/kg)(15)dmExposure is the ionization equivalent of the collision kerma in air for photons W e airAs an electron slows down in a gas, it loses energy by ionizing the gas. The quantity W is themean energy expended in the gas per ion pair formed, usually expressed in units of eV per ionpair. For air, this quantity is found to be a constant, independent of the electron energy above afew keV. The value isW 33.97 eV/ ion pairA more useful form of this quantity is in terms of the charge release. W 33 . 97 J / C e air(16)(W/e)air is the number of joules of energy deposited in the air per coulomb of charge released.Alternatively, (e/W)air is the number of coulombs released per joule of energy deposited in theair.Exposure and Air KermaThe definition of collision kerma states that it gives the energy transferred (less radiativelosses) to charge particles per unit mass. Multiplying (e/W)air by, the number of coulombs ofcharge created per joule of energy deposited will give the charge created per unit mass of air,i.e. the exposure: e X K c air W air en 8air C e W/ kg air (17)
e X cpe Dair W airThe relationship between air kerma and exposure is:( K c ) air ew X(18)or 1 g K air weX(19)where (Kc)air is the collisional part of air kerma, Kair is the total air kerma and g is the fractionof initial kinetic energy of electrons radiated as bremsstrahlung.The amount of energy lost to bremsstrahlung photons is not included in exposure becauseexposure is a measure of ionization finally produced by the secondary electrons. So the totalkerma includes the fraction g radiates as bremsstrahlung. In any event, f is a small value. For60Co, g 0.003; for 137Cs, g 0.002; and for orthovoltage g 0.0.The numerical relationship between air kerma and exposure for 60Co is:(1-0.003) Kair (J/kg) 33.97 (J/C) X (C/kg)0.997 Kair (Gy) 33.97 2.58 10-4 X (R)0.997 Kair (Gy) 8.76 10-3 X (R)Kair (Gy) 8.79 10-3 X (R)Similarly we may show that for 137Cs gamma rays we haveKair (Gy) 8.78 10-3 X (R)and for orthovoltage x-rays and lower energy x-rays we haveKair (Gy) 8.76 10-3 X (R)9
Measurement of ExposurePA schematic diagram of a free-air chamber.XD Q AD L(20)In a free-air chamber, ionization is collected from the volume between the dotted lines. Thiscollecting volume must be far enough removed from the diagram so that electronic equilibriumwill be established. This distance must be greater than the maximum range of secondaryelectrons created. The plate separation must be twice this distance. Under these conditionselectrons generate din the beam will expand all their energy before striking the collector.The definition of the Roentgen is in terms of mass of air. The use of the term air implies whatsome have termed ‘dry air’. Humidified air is a mixture of components of air and water vapor.Correction factors must be applied for measurement conditions other than those of thedefinition. At normal operating temperature and pressure the perfect gas law may be used. Ifthe mass of air in the definition is taken as a unit mass, then the number of units in a volume Vof ‘dry air’ is273 .2TPV760Because of difference in W values and electron densities between air and water vapor acorrection must be applied for humid air. In this case the number of unit masses of volume V is(21)273 . 2T P 0 . 238 P1 V760where, P1 is the vapor pressure of moisture in the air in mm of mercury.The collection volume is defined by the entrance aperture and the length of the collectingelectrode. If the cross sectional area of the x-ray beam is Ad at point P inside the chamber thenthe exposure at that point is10
Xwithd Q Ad L(22)Q collected charge density of airL length of collecting electrodeThe exposure at the end entrance diaphragm is2 S2 X DS 1 with S2 distance from source to point P inside chamber.S1 distance from source to entrance diaphragm DQ Ad L(23)Now the area of the entrance diaphragm is equal to Ad times an inverse square factor, i.e. S Ad AD 2 S1 2(24)with AD area of entrance diaphragm. ThenXD Q2 S AD 2 L S1 S2 S1 2(25)orXD Q AD L(26)This relation tells us that the exposure at the entrance diaphragm is equal to the chargecollected from the free-air chamber decided by the product of air density, the area of theentrance diaphragm, and the length of the collecting electrode.Cavity chambersThe basis of an air-wall chamber is that the air surrounding the active volume can be"condensed" into a "solid air" wall. A wall thick enough to provide electronic equilibriumpermits measurement of the Roentgen with cavity chambers. The definition of an air-wallchamber is a chamber whose walls interact with radiation in the same manner as air interacts.11
This condition means that the mass energy absorption and mass attenuation coefficients for thematerial should be the same as for air.To ensure that the walls are equilibrium thickness and to correct for wall attenuation,measurements must be made for a number of wall thicknesses. If the first wall thickness is lessthan equilibrium thickness then as the thickness increases the signal will increase until theequilibrium thickness is reached. The signal will decrease with increasing wall thicknessbecause of absorption of the primary photon beam in the wall. The exposure corrected for wallattenuation is found by extrapolating the attenuation curve back to zero wall thickness.The use of cavity chambers to measure exposure is based on the Bragg-Gray relation. Basicallythe Bragg-Gray theory relates the energy absorbed in a medium to energy absorbed in a cavityin that medium. Equation (27) below considers the medium to be material “w” and the cavityto contain a gas “g”. The energy a
The energy transferred in a volume, V is by definition (9) where (Rin)u is the radiant energy of uncharged particles entering V, (and radiant energy is the particles energy ignoring the rest mass); (Rout)u nonr is the radiation energy of uncharged particles leaving V, except that which originated fr