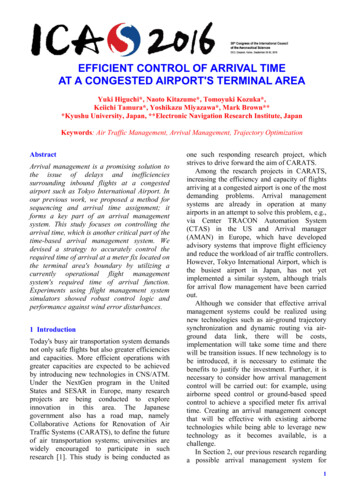
Transcription
EFFICIENT CONTROL OF ARRIVAL TIMEAT A CONGESTED AIRPORT'S TERMINAL AREAYuki Higuchi*, Naoto Kitazume*, Tomoyuki Kozuka*,Keiichi Tamura*, Yoshikazu Miyazawa*, Mark Brown***Kyushu University, Japan, **Electronic Navigation Research Institute, JapanKeywords: Air Traffic Management, Arrival Management, Trajectory OptimizationAbstractArrival management is a promising solution tothe issue of delays and inefficienciessurrounding inbound flights at a congestedairport such as Tokyo International Airport. Inour previous work, we proposed a method forsequencing and arrival time assignment; itforms a key part of an arrival managementsystem. This study focuses on controlling thearrival time, which is another critical part of thetime-based arrival management system. Wedevised a strategy to accurately control therequired time of arrival at a meter fix located onthe terminal area's boundary by utilizing acurrently operational flight managementsystem's required time of arrival function.Experiments using flight management systemsimulators showed robust control logic andperformance against wind error disturbances.1 IntroductionToday's busy air transportation system demandsnot only safe flights but also greater efficienciesand capacities. More efficient operations withgreater capacities are expected to be achievedby introducing new technologies in CNS/ATM.Under the NextGen program in the UnitedStates and SESAR in Europe, many researchprojects are being conducted to exploreinnovation in this area. The Japanesegovernment also has a road map, namelyCollaborative Actions for Renovation of AirTraffic Systems (CARATS), to define the futureof air transportation systems; universities arewidely encouraged to participate in suchresearch [1]. This study is being conducted asone such responding research project, whichstrives to drive forward the aim of CARATS.Among the research projects in CARATS,increasing the efficiency and capacity of flightsarriving at a congested airport is one of the mostdemanding problems. Arrival managementsystems are already in operation at manyairports in an attempt to solve this problem, e.g.,via Center TRACON Automation System(CTAS) in the US and Arrival manager(AMAN) in Europe, which have developedadvisory systems that improve flight efficiencyand reduce the workload of air traffic controllers.However, Tokyo International Airport, which isthe busiest airport in Japan, has not yetimplemented a similar system, although trialsfor arrival flow management have been carriedout.Although we consider that effective arrivalmanagement systems could be realized usingnew technologies such as air-ground trajectorysynchronization and dynamic routing via airground data link, there will be costs,implementation will take some time and therewill be transition issues. If new technology is tobe introduced, it is necessary to estimate thebenefits to justify the investment. Further, it isnecessary to consider how arrival managementcontrol will be carried out: for example, usingairborne speed control or ground-based speedcontrol to achieve a specified meter fix arrivaltime. Creating an arrival management conceptthat will be effective with existing airbornetechnologies while being able to leverage newtechnology as it becomes available, is achallenge.In Section 2, our previous research regardinga possible arrival management system for1
Y. HIGUCHI et al.Tokyo International Airport is introduced,which has been published in references [2 4].It consists of a potential benefit analysis andoptimal arrival time assignment, both of whichshowed promising results. The next key issue isthe control of the arrival time at a meter fix onthe boundary of a terminal area. In Section 3,we therefore discuss the required time of arrival(RTA) function of a flight management system(FMS) to realize the assigned arrival time viaonboard equipment. A flight managementworkstation (FMW) operated by the ENRI isused for this research. Auto-flight commandsgenerated by the FMS are examined bycomparing off-line trajectory optimizations. Thesimulation aims to act against wind errordisturbances and has shown promising resultsand provides useful information for futureground control strategy development.2. Previous work2.1 Efficiency analysis of inbound flights toTokyo International AirportAs congested airports generally suffer fromflight delays and resulting flight inefficiencies,it is useful to conduct a quantitative objectiveanalysis of the potential benefits (i.e., acomparison between the current state and anideal but still achievable state) since it willenable future plans for improvements to bedrawn up. We conducted a potential benefitanalysis for inbound flights to TokyoInternational Airport, in which surveillance datafrom an SSR Mode S, experimentally operatedby the ENRI, Tokyo, were used and in whichthe initial points depended on radar coverage,i.e., they were located within approximately 200NM of the airport.For the analysis, we developed a flightparameter estimation approach (including a fuelconsumption estimation) from surveillanceradar positioning data using the JapanMeteorological Agency's numerical weatherprediction Grid Point Value (GPV) data [5] toestimate the air data parameters, such as true airspeed, calibrated airspeed, and Mach number.Additionally, Base of Aircraft Data (BADA,Rev. 3.11), developed by EUROCONTROL,were used to estimate performance data, such asdrag and fuel flow [6]. Furthermore, wecalculated the optimal trajectory for each flightwith dynamic programming (DP) by inputtingthe initial and terminal condition of the actualflightandusingthecorrespondingmeteorological condition's GPV and the BADAperformance model. This revealed that asignificant amount of flight time and fuel arewasted during the descent phase; therefore,arrival management for inbound flights isdefinitely necessary [2].2.2 Optimal sequencing and arrival timeassignmentOne of the key issues of an arrival managementsystem is the fair assignment and accuratecontrol of the arrival time at a meter fix on theboundary of the terminal area, which is essentialto achieve safe separations between the aircraftFig. 1 Horizontal plane trajectories of inbound flights landing on RWY34L (left) and the distance tothe approach fix ARLON as a function of flight time (right) between 19:30 and 23:00, May 9th, 2012.2
EFFICIENT CONTROL OF ARRIVAL TIME ATA CONGESTED AIRPORT'S TERMINAL AREAFig. 2 Arrival time separation at ARLON from 7:00 to 23:00 on May 9th, 2012 (left: actual; center:optimal trajectory without constraint; and right: optimal trajectory with 90 s time separation constraint).Fig. 3 Difference of arrival times of optimal trajectories with and without time separation constraint,STA–ETA (STA: scheduled time of arrival; ETA: estimated time of arrival), May 9th, 2012.Table 1 Average fuel consumption and flight time for 375 flights.Actual flightFuel burn (kg)Flight time (s)42173741Optimal trajectories (difference, ratio relative to actual flight)(a) Without time constraint(b) With time constraint3658( 559, 13.24%)3691( 526, 12.47%)3592( 149, 4.00%)3594( 147, 3.93%)on the predetermined route to the runway.Arrival sequencing and arrival time assignmentby the ground system should consider the windconditions and each aircraft's performance. Inour previous work, we proposed a concept forsequencing and arrival time assignment thatadequately provided efficient flight paths for allarrival flights. [3][4] The total performanceindices, consisting of the performance indices ofeach flight, were optimized so that they wouldequally share the effects caused by theconstraints of the time separation at the meterfix. The concept was examined by applying tothe arrival flights to Tokyo International Airportusing surveillance data from the entirety ofJapanese airspace (CARATS Open Data [7]).Figs. 1 3 are examples of the results of allflights that landed on RW34L during one day.Fig. 1 shows that sequencing and time(b)–(a) 33 2separation are currently accomplished byvectoring to add delays in most cases. Fig. 2shows the time separation at an approach fixARLON for three cases: actual, optimal withouttime separation constraint, and optimal withtime separation constraint. Table 1 shows thatthe differences in fuel savings and flight timesbetween those obtained with and without thearrival time constraint were fairly minimal. Inparticular, the average flight time was almostthe same, which means that the arrival timeadjustment was not only achieved with timedelays but also time advances. Fig. 3 shows theeach flight's arrival time adjustment (STA-ETA)necessary for the time separation constraint, i.e.,the positive value is time delay and the negativevalue is time advance.3. Arrival time control3
Y. HIGUCHI et al.3.1 FMS with all-phase RTAArrival time assignment based on our potentialbenefit analysis has shown that significantimprovements in air traffic efficiency arepossible. Arrival time control should beperformed by utilizing speed instead of beingbased on detours (i.e., vectoring) to achieve anideal performance. Currently, an onboard FMSoptimizes its own flight profile usingperformance-related parameters such as take-offweight and meteorological information. MostFMSs have an RTA function. Some of the RTAfunctions, however, are limited to the cruisingphase only, while others, referred to as all-phaseRTA functions, can control the arrival time at ameter fix on the boundary of the terminal area.Approximately half of all currently operationalaircraft in Japan are equipped with all-phaseRTA functions, but the proportion is increasing.As the control strategy of an operationalFMS can be a reference for ground control forfuture possible developments, all-phase RTAfunctions were evaluated. The ENRI's GEAviation FMW for a single-aisle jet passengeraircraft (Fig. 4), which can simulate an FMS-inthe-loop flight, was used for that purpose. Thesimulation scenario conducted was fromFukuoka Airport to Tokyo International Airport,as shown in Fig. 5. The analyzed extent wasfrom 15,000 ft during the climb phase to themeter fix ADDUM at 10,000 ft. The flight planfollowed standard air routes. The flight distancewas about 500 NM.An FMS essentially optimizes fuel burn andflight time, where the trade-off parameter iscalled the cost index (CI), which is the ratio ofthe flight time value against the fuel value. Thearrival time is controlled by changing the CI toensure that the estimated time of arrival (ETA)is equal to the RTA. Fig. 6 plots assignableRTAs, which are limited by the earliest arrivaland the latest arrival time relative to the arrivaltime obtained by the simulation with CI 25.Plots with different CIs (between 0 and 100)show the time difference with relation to thetrajectory of CI 25. They show which CIvalue should be chosen at each position tochange the arrival time. The flight time delay ofthe latest arrival is more than that for CI 0,which means that a negative CI is used in theFMS, although pilots cannot input negative CIvalues.Our trajectory optimization by DP essentiallyconducts the same optimization, except that ituses the BADA performance model, whichutilizes different performance data than thoseimplemented in the FMS. The relation betweenthe CI and the performance parameters, i.e., thefuel burn and the flight time, was evaluated bycomparing the simulation results with optimaltrajectories calculated via the DP and BADAperformance models. Fig. 7 shows the results.As the direct output of fuel burn from thesimulation is not accurate, it was calculatedusing both flight parameters and the BADAmodel. A flight parameter for the DP calculationwas modified to follow the limitations of FMSs,i.e., that the minimum speed is limited to 230 kt,which is the given terminal speed at the meterfix. A simple three-parameter-model optimaltrajectory (3 Param. Opt.) in which only threeparameters, i.e., climb CAS, cruise Mach, anddescent CAS are optimized is also considered tosimulate the FMS outputs. Fig. 8 plots the timehistories of the FMS's simulator output and theoptimal trajectories.Fig. 4 Flight Management Workstation.Fig. 5 Horizontal plane trajectory of the flightplan.4
EFFICIENT CONTROL OF ARRIVAL TIME ATA CONGESTED AIRPORT'S TERMINAL AREAFig. 6 Assignable RTA depending on distance tothe target waypoint (CI 25).Fig. 7 Fuel burn and flight time trade-off ofFMS simulator and trajectory optimizations.Fig. 8 Fuel burn and flight time, simulation results and off-line trajectory optimization.5
Y. HIGUCHI et al.Although the accuracy of the arrival time iscritical for time separation at the meter fix, itcan deteriorate owing to wind prediction errors;therefore, an evaluation of the RTA function'sperformance is an important issue in actuallyimplementing an FMS RTA function in anarrival management system [8]–[10]. Arrivaltime errors owing to wind prediction errors wereevaluated by using the same flight scenarios.Fig. 9 shows the wind error profile. The errorvaried between 30% and 30% in 10% steps.This means that the error level was much largerthan in our weather forecast data analysis usingquick-access recorder (QAR) data or SSR modeS downlink aircraft parameter data [11][12]. Asthe table shows, however, the arrival time errorswere less than 12 s.Next, a step input of the wind error wasconsidered to identify the feedback controlstrategy. As explained in Ref. [13], FMSsrecalculate the flight profile when the differencebetween the RTA and ETA exceeds a thresholdthat depends on the time-to-go, i.e., thedifference between the RTA and the presenttime.Table 3 shows six cases of the wind error andthe RTA performance of each simulation. Figs.11–16 show the time histories of each case.Except for Case 1-2, the RTA error was lessthan 10 s. As shown in Fig. 6, the early arrivalcapability was less than the late arrivalcapability, arrival time control against stronghead-wind bias errors (like for Case 1-2) wasmore limited than that against tail-wind biaserrors. The threshold for recalculation illustratedin Fig. 17 was confirmed by the simulation.Table 2 Results of arrival time error at the targetwaypoint ADDUM (ATA, actual time of arrival).Wind errorFig. 9 Simulated wind conditions.(a) AltitudeNo error 10%–10% 20%-20% 30%-30%Arrival time errorATA-RTA[sec]912101043CDU outputON TIMELATE 00:12ON TIMEON TIMEON TIMEON TIMEON TIME(b) Calibrated air speed(c) Mach(d) ThrustFig. 10 Responses to wind error of RTA.6
EFFICIENT CONTROL OF ARRIVAL TIME ATA CONGESTED AIRPORT'S TERMINAL AREATable 3 Wind profiles studied for RTA function and simulation results.CASE1-11-22-12-22-32-4Wind condition simulated[kt](Wind direction 270 0000-0 ft40-40040000-40000040000-400Input toFMS[kt]000000ATA-RTA[sec]0589-6-64TIMEERRORON TIMELATE 1:05ON TIMEON TIMEON TIMEON TIME(a) CAS(b) ETA-RTAFig. 11 Response of CAS and ETA-RTA (CASE 1-1).(a) CAS(b) ETA-RTAFig. 12 Response of CAS and ETA-RTA (CASE 1-2).(a) CAS(b) ETA-RTAFig. 13 Response of CAS and ETA-RTA (CASE 2-1).(a) CAS(b) ETA-RTAFig. 14 Response of CAS and ETA-RTA (CASE 2-2).7
Y. HIGUCHI et al.(a) CAS(b) ETA-RTAFig. 15 Response of CAS and ETA-RTA (CASE 2-3).(a) CAS(b) ETA-RTAFig. 16 Response of CAS and ETA-RTA (CASE 2-4).The control strategy identified from thesimulation results was evaluated by comparingit with those from trajectory optimization usingthe same control logic (shown in Fig. 17). Figs.18 and 19 show an example of the time historiesof the off-line optimal trajectories. It revealsthat wind error compensation is effective toreduce the RTA error, even though some sort offurther studies are necessary.Fig. 17 Control dead-band of recalculation forRTA.(a) CAS(b) ETA-RTAFig. 18 RTA control by off-line optimal trajectory without a wind error compensator (Case 1-1).8
EFFICIENT CONTROL OF ARRIVAL TIME ATA CONGESTED AIRPORT'S TERMINAL AREA(a) CAS(b) ETA-RTAFig. 19 RTA control by off-line optimal trajectory with a wind error compensator (Case 1-1).Although the simulation results from theFMW are encouraging for arrival time control,it should be taken into account that the testedsimulation was a fast time simulation in whichaircraft dynamics were not taken into accountaccurately. Evaluation by a training flightsimulator or actual flight test using currentlyoperational RTA functions of FMSs isnecessary.3.2 RTA control by ground controllerAs has been discussed, arrival sequencing andtime separation are currently controlled by theen-route ground controller's vector control ofmultiple aircraft. Our previous research showedthat there would be advantages in replacing thisprocedure by speed control, which is calculatedfrom the assigned arrival time without lateralnavigation.For aircraft not equipped with all-phase RTAfunctions, a practical method of arrival timecontrol should be explored, such as velocityassignment by the ground controller during thecruise and descent phases through voicecommunication using a conventional system.The accuracy of the arrival time should beevaluated against wind prediction errors for thisapproach as in the previous section for all-phaseRTA functions of onboard FMSs. A fourdimensional trajectory estimation of the groundgenerated trajectory is expected to be moreaccurate because it uses more precise windprediction data than onboard FMSs. The voicecommunication necessary for arrival timecontrol is expected to be so limited that it couldsignificantly reduce the workload of air trafficcontrollers and pilots. Although the research isin progress, the performance of the currentlyoperational FMS all-phase RTA functions isstill the key to future system developments.Ideally, a best-equipped and best-servedenvironment could accelerate the installation ofthe function.4. Concluding remarksThis paper studies the feasibility of the RTAfunction of onboard FMSs to be used as part ofan arrival management system for TokyoInternational Airport. The all-phase RTAfunction is evaluated by an FMW, which cansimulate FMS-in-the-loop automatic flightsunder pre-assigned wind conditions. Theoptimal trajectory that corresponds to thesimulation condition is calculated using theBADAperformancemodelandthecorresponding wind conditions to evaluate theresult.The simulation reveals that- FMS optimizes its trajectory to minimize thefuel burn and flight time for a given weightingparameter CI.- When the RTA function is active, FMSoptimizes its trajectory to minimize the fuelburn with a constraint of arrival time byadjusting CI to let the ETA equal the RTA.- Wind error generates a dynamic change ofETAs, which causes the differences betweenETAs and RTAs, but the FMS recalculate newtrajectories to change the ETA when thedifference exceeds a threshold depending on thetime distance to a meter fix. As a result of thefeedback control, arrival time error is very9
Y. HIGUCHI et al.This research was financially supported by theMinistry of Land, Infrastructure, Transport andTourism in Japan via their Program forPromoting Technological Development ofTransportation. It is a partial extension of thecollaborative research on air traffic efficiencyanalysis between Kyushu University and ENRI.Numerical weather prediction GPV Datareleased by the Japan Meteorological Agencyand handled by the Research Institute ofSustainable Humanosphere, Kyoto University,and the BADA model developed by Eurocontrolare effectively used to reconstruct flightparameters from surveillance information dataand to optimize flight trajectories. Theseorganizations' support of the research is e/f-online0.html[6] EUROCONTROL Experimental center, User manualfor the base of aircraft data (BADA) Revision 3.11:EEC Technical/Scientific Report No. 13/04/16-01,2013.[7] Oka, M, Fukuda, Y and Uejima, K. Outline of AirTraffic Open Data and Application, in Japanese, The52nd JSASS Aircraft Symposium, Nagasaki, 2014.[8] Klooster,J and Smedt, D. Controlled time of arrivalspacing analysis, The 9th USA/Europe Air TrafficManagement Research and Development Seminar,Berlin, Germany, 2011.[9] Reynolds, T, McPartland, M, Teller, T and Troxel, S.Exploring Wind Information Requirements for FourDimensional Trajectory-Based Operations., The 11thUSA/Europe Air Traffic Management Research andDevelopment Seminar, Lisbon, Portugal, 2015.[10] Jones, J, McPartland, M, Reynolds, T, Glina, Y,Edwards, C and Troxel, S. Methods of SelectingForecast Winds for Flight Management Systems toSupport Four Dimensional Trajectory-BasedOperations, AIAA 2016-4069, AIAA ATIOConference, Washington DC., 2016.[11] Totoki H, Kozuka T, Miyazawa Y and Funabiki K.Comparison of JMA Numerical prediction GPVmeteorological data and airliner flight data, (inJapanese), JSASS Kouku-Uchu Gijutu (AerospaceTechnology), Vol. 12, pp. 57-63, 2013.[12] Shigetomi, S, Kozuka, Y, Totoki, H, Miyazawa, Y,Brown, M and Fukuda, Y. Evaluation analysis ofseasonal GPV meteorological data with SSR Mode Ssurveillance data, ICRAT2014, Istanbul, 2014.[13] Klooster, J. Required time of arrival (RTA)functional description, GE Aviation, 2009.ReferencesCopyright Statement[1] Study Group for the Future air traffic systems, Longterm vision for the future air traffic systems,CARATS, Collaborative Actions for renovation of airtraffic systems, URL: http://www.mlit.go.jp/common/000128185.pdf, 2010.[2] Harada,A,Kozuka,T,Miyazawa,Y,Wickramasinghe, N, Brown, M and Fukuda, Y.Analysis of air traffic efficiency using Dynamicprogramming trajectory optimization, 29th ICAS,Saint Petersburg, 2014.[3] Miyazawa, Y, Matsuda, H, Shigetomi, S, Harada, Aand Kozuka, T et al. Potential benefit of arrival timeassignment - Dynamic programming trajectoryoptimization Applied to the Tokyo InternationalAirport, 11th ATM Seminar, Lisbon, 2015.[4] Matsuda, H, Harada, A, Kozuka, T, Miyazawa, Y andWickramashinghe, N. Arrival time assignment byDynamic programming optimization, EN-A-019,EIWAC2015, 2015.[5] Japan Meteorological Business Support CenterOnline data service, URL:The authors confirm that they, and/or their company ororganization, hold copyright on all of the original materialincluded in this paper. The authors also confirm that theyhave obtained permission, from the copyright holder ofany third party material included in this paper, to publishit as part of their paper. The authors confirm that theygive permission, or have obtained permission from thecopyright holder of this paper, for the publication anddistribution of this paper as part of the ICAS proceedingsor as individual off-prints from the proceedings.limited in almost all cases, except that thenecessary control exceeds the speed limitationin the case of large head-wind errors.Although the simulation result is encouragingfor the use of all-phase RTA functions as part oftime-based arrival management systems, thissimulation neglects dynamics errors; therefore,future work will focus on evaluating the processwith more accurate simulations.AcknowledgementThis document has been created by or contains partswhich have been made available by the EuropeanOrganization for the Safety of Air Navigation(EUROCONTROL). EUROCONTROL 2016. Allrights reserved. EUROCONTROL shall not be liable forany direct, indirect, incidental or consequential damagesarising out of or in connection with this product ordocument, including with respect to the use of BADA 3.10
arrival time is controlled by changing the CI to ensure that the estimated time of arrival (ETA) is equal to the RTA. Fig. 6 plots assignable RTAs, which are limited by the earliest arrival and the latest arrival time relative to the arrival time obtained by the simulation with CI 25. Plots with different CIs (between 0 and 100)