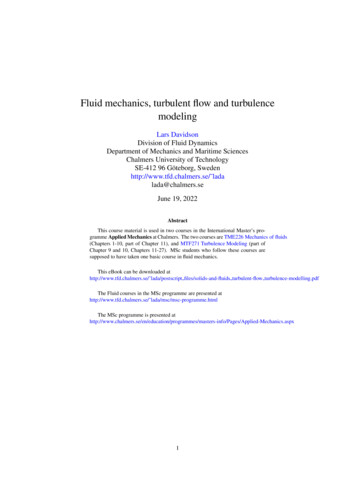
Transcription
Fluid mechanics, turbulent flow and turbulencemodelingLars DavidsonDivision of Fluid DynamicsDepartment of Mechanics and Maritime SciencesChalmers University of TechnologySE-412 96 Göteborg, Swedenhttp://www.tfd.chalmers.se/ ladalada@chalmers.seJune 19, 2022AbstractThis course material is used in two courses in the International Master’s programme Applied Mechanics at Chalmers. The two courses are TME226 Mechanics of fluids(Chapters 1-10, part of Chapter 11), and MTF271 Turbulence Modeling (part ofChapter 9 and 10, Chapters 11-27). MSc students who follow these courses aresupposed to have taken one basic course in fluid mechanics.This eBook can be downloaded athttp://www.tfd.chalmers.se/ lada/postscript files/solids-and-fluids turbulent-flow turbulence-modelling.pdfThe Fluid courses in the MSc programme are presented athttp://www.tfd.chalmers.se/ lada/msc/msc-programme.htmlThe MSc programme is presented asters-info/Pages/Applied-Mechanics.aspx1
Contents1234Motion, flow1.1Eulerian, Lagrangian, material derivative . . . . . . .dv2 v21.2What is the difference betweenand? . . . .dt t1.3Viscous stress, pressure . . . . . . . . . . . . . . . .1.4Strain rate tensor, vorticity . . . . . . . . . . . . . . .1.5Product of a symmetric and antisymmetric tensor . .1.6Deformation, rotation . . . . . . . . . . . . . . . . .1.7Irrotational and rotational flow . . . . . . . . . . . .1.7.1 Ideal vortex line . . . . . . . . . . . . . . . .1.7.2 Shear flow . . . . . . . . . . . . . . . . . . .1.8Eigenvalues and eigenvectors: physical interpretation. . . . . . . .1414.151517192021232525Governing flow equations2.1The Navier-Stokes equation . . . . . . . . . . . . . . . . . . . . .2.1.1 The continuity equation . . . . . . . . . . . . . . . . . . .2.1.2 The momentum equation . . . . . . . . . . . . . . . . . .2.2The energy equation . . . . . . . . . . . . . . . . . . . . . . . . .2.3Transformation of energy . . . . . . . . . . . . . . . . . . . . . .2.4Left side of the transport equations . . . . . . . . . . . . . . . . .2.5Material particle vs. control volume (Reynolds Transport Theorem).2727272728303132Solutions to the Navier-Stokes equation: three examples3.1The Rayleigh problem . . . . . . . . . . . . . . . . .3.2Flow between two plates . . . . . . . . . . . . . . . .3.2.1 Curved plates . . . . . . . . . . . . . . . . .3.2.2 Flat plates . . . . . . . . . . . . . . . . . . .3.2.3 Force balance, channel flow . . . . . . . . . .3.2.4 Balance equation for the kinetic energy . . . .3.3Two-dimensional boundary layer flow over flat plate .3.3.1 Momentum balance, boundary layer . . . . .333336363739404143.Vorticity equation and potential flow4.1Vorticity and rotation . . . . . . . . . . . . . . . . . . . . . . . . .4.2The vorticity transport equation in three dimensions . . . . . . . . .4.3The vorticity transport equation in two dimensions . . . . . . . . . .4.3.1 Boundary layer thickness from the Rayleigh problem . . . .4.4Potential flow . . . . . . . . . . . . . . . . . . . . . . . . . . . . .4.4.1 The Bernoulli equation . . . . . . . . . . . . . . . . . . . .4.4.2 Complex variables for potential solutions of plane flows . . .4.4.3 f z n . . . . . . . . . . . . . . . . . . . . . . . . . . . . .4.4.3.1 Parallel flow . . . . . . . . . . . . . . . . . . . . .4.4.3.2 Stagnation flow . . . . . . . . . . . . . . . . . . . .4.4.3.3 Flow over a wedge and flow in a concave corner . .4.4.4 Analytical solutions for a line source . . . . . . . . . . . . .4.4.5 Analytical solutions for a vortex line . . . . . . . . . . . . .4.4.6 Analytical solutions for flow around a cylinder . . . . . . . .4.4.7 Analytical solutions for flow around a cylinder with circulation4.4.7.1 The Magnus effect . . . . . . . . . . . . . . . . . .24747495253555556575858596061626568
34.4.85678The flow around an airfoil . . . . . . . . . . . . . . . . . . .Turbulence5.1Introduction . . . . . . . . . . . . . . .5.2Turbulent scales . . . . . . . . . . . . .5.3Energy spectrum . . . . . . . . . . . . .5.4The cascade process created by vorticity70.7272737478Turbulent mean flow6.1Time averaged Navier-Stokes . . . . . . . . . . .6.1.1 Boundary-layer approximation . . . . . .6.2Wall region in fully developed channel flow . . .6.3Reynolds stresses in fully developed channel flow6.4Boundary layer . . . . . . . . . . . . . . . . . . .828284848991.Probability density functions93Transport equations for turbulent kinetic energy8.1Rules for time averaging . . . . . . . . . . . . . . . . . . . . . . . .8.1.1 What is the difference between v1′ v2′ and v1′ v2′ ? . . . . . . .96969628.28.38.48.58.698.1.2 What is the difference between v1′2 and v1′ ? . . . . . . . . . 978.1.3 Show that v̄1 v1′2 v̄1 v1′2 . . . . . . . . . . . . . . . . . . . 978.1.4 Show that v̄ 1 v̄1 . . . . . . . . . . . . . . . . . . . . . . . 98The Exact k Equation . . . . . . . . . . . . . . . . . . . . . . . . . 988.2.1 Spectral transfer dissipation εκ vs. “true” viscous dissipation, ε 102The Exact k Equation: 2D Boundary Layers . . . . . . . . . . . . . 102Spatial vs. spectral energy transfer . . . . . . . . . . . . . . . . . . 104The overall effect of the transport terms . . . . . . . . . . . . . . . . 105The transport equation for v̄i v̄i /2 . . . . . . . . . . . . . . . . . . . 106Transport equations for Reynolds stresses9.1Source terms . . . . . . . . . . . . . . . . . . . . . . . . . . . . . .9.2Reynolds shear stress vs. the velocity gradient . . . . . . . . . . . .10811211310 Correlations10.1 Two-point correlations . . . . . . . . . . . . . . . . . . . . . . . . .10.2 Auto correlation . . . . . . . . . . . . . . . . . . . . . . . . . . . .10.3 Taylor’s hypothesis of frozen turbulence . . . . . . . . . . . . . . .11711711912011 Reynolds stress models and two-equation models11.1 Mean flow equations . . . . . . . . . . . . . .11.1.1 Flow equations . . . . . . . . . . . .11.1.2 Temperature equation . . . . . . . . .11.2 The exact vi′ vj′ equation . . . . . . . . . . . .11.3 The exact vi′ θ′ equation . . . . . . . . . . . .11.4 The k equation . . . . . . . . . . . . . . . . .11.5 The ε equation . . . . . . . . . . . . . . . . .11.6 The Boussinesq assumption . . . . . . . . . .11.7 Modeling assumptions . . . . . . . . . . . . .11.7.1 Production terms . . . . . . . . . . .122122122123123125127128129130130.
411.811.911.1011.1111.1211.1311.1411.7.2 Diffusion terms . . . . . . . . . . . . . . . . . . . . . . . .11.7.3 Dissipation term, εij . . . . . . . . . . . . . . . . . . . . .11.7.4 Slow pressure-strain term . . . . . . . . . . . . . . . . . . .11.7.5 Rapid pressure-strain term . . . . . . . . . . . . . . . . . .11.7.6 Wall model of the pressure-strain term . . . . . . . . . . . .The k ε model . . . . . . . . . . . . . . . . . . . . . . . . . . . .The modeled vi′ vj′ equation with IP model . . . . . . . . . . . . . .Algebraic Reynolds Stress Model (ASM) . . . . . . . . . . . . . . .Explicit ASM (EASM or EARSM) . . . . . . . . . . . . . . . . . .Derivation of the Explicit Algebraic Reynolds Stress Model (EARSM)11.12.1 The linear assumption of aij . . . . . . . . . . . . . . . . .Boundary layer flow . . . . . . . . . . . . . . . . . . . . . . . . . .Wall boundary conditions . . . . . . . . . . . . . . . . . . . . . . .11.14.1 Wall Functions . . . . . . . . . . . . . . . . . . . . . . . .11.14.2 Low-Re Number Turbulence Models . . . . . . . . . . . . .11.14.3 Low-Re k ε Models . . . . . . . . . . . . . . . . . . . . .11.14.4 Wall boundary Condition for k . . . . . . . . . . . . . . . .11.14.5 Different ways of prescribing ε at or near the wall . . . . . .12 Reynolds stress models vs. eddy-viscosity models12.1 Stable and unstable stratification . . . . . . .12.2 Curvature effects . . . . . . . . . . . . . . . .12.3 Stagnation flow . . . . . . . . . . . . . . . .12.4 RSM/ASM versus k ε models . . . . . . 59159.16116116216516613 Realizability13.1 Two-component limit . . . . . . . . . . . . . . . . . . . . . . . . .16716814 Non-linear Eddy-viscosity Models17015 The V2F Model15.1 Modified V2F model . . . . . . . . . . . . . . . . . . . . . . . . . .15.2 Realizable V2F model . . . . . . . . . . . . . . . . . . . . . . . . .15.3 To ensure that v 2 2k/3 . . . . . . . . . . . . . . . . . . . . . . .17317617717716 The SST Model17817 Overview of RANS models18318 Large Eddy Simulations18.1 Time averaging and filtering . . . . . . . . . . . . . . . . . . . . . .18.2 Differences between time-averaging (RANS) and space filtering (LES)18.3 Resolved & SGS scales . . . . . . . . . . . . . . . . . . . . . . . .18.4 The box-filter and the cut-off filter . . . . . . . . . . . . . . . . . .18.5 Highest resolved wavenumbers . . . . . . . . . . . . . . . . . . . .18.6 Subgrid model . . . . . . . . . . . . . . . . . . . . . . . . . . . . .18.7 Smagorinsky model vs. mixing-length model . . . . . . . . . . . . .18.8 Energy path . . . . . . . . . . . . . . . . . . . . . . . . . . . . . .18.9 SGS kinetic energy . . . . . . . . . . . . . . . . . . . . . . . . . .18.10 LES vs. RANS . . . . . . . . . . . . . . . . . . . . . . . . . . . . .18.11 The dynamic model . . . . . . . . . . . . . . . . . . . . . . . . . .184184185186187188189190190191192192.
518.12 The test filter . . . . . . . . . . . . . . . . . . . . .18.12.1 2D filtering . . . . . . . . . . . . . . . . .18.12.2 3D filtering . . . . . . . . . . . . . . . . .18.13 Stresses on grid, test and intermediate level . . . . .18.14 Numerical dissipation . . . . . . . . . . . . . . . .18.15 Scale-similarity Models . . . . . . . . . . . . . . .18.16 The Bardina Model . . . . . . . . . . . . . . . . .18.17 Redefined terms in the Bardina Model . . . . . . .18.18 A dissipative scale-similarity model. . . . . . . . .18.19 Forcing . . . . . . . . . . . . . . . . . . . . . . . .18.20 Numerical method . . . . . . . . . . . . . . . . . .18.20.1 RANS vs. LES . . . . . . . . . . . . . . .18.21 One-equation ksgs model . . . . . . . . . . . . . .18.22 Smagorinsky model derived from the ksgs equation18.23 A dynamic one-equation model . . . . . . . . . . .18.24 A Mixed Model Based on a One-Eq. Model . . . .18.25 Applied LES . . . . . . . . . . . . . . . . . . . . .18.26 Resolution requirements . . . . . . . . . . . . . . 0520619 URANS: Unsteady RANS19.1 Turbulence Modeling . . . . . . . . . . . . . . . . . . . . . . . . .19.2 Discretization . . . . . . . . . . . . . . . . . . . . . . . . . . . . .20820921020 DES: Detached-Eddy-Simulations20.1 DES based on two-equation models . . . . . . . . . . . . . . . . . .20.2 DES based on the k ω SST model . . . . . . . . . . . . . . . . .20.3 DDES . . . . . . . . . . . . . . . . . . . . . . . . . . . . . . . . .21321321421621 Hybrid LES-RANS21.1 Momentum equations in hybrid LES-RANS . . . . . . . . . . . . .21.2 The one-equation hybrid LES-RANS model . . . . . . . . . . . . .21721921922 The SAS model22.1 Resolved motions in unsteady . . . . . . . . . . . . . . .22.2 The von Kármán length scale . . . . . . . . . . . . . . . .22.3 The SST-SAS model . . . . . . . . . . . . . . . . . . . . .22.4 The second derivative of the velocity . . . . . . . . . . . .22.5 Evaluation of the von Kármán length scale in channel flow.22022022122222322323 The PANS Model23.1 PANS as a hybrid LES-RANS model . . . . . . . . . . . . .23.1.1 The interface conditions at the RANS-LES interface .23.2 Zonal PANS: different treatments of the RANS-LES interface23.2.1 The Interface Condition . . . . . . . . . . . . . . . .23.2.2 Modeling the Interface . . . . . . . . . . . . . . . .23.2.2.1 Interface Model 1 . . . . . . . . . . . . . .23.2.2.2 Interface Model 2 . . . . . . . . . . . . . .23.2.2.3 Interface Model 3 . . . . . . . . . . . . . .23.2.2.4 Interface Model 4 . . . . . . . . . . . . . .23.3 A new formulation of fk for the PANS model . . . . . . . .226229229230231233233233234234235
623.3.1 fk derived from the equivalence criterion . . .The IDD-PANS model . . . . . . . . . . . . . . . . .23.4.1 LES length scale . . . . . . . . . . . . . . .23.4.2 The IDDES model . . . . . . . . . . . . . . .23.4.3 Equivalence between PANS and DES/IDDES23.4.3.1 PANS equations . . . . . . . . . . .23.4.3.2 IDDES equations . . . . . . . . . .23.4.3.3 PITM equations . . . . . . . . . . .23.4.4 Deriving an expression for fk for PANS . . .23.4.5 Deriving an expression for fk for PITM . . .23.4.6 Turbulence with convection . . . . . . . . . .23.4.6.1 PANS/PITM equations . . . . . . . .23.4.6.2 DES equations . . . . . . . . . . . .23523723723723824024024124124224224224324 The PITM model24.1 RANS mode . . . . . . . . . . . . . . . . . . . . . . . . . . . . . .24.2 LES mode . . . . . . . . . . . . . . . . . . . . . . . . . . . . . . .24524524525 Hybrid LES/RANS for Dummies25.1 Introduction . . . . . . . . . . . . . . . . . . . . . . . . . .25.1.1 Reynolds-Averaging Navier-Stokes equations: RANS25.1.2 Large Eddy Simulations: LES . . . . . . . . . . . .25.1.3 Zonal LES/RANS . . . . . . . . . . . . . . . . . . .25.2 The PANS k ε turbulence model . . . . . . . . . . . . . .25.3 Zonal LES/RANS: wall modeling . . . . . . . . . . . . . . .25.3.1 The interface conditions . . . . . . . . . . . . . . . .25.3.2 Results . . . . . . . . . . . . . . . . . . . . . . . . .25.4 Zonal LES/RANS: embedded LES . . . . . . . . . . . . . .25.4.1 The interface conditions . . . . . . . . . . . . . . . .25.4.2 Results . . . . . . . . . . . . . . . . . . . . . . . . .24924924924925025025125125225225225423.4.26 Commutation terms in the k and ω equations27 Inlet boundary conditions27.1 Synthesized turbulence . . . . . . . . . . . . . . . . . . . . . .27.2 Random angles . . . . . . . . . . . . . . . . . . . . . . . . . . .27.3 Highest wave number . . . . . . . . . . . . . . . . . . . . . . .27.4 Smallest wave number . . . . . . . . . . . . . . . . . . . . . . .27.5 Divide the wave number range . . . . . . . . . . . . . . . . . .27.6 von Kármán spectrum . . . . . . . . . . . . . . . . . . . . . . .27.7 Computing the fluctuations . . . . . . . . . . . . . . . . . . . .27.8 Introducing time correlation . . . . . . . . . . . . . . . . . . . .27.9 Anisotropic Synthetic Turbulent Fluctuations . . . . . . . . . . .27.9.1 Eigenvalues and eigenvectors . . . . . . . . . . . . . . .27.9.2 Synthetic fluctuations in the principal coordinate system .255.25925925926026026026126126126526626728 Overview of LES, hybrid LES-RANS and URANS models26829 Best practice guidelines (BPG)29.1 EU projects . . . . . . . . . . . . . . . . . . . . . . . . . . . . . .271271
729.229.329.4ABCDErcoftac workshops . . . . . . . . . . . . . . . . . . . . . . . . . .Ercoftac Classical Database . . . . . . . . . . . . . . . . . . . . . .ERCOFTAC QNET Knowledge Base Wiki . . . . . . . . . . . . . .TME226 Lecture NotesA.1 Lecture 1 . . . . . .A.2 Lecture 2 . . . . . .A.3 Lecture 3 . . . . . .A.4 Lecture 4 . . . . . .A.5 Lecture 5 . . . . . .A.6 Lecture 6 . . . . . .A.7 Lecture 7 . . . . . .A.8 Lecture 8 . . . . . .A.9 Lecture 9 . . . . . ion to tensor notationB.1 What is a tensor? . . . . . . . . . . . . . . . . . . . . . .B.2 Examples of equations using tensor notation . . . . . . .B.2.1 Newton’s second law . . . . . . . . . . . . . . .B.2.2 Divergence · v 0 . . . . . . . . . . . . . .B.2.3 The left-hand side of Navier-Stokes vℓ vm / xℓB.3 Contraction . . . . . . . . . . . . . . . . . . . . . . . .B.4 Two Tensor Rules . . . . . . . . . . . . . . . . . . . . .B.4.1 The summation rule . . . . . . . . . . . . . . .B.4.2 Free Index . . . . . . . . . . . . . . . . . . . .B.5 Special Tensors . . . . . . . . . . . . . . . . . . . . . .B.5.1 Kroenecker’s δ (identity tensor) . . . . . . . . .B.5.2 Levi-Civita’s εijk (permutation tensor) . . . . .B.6 Symmetric and antisymmetric tensors . . . . . . . . . . .B.7 Vector Product . . . . . . . . . . . . . . . . . . . . . . .B.8 Derivative Operations . . . . . . . . . . . . . . . . . . .B.8.1 The derivative of a vector B: . . . . . . . . . . .B.8.2 The gradient of a scalar a: . . . . . . . . . . . .B.8.3 The divergence of a vector B: . . . . . . . . . .B.8.4 The curl of a vector B: . . . . . . . . . . . . . .B.8.5 The Laplace operator on a scalar a: . . . . . . .B.9 Integral Formulas . . . . . . . . . . . . . . . . . . . . .B.10 Multiplication of tensors . . . . . . . . . . . . . . . . . 35335336336336336336.TME226: ǫ δ identityTME226 Assignment 1 in 2020: laminar flow in a channelD.1 Fully developed region . . . . . . . . . . . . . . . . .D.2 Wall shear stress . . . . . . . . . . . . . . . . . . . . .D.3 Inlet region . . . . . . . . . . . . . . . . . . . . . . . .D.4 Wall-normal velocity in the developing region . . . . .D.5 Vorticity . . . . . . . . . . . . . . . . . . . . . . . . .D.6 Deformation . . . . . . . . . . . . . . . . . . . . . . .D.7 Dissipation . . . . . . . . . . . . . . . . . . . . . . . .D.8 Eigenvalues . . . . . . . . . . . . . . . . . . . . . . .338.339340340341341341341341342
8D.9EFGHEigenvectors . . . . . . . . . . . . . . . . . . . . . . . . . . . . . .342TME226 Assignment 1 in 2021: laminar flow in a boundary layerE.1 Velocity profiles . . . . . . . . . . . . . . . . . . . . . . . . .E.2 Boundary layer thickness . . . . . . . . . . . . . . . . . . . .E.3 Velocity gradients . . . . . . . . . . . . . . . . . . . . . . . .E.4 Skinfriction . . . . . . . . . . . . . . . . . . . . . . . . . . .E.5 Vorticity . . . . . . . . . . . . . . . . . . . . . . . . . . . . .E.6 Deformation . . . . . . . . . . . . . . . . . . . . . . . . . . .E.7 Dissipation . . . . . . . . . . . . . . . . . . . . . . . . . . . .E.8 Eigenvalues . . . . . . . . . . . . . . . . . . . . . . . . . . .E.9 Eigenvectors . . . . . . . . . . . . . . . . . . . . . . . . . . .E.10 Terms in the v1 equation . . . . . . . . . . . . . . . . . . . . .343344345345346346346346347347347TME226, Assignment 2: Turbulent flow using STAR-CCM F.1Backward Facing Step Tutorial . . . . . . . . . . . . . .F.22D Hill Flow . . . . . . . . . . . . . . . . . . . . . . . .F.3Steady Flow: 2D Hill Flow Tutorial . . . . . . . . . . . .F.3.1 Pressure . . . . . . . . . . . . . . . . . . . . . .F.3.2 Skinfriction . . . . . . . . . . . . . . . . . . . .F.3.3 Vorticity . . . . . . . . . . . . . . . . . . . . . .F.3.4 Turbulent viscosity . . . . . . . . . . . . . . . .F.3.5 Diffusion . . . . . . . . . . . . . . . . . . . . .F.3.6 Production . . . . . . . . . . . . . . . . . . . . .F.3.7 Wall boundary conditions for ε . . . . . . . . . .F.3.8 Near-wall behaviour of fµ . . . . . . . . . . . .F.3.9 Compare with experiments . . . . . . . . . . . .349349349350356356357357358358358358359TME226 Assignment 2: turbulent flow (2017)G.1 Time history . . . . . . . . . . . . . . . .G.2 Time averaging . . . . . . . . . . . . . .G.3 Mean flow . . . . . . . . . . . . . . . . .G.4 The time-averaged momentum equation .G.5 The gradient . . . . . . . . . . . . . . . .G.6 Wall shear stress . . . . . . . . . . . . . .G.7 Resolved stresses . . . . . . . . . . . . .G.8 Fluctuating wall shear stress . . . . . . . .G.9 Production terms . . . . . . . . . . . . . .G.10 Pressure-strain terms . . . . . . . . . . . .G.11 Dissipation . . . . . . . . . . . . . . . . .G.12 Do something fun! . . . . . . . . . . . . .360360360361361362363363363363364364365TME226: Fourier seriesH.1 Orthogonal functions . . .H.2 Trigonometric functions . .H.2.1 “Length” of ψk . .H.2.2 Orthogonality of φnH.2.3 Orthogonality of φnH.2.4 “Length” of φk . .H.3 Fourier series of a function.366366367368368368368369. . . . . . . . . .and ψkand φk. . . . . . .
9H.4H.5IJKDerivation of Parseval’s formula . . . . . . . . . . . . . . . . . . .Complex Fourier series . . . . . . . . . . . . . . . . . . . . . . . .369371TME226: Why does the energy spectrum, E, have such strange dimensions?372I.1Energy spectrum for an ideal vortex . . . . . . . . . . . . . . . . . . 372I.2An example . . . . . . . . . . . . . . . . . . . . . . . . . . . . . . 373I.3An example: Matlab code . . . . . . . . . . . . . . . . . . . . . . . 374TME226 Learning outcomes 2020MTF271 Lecture NotesK.1 Lecture 1 . . . . .K.2 Lecture 2 . . . . .K.3 Lecture 3 . . . . .K.4 Lecture 4 . . . . .K.5 Lecture 5 . . . . .K.6 Lecture 6 . . . . .K.7 Lecture 7 . . . . .K.8 Lecture 8 . . . . .K.9 Lecture 9 . . . . .K.10 Lecture 10 . . . .K.11 Lecture 11 . . . .K.12 Lecture 12 . . . 1: Some properties of the pressure-strain term449MMTF271: Galilean invariance450NMTF271: Computation of wavenumber vector and anglesN.1 The wavenumber vector, κnj . . . . . . . . . . . . . . . . . . . . . .N.2 Unit vector σin . . . . . . . . . . . . . . . . . . . . . . . . . . . . .452452453OMTF271: 1D and 3D energy spectraO.1 Energy spectra from two-point correlations . . . . . . . . . . . . . .454455PMTF271. Derivations of the IDD-PANS modelP.1DES model . . . . . . . . . . . . . . . . . . . . . . . . . . . . . . .P.2PANS model . . . . . . . . . . . . . . . . . . . . . . . . . . . . . .P.3PITM model . . . . . . . . . . . . . . . . . . . . . . . . . . . . . .457457458459QMTF271, Assignment 1: Reynolds averaged Navier-StokesQ.1 Part I: Data of Two-dimensional flow . . . . . . . . . .Q.1.1 Analysis . . . . . . . . . . . . . . . . . . . . .Q.1.2 The momentum equations . . . . . . . . . . . .Q.1.3 The turbulent kinetic energy equation . . . . .Q.1.4 The Reynolds stress equations . . . . . . . . .Q.2 How to compute derivatives on a curvi-linear mesh . . .Q.2.1 Geometrical quantities . . . . . . . . . . . . .Q.3 Part II: StarCCM . . . . . . . . . . . . . . . . . . . .Q.3.1 Backward Facing Step Tutorial . . . . . . . . .Q.3.2 Asymmetric diffuser case . . . . . . . . . . . .461461461462463464465467467467468.
10Q.3.3 Brief instruction to begin the asymmetric diffuser case . . .RMTF271, Assignment: LES, Reτ 1 000R.1 Time history . . . . . . . . . . . . . . .R.2 Time averaging . . . . . . . . . . . . .R.3 Auto correlation . . . . . . . . . . . . .R.4 Probability density/histogram . . . . . .R.5 Frequency spectrum . . . . . . . . . . .R.6 Backstep . . . . . . . . . . . . . . . . .R.7 Channel flow: resolved stresses . . . . .R.8 Resolved production and pressure-strainR.9 Filtering . . . . . . . . . . . . . . . . .R.10 SGS stresses: Smagorinsky model . . .R.11 SGS stresses: WALE model . . . . . . .R.12 Dissipations . . . . . . . . . . . . . . .R.13 Test filtering . . . . . . . . . . . . . . .R.14 Near-wall behavior . . . . . . . . . . . .R.15 Two-point correlations . . . . . . . . . .R.16 Energy spectra, optional . . . . . . . . .R.17 Something fun . . . . . . . . . . . . . 485486486SMTF271: Compute energy spectra from LES/DNS data using PythonS.1 Introduction . . . . . . . . . . . . . . . . . . . . . . . . . . . . . .S.2 An example of using FFT . . . . . . . . . . . . . . . . . . . . . . .S.3 Energy spectrum from the two-point correlation . . . . . . . . . . .S.4 Energy spectra from the autocorrelation . . . . . . . . . . . . . . . .487487487490492TMTF271, Assignment 2a: DES, DDES and SAST.1 Time history . . . . . . . . . . . . . . . . .T.2 Mean velocity profile . . . . . . . . . . . .T.3 Resolved stresses . . . . . . . . . . . . . .T.4 Turbulent kinetic energy . . . . . . . . . . .T.5 The modelled turbulent shear stress . . . . .T.6 Location of interface in DES and DDES . .T.7 SAS turbulent length scales . . . . . . . . .T.8 Anisotropic errors (optional) . . . . . . . .493493495495496496496497497.UMTF271, Assignment 2b, recirculating flow:U.1 Discretization schemes . . . . . . . . .U.2 The modeled turbulent shear stress . . .U.3 The turbulent viscosity . . . . . . . . .U.4 Location of interface . . . . . . . . . . .U.5 Location of interface in DES and DDESU.6 The SAS model . . . . . . . . . . . . .U.7 Different ways to evaluate resolution . .U.7.1 Anisotropic errors (optional) . .U.7.2 Two-point correlations . . . . .PANS, DES, DDES and SAS499. . . . . . . . . . . . . . . 500. . . . . . . . . . . . . . . 501. . . . . . . . . . . . . . . 501. . . . . . . . . . . . . . . 502. . . . . . . . . . . . . . . 503. . . . . . . . . . . . . . . 504. . . . . . . . . . . . . . . 504. . . . . . . . . . . . . . . 505. . . . . . . . . . . . . . . 506VMTF271, Assignment: Embedded LES with PANS, channel flowV.1 Time history . . . . . . . . . . . . . . . . . . . . . . . . . . . . . .508508
11V.2V.3V.4V.5.509509510510W MTF271, Assignment 3: Hybrid LES-RANS, channel flowW.1 Time history . . . . . . . . . . . . . . . . . . . . . . .W.2 Mean velocity profile . . . . . . . . . . . . . . . . . .W.3 Resolved stresses . . . . . . . . . . . . . . . . . . . .W.4 Turbulent kinetic energy . . . . . . . . . . . . . . . . .W.5 The modelled turbulent shear stress . . . . . . . . . . .W.6 Location of interface in DES and DDES . . . . . . . .W.7 Turbulent length scales . . . . . . . . . . . . . . . . 518518520521521XResolved stresses . . . .Turbulent viscosity . . . .Modeled stresses . . . . .Turbulent SGS dissipation.MTF271, Assignment 4: Synthetic inlet fluctuationsX.1 synt main . . . . . . . . . . . . . . . . . . . .X.2 angles . . . . . . . . . . . . . . . . . . . . . .X.3 rand1.m . . . . . . . . . . . . . . . . . . . . .X.4 rand2.m . . . . . . . . . . . . . . . . . . . . .X.5 Mean values; randomness . . . . . . . . . . . . .X.6 RMS and shear stress . . . . . . . . . . . . . . .X.7 Two-point correlations . . . . . . . . . . . . . . .X.8 The integral length scale . . . . . . . . . . . . . .X.9 Correlation in time . . . . . . . . . . . . . . . . .X.10 Autocorrelation in time . . . . . . . . . . . . . .X.11 Spectrum . . . . . . . . . . . . . . . . . . . . . .X.12 Anisotropic fluctuations . . . . . . . . . . . . . .YMTF271, Assignment 5: synthetic anisotropic fluctuations using EARSM524Y.1 Mean flow . . . . . . . . . . . . . . . . . . . . . . . . . . . . . . . 524Y.2 Synthetic fluctuations . . . . . . . . . . . . . . . . . . . . . . . . . 524Y.2.1 Chapter 1 . . . . . . . . . . . . . . . . . . . . . . . . . . . 525Y.2.2 Chapter 2-3 . . . . . . . . . . . . . . . . . . . . . . . . . . 525Y.3 Exercises . . . . . . . . . . . . . . . . . . . . . . . . . . . . . . . . 525ZMTF271: Transformation of tensorsZ.1 Rotation from x1 x2 to x1 x2 . . . . . . . . . . . . . . . . .Z.2 Rotation from x1 x2 to x1 x2 . . . . . . . . . . . . . . . . .Z.3 Transformation of a velocity gradient . . . . . . . . . . . . . . . . .AA MTF271: Green’s formulasAA.1 Green’s first formula . . . . . . . . . . .AA.2 Green’s second formula . . . . . . . . .AA.3 Green’s third formula . . . . . . . . . .AA.4 Analytical solution to Poisson’s equation.526526527528529529529529532AB MTF271: Learning outcomes for 2022533AC MTF271: Discussion seminarsAC.1 Seminar 1 . . . . . . . . . . . . . . . . . . . . . . . . . . . . . . .AC.1.1 Basic topics . . . . . . . . . . . . . . . . . . . . . . . . . .542542542
12AC.1.2 Advanced topicsAC.2 Seminar 2 . . . . . . .AC.2.1 Basic topics . .AC.2.2 Advanced topicsAC.3 Seminar 3 . . . . . . .AC.3.1 B
Fluid mechanics, turbulent flow and turbulence modeling Lars Davidson Divisionof Fluid Dynamics Department of Mechanics and Maritime Sciences Chalmers University of Technology