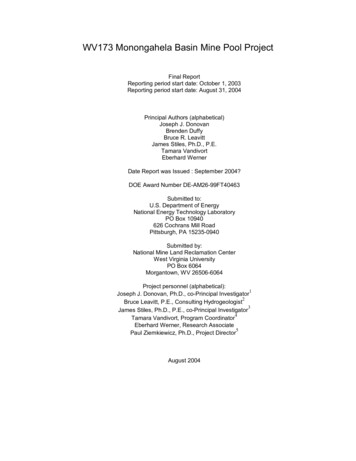
Transcription
WV173 Monongahela Basin Mine Pool ProjectFinal ReportReporting period start date: October 1, 2003Reporting period start date: August 31, 2004Principal Authors (alphabetical)Joseph J. DonovanBrenden DuffyBruce R. LeavittJames Stiles, Ph.D., P.E.Tamara VandivortEberhard WernerDate Report was Issued : September 2004?DOE Award Number DE-AM26-99FT40463Submitted to:U.S. Department of EnergyNational Energy Technology LaboratoryPO Box 10940626 Cochrans Mill RoadPittsburgh, PA 15235-0940Submitted by:National Mine Land Reclamation CenterWest Virginia UniversityPO Box 6064Morgantown, WV 26506-6064Project personnel (alphabetical):1Joseph J. Donovan, Ph.D., co-Principal Investigator2Bruce Leavitt, P.E., Consulting Hydrogeologist3James Stiles, Ph.D., P.E., co-Principal Investigator4Tamara Vandivort, Program CoordinatorEberhard Werner, Research Associate5Paul Ziemkiewicz, Ph.D., Project DirectorAugust 2004
DISCLAIMERThis report was prepared as an account of work sponsored by an agency of the UnitedStates Government. Neither the United States Government nor any agency thereof, norany of their employees, makes any warranty, express or implied, or assumes any legalliability or responsibility for the accuracy, completeness, or usefulness of anyinformation, apparatus, product, or process disclosed, or represents that its use wouldnot infringe privately owned rights. Reference herein to any specific commercialproduct, process, or service by trade name, trademark, manufacturer, or otherwise doesnot necessarily constitute or imply its endorsement, recommendation, or favoring by theUnited States Government or any agency thereof. The views and opinions of authorsexpressed herein do not necessarily state or reflect those of the United StatesGovernment or any agency thereof.
INSERT TABLE OF CONTENTS HERE
ACKNOWLEDGEMENTSThis work was assisted by numerous individuals over the course of the project whoworked on the project but whose names do not appear on the final report. Notableamong these: Jerry Steketee of the Natural Resource Analysis Center, West Virginia University,who helped with early efforts to compile mapping for the project Dave Dzombak of Carnegie Mellon University, who made early contributions onpyrite solubility and on interpretation of aqueous chemistry Rosemary Capo of University of Pittsburgh, who made strong contributions onaqueous chemistry and its relation to overburden characteristics Larry Cook, who sampled water chemistry in the field and contributed towards amultivariate analysis of these data in his Master's research at WVU Kurt McCoy, who completed Master's research on barrier leakage and rechargequantification at WVU. Jeremy Wolpert and Jeff Chaumba, who helped with fieldwork staff of the Hydrology Research Center, including Annie Morris, who ablymapped mines, modeled water levels, and performed fieldwork; Brenden Duffy,for outstanding GIS analysis, web authoring, and logger maintenance; and,especially, Eb Werner, who was with the project since its inception and has beeninvolved with virtually every aspect of its work. numerous staff of state agencies in West Virginia and Pennsylvania, forgenerous sharing of data Joe Schueck of Pennsylvania DEP, for interest and support the Eastern Mine Drainage Federal Consortium, for consultation and support indeveloping ideas behind the project Finally, Dan Sweeney, Gary Bryant, and, especially, the late Ray George of theU.S. Environmental Protection Agency, for their direct involvement and support inthe project.
1 TASK 1 –1.1 Experimental1.2 Results and Discussion1.3 Conclusion1.4 Figures and Tables
2 TASK 2 2.1 Experimental2.2 Results and Discussion2.3 Conclusion2.4 Figures and Tables
3 TASK 3 - FIELD CHARACTERIZATION3.1 Mapping of Mines in the Pittsburgh seam3.1.1 Executive summaryMine mapping products including mine outlines and flooding (circa end of 2003) maps atscale 1:24,000 have been completed on a topographic map base for 61 quadrangles in WestVirginia and Pennsylvania. They were created by obtaining original mine maps of approximatescale 1:5000, georeferencing using field- and map-locatable points, and digitization of mineoutlines and pillars. The maps show considerable variation in quality and accuracy from placeto place, depending primarily on the age and quality of available mine maps. The mine mappingwas performed specifically to address mine-flooding issues at scales of 1:24:000 or coarser.Every effort was made to preserve the relative geometry and thickness of mine barriers, even atthe expense of distortion of the mined areas themselves. This is because the flow betweenmines induced by barrier leakage is a major variable in the hydrology of mine flooding, to agreater degree than variation of mine area itself.These maps are available for public access and retrieval on www.hrc.nrcce.wvu.edu.3.1.2 ExperimentalMine maps were created in vector form at a nominal scale of 1:24,000 from original minemaps, scanned and georeferenced into a mosaic of maps. The purpose of these outlines wasto provide a base to represent both active and closed mines within the Pittsburgh seam, andwithin the closed mines, to represent state of flooding at various times.3.1.2.1 Mine dataSome of the mine outlines were obtained in digital vector form. The largest source of suchdata was from mapping compiled by the West Virginia Geologic and Economic Survey Coal-BedMapping Program (2001); data for several mines were obtained from mine operators underconfidentiality agreements.Original mine maps at various scales were used for the remaining mine outlines. Mapswere collected from various sources and scanned. Poor quality of many of the maps requiredimage-processing after scanning to improve legibility; for some maps portions remained illegibleafter repeated attempts. Many of these maps were of sections of mines and had to be joined.Very few maps were originals, and so distortions induced by scanning/photography madereconstruction of some of the mine maps difficult. Although sections were distorted to allowsplicing, these distortions did not rectify all errors induced by copying. Finally, adjacent mineswith common barriers in some cases did not agree as to the location of that barrier, and in suchcases the barrier location had to be arbitrarily chosen to maintain integrity of the mapping withrespect to the barrier geometry. For all these reasons, many maps are only approximatelyaccurate. The quality varied greatly from location to location. More recent mine maps are, ingeneral, highly accurate relative to maps of older mines.These scanned and reassembled maps were then georeferenced to modern topographicmaps, often augmented by GPS coordinates taken at recognizable features in the field that
correspond to features on the maps such as shafts or boreholes. Errors were probablyintroduced at this stage because, although the surface features shown on the mine maps weresimilar in shape and general relationships to features shown on modern topographic maps,there is no guarantee that roads have not been moved or old buildings removed and new onesbuilt nearby in a similar arrangement. Such shifts of cultural features are known to haveoccurred. Also, the quality of some of the mine maps was poor; it was often difficult to identifythe precise location on the map of features which had been defined by GPS coordinates on thesurface.After the initial georeferencing, a number of the maps underwent one or more additionalcycles of georeferencing, usually alternations of referencing one map against an adjacent mine,and then switching roles between the two maps, in order to reduce the relative error betweenadjacent mine outlines.Following georeferencing, the mine maps were digitized using ESRI’s ArcView or GoldenSoftware’s Didger. Data derived by this process were outlines of mining, outlines of unminedcoal within the general mining area, and locations of various types of openings to the mine. Theresult was a reasonable representation of the underground mined areas. However, theseresults are not completely accurate because some segments of the maps were illegible ormissing, so these portions of the mine outlines were estimated. Mining may be more extensivethan shown because some maps were not closure maps, or mining which occurred at the end ofoperations was not recorded on the mine maps.As a result of variations in mine map accuracy, it is impossible to apply a uniform estimateof inaccuracy (error) to mine-outline and barrier locations. In some locations, errors of less than50 feet are likely valid. In others, errors in excess of 200 feet are quite possible. In fact, forsome very old mine maps, it is possible that the mapping employed is highly in error due toduplication/archival difficulties with the original mine maps. For this reason, the mine mappingpresented at scales as large as 1:24,000 may be up to hundreds of feet in error. In any case, itis unlikely to be more accurate than about 20 feet, the "best case" accuracy of GPS readingsused to aid georeferencing of mine maps. The accuracy will be highest for recently-closed andactive mines, and lowest for very old, long-closed mines.3.1.2.2 Structural dataStructure contour and outcrop data were obtained from the following sources: elevations compiled by the West Virginia Geologic and Economic Survey CoalBed Mapping Program (2000)basic geological references for the area (Berryhill and others, 1971; Shaulis,1985; Skema, 1988)structure contours of the Pittsburgh coal from Roen and Farrel (1980), Fedorko(1990a, 1990b, 1990c, 1990d, 1990e, 1990f, 1990g, 1990h, 1990i, 1990j,1990k), and Ruppert and others (1997)elevations abstracted from original mine mapsoriginal mine maps, at scales from 1:1200 to 1:24,000These data were combined into a single map theme with a contour interval of 20 feet,although in some cases there was a discrepancy of more than 20 feet between overlapping datasets. Best judgement was used to adjust the contour lines in these cases of discrepancy.
3.1.2.3 Mine-associated point dataAs noted above, mine openings, such as shafts, portals, boreholes, and other openingswere digitized when found on mine maps. Additional points were derived from U. S. GeologicalSurvey topographic maps. However, most of the points were collected from field investigationsusing GPS readings.A major effort for the current period was the location and characterization of minedrainages. The search for these involved identifying probable discharge areas using acombination of the mine maps, the structure contour map, the outcrop map, and topography.3.1.2.4 Mine floodingApproximate flooding areas were delineated using the structure contour map, the miningextent map, and water level data derived from water level loggers, elevation of mine waterdischarges, and spot water elevation data using a water level tape.3.1.3 Results and Discussion3.1.3.1 Quadrangle Maps of Mine Extent (1:24,000)Mapping for mine extent, shafts, geology, and hydrogeology are presented in a series of1:24,000 quadrangles. For this project, 61 quadrangles (Appendix A), which are partly or fully inthe area of Pittsburgh seam mining, have been completed. Twenty-four of the quadrangles arenew in this report. Of the remainder, 13 were completed in Phase II of this project; 23 werecompleted in Phase III of this project, of these 7 have been revised and are included in thisreport. These maps are based on the U. S. Geological Survey topographic quadrangles withgreen (forest) and brown (contours and other elevation information) removed. Table 3.1 is a listof these quadrangles and figure 3.1 is an index map. The following themes, where suchfeatures exist, are overlaid on this base: extent of underground mine workings determined from mine mapping at variousscalesblocks of unmined coal within mine workingsstructure contour elevation of the bottom of the Pittsburgh coaloutcrop of the Pittsburgh coallocation of point-source discharge from an underground minemine shaft, existingmine shaft, sealed or closedmining-related well or borehole (location verified)mine water treatment plant.This mapping is a reasonable, but not exact, representation of the distribution ofunderground mined areas. As noted in the preceding section, the factors involved in theproduction of the data for these maps introduced certain inaccuracies. Due to the complexity ofthese factors, the absolute accuracy of specific barrier locations, mine outlines, and unminedcoal blocks may not be easily measured. However, it is estimated that errors may locally be asgreat as 100 meters, although in general the mapping may be somewhat more accurate thanthis. Mine features have been generalized; in that individual mains, longwall panels, and pillars
are not explicitly shown. Unmined coal blocks within mines were mapped only if their total areawas greater than about 20 acres, although the minimum area of individual blocks varies widely.This mapping is considered the best regional product of its type for Pittsburgh seam mining.However, it is cautioned that these maps would not be amenable to very large-scaleapplications, such as examination of local mine subsidence effects or the location of individualmine features such as gas wells, mains, etc.3.1.3.2 Quadrangle Maps of Mine Flooding (1:24,000)A similar set of maps to the Mine Extent series, also showing extent of flooding for year2003, has been prepared at a scale of 1:24,000 (Appendix B). These maps show mine extent,shafts, and discharges, but also show the estimated or modeled extent of subsurface mineflooding in December 2003, shown as a blue area (flooded) over the underlying grey area ofclosed, unflooded or free-draining mines. The rose color on these quads corresponds to activeoperations as of December 2003. In active mines, no flooding has been mapped, and thesemines are designated using their own color.3.1.4 ConclusionMine mapping products including mine outlines and flooding (circa end of 2003) maps atscale 1:24,000 have been completed on a topographic map base for 61 quadrangles in WestVirginia and Pennsylvania. These maps are available for public access and retrieval onwww.hrc.nrcce.wvu.edu.3.1.5 References citedBerryhill, H. L., S. P. Schweinforth and B. H. Kent. 1971. Coal-bearing Upper Pennsylvania andLower Permian rocks, Washington area, PA. U.S. Geol. Survey Professional Paper 621,47pp.Fedorko, Nick. 1990a. Pittsburgh coal structure contours - working map, Paden City quadrangle,WV-OH: West Virginia Geological and Economic Survey Open File Report-014, scale1:24,000. 1990b. Pittsburgh coal structure contours - working map, Porters Fall quadrangle,WV: West Virginia Geological and Economic Survey Open File Report-014, scale 1: 24,000. 1990c. Pittsburgh coal structure contours - working map, Pine Grove quadrangle,WV: West Virginia Geological and Economic Survey Open File Report-014, scale 1:24,000. 1990d. Pittsburgh coal structure contours, Big Run quadrangle, WV: West VirginiaGeological and Economic Survey Open File Report-014, scale 1:24,000. 1990e. Pittsburgh coal structure, contour map, Glover Gap quadrangle, WV: WestVirginia Geological and Economic Survey Open File Report-014; scale 1:24,000., 1990f, Pittsburgh coal structure, contour map, New Martinsville quadrangle, WV:West Virginia Geological and Economic Survey Open File Report-014; scale 1:24,000.
. 1990g. Pittsburgh coal structure, contour map, Wileyville quadrangle, WV: WestVirginia Geological and Economic Survey Open File Report-014; scale 1:24,000. 1990h. Pittsburgh coal structure, contour map, Littleton, WV-PA: West VirginiaGeological and Economic Survey Open File Report-014; scale 1:24,000. 1990i. Pittsburgh coal structure, contour map, Hundred quadrangle, WV-PA: WestVirginia Geological and Economic Survey Open File Report-014; scale 1:24,000. 1990j. Pittsburgh coal structure, contour map - working copy, Center Pointquadrangle, WV: West Virginia Geological and Economic Survey Open File Report-014;scale 1:24,000. 1990k. Pittsburgh coal structure, contour map - working copy, Folsom quadrangle,WV: West Virginia Geological and Economic Survey Open File Report-014; scale 1:24,000.Roen, J. B., and Farrel, D. E. 1980. Structure contour map of the base of the Pittsburgh coalbed, southwestern Pennsylvania and northern West Virginia. USGS Coal Investigations MapC-88.Ruppert, L., Tewalt, S., and Bragg, L. 1997. Map showing areal extent of the Pittsburgh coalbed and horizon and mined areas of the Pittsburgh coal bed in Pennsylvania, Ohio, WestVirginia, and Maryland, digitally compiled by Tully, J., Pierce., J., Weller, A., and Yarnel, J.:U.S. Geological Survey Open-File Report 96-280, scale 1:425,000.Shaulis, J. R. 1985. Coal resources of Fayette County, Pennsylvania, Part 1. Coal crop lines ,mined-out areas, and structure contours: Harrisburg, PA, Bureau of Topographic andGeologic Survey, Mineral Resource Report 91, Part 1, p. 10, 34, 66, 79, 88.Skema, V. W. 1988. Coal resources of Westmoreland County, Pennsylvania, Part 1. Coal croplines, mined-out areas, and structure contours: Harrisburg, PA, Bureau of Topographic andGeologic Survey, Mineral Resource Report 94, Part 1, p. 20, 44, 48, 57, 77,105.West Virginia Coal-Bed Mapping Project. 2001. Shape files. West Virginia Geological &Economic Survey, 1 compact disc.
3.1.6 Figures and TablesTable 3.1. List of the quadrangles of the area investigated in this project, and presentedin appendices A and rmont WestFayette CityGarards FortGlassportGlen EastonGlover GapGrant townMatherMcKeesportMidwayMonongahelaMorgantown NorthMoundsvilleMurraysvilleNew SalemOakdaleOak ForestOsagePittsburgh EastPittsburgh WestPowhatan villeSmithtonSteubenville EastTiltonsvilleValley GroveWadestownWallaceWashington EastWashington WestWaynesburgWest MiddletonWheelingWind RidgeWolf Summit
Figure 3.1. Index map of the quadrangle maps produced in this project, and included asmining extent maps in Appendix A and flooding maps in Appendix B.
3.2 Pittsburgh mine discharge chemistry3.2.1 Executive summarySpatial distribution on mine-water chemistry was plotted using 161 samples of mine watertaken during 1999-2003 at 115 locations throughout the Pittsburgh coal basin. The followingobservations have been made: pH is bimodal throughout the basin, with few exceptions, with most above-drainagemines being less than 4.5 and most below-drainage mines being above 4.5 iron is by far the highest-concentration metal and is more-or-less uniformlydistributed, with minor variations apparently related to discharge age manganese and aluminum are low in concentration and generally restricted toabove-drainage and near-outcrop mine settings. alkalinity is found primarily in deeper, below drainage mines, especially those thathave been discharging for several years. A few mines show very high alkalinities,attributed to high pCO2 values sustained in these waters net alkalinity is bimodal as for pH. Most mines that are below drainage in the basinare net alkaline, even though they carry a significant iron load. sodium is present in elevated concentrations in a few mines and appears to berestricted to immature (recent) mine discharges sulfate is generally uniform but tends to be higher in more recently flooded mines.Spatial variability of mine-water chemistry in the Pittsburgh mine basin is thus believed tobe non-random and thought to be controlled by three principal factors age of mine discharge post-flooding – the time duration for which the discharge hasexisted depth of mine and degree of exposure to oxygen, e.g. below drainage versus abovedrainage, and spatial variability between mine locations in factors such as overburdengeology/chemistry and closed-mine management (sludge injection, rock dustapplication, etc)It is possible, within limits of uncertainty, to quantitatively assess mine discharge age, althoughthis has not been attempted in this work. Depth of mining and degree of mine saturation maybe readily assessed. The third factor – spatial variability in geology and mine reclamationhandling – is not easily assessed and its role can only be speculated on the basis of empiricalobservation.
3.2.2 ExperimentalMine waters originating from mines in the Pittsburgh seam were sampled from 1999-2003.These samples are of several varieties: (1) samples from pumping of boreholes in closed mines;(b) samples from naturally-flowing portal or flowing-well discharges, (c) pumping dischargesfrom post-closure treatment plants, and (d) pumped discharges from active operations.The protocol for sampling was straightforward except for the pumped boreholes, as otherflows were continuous and thus no well purging was required. For class (1) of wells, a nominal20 gpm high-lift 3 hp pump was employed. Enough water was pumped from each well to purgethe casing 1.5 times, a purging that required in most cases several hours of pumping. Sampleswere collected in a single clean container from which samples were drawn for filtration. Onefiltered (0.45 µ) aliquot was chilled immediately, the other was acidified with 1% by volumeconcentrated hydrochloric acid. Raw pH and conductivity were measured in the field in thepump discharge. Alkalinity was determined in the field for samples with pH 4.5 on the rawsample using a Hach digital titrator dispensing 1.6 N sulfuric acid; thereupon it was used toestimate HCO3, CO3, and pCO2 concentrations using the equilibrium solver PHREEQC. Thefiltered acidified sample was thereupon used for analysis of major dissolved cations (Ca, Mg,Na, K, Fe, Mn, Al) by inductively-coupled plasma (ICP) spectrometry. The filtered unacidifiedsample was analyzed using Lachet colorimetry for most anion analytes (Cl, F, NO3, SO4). SO4gave some significant charge balance errors in the samples through 2002, attributed to loss ofsulfate by adsorption onto ferric oxyhydroxides in the unacidified sample; this precipitationoccurred especially rapidly in the alkaline samples. Therefore, in 2003 samples, the analysis forSO4 was done by ICP spectrometry, with better results and charge balances.Alkalinities were measured directly by field titration. Net alkalinity was also calculated usinga combination of measured alkalinity and calculated metal plus hydrogen ion acidity. Metalacidity was calculated using stoichiometric coefficients of 2, 2, and 3 for Fe, Mn, and Al,respectively. This makes the implicit assumption that all iron and manganese are in a fullyreduced state in the water discharging from each mine. This assumption is easily justified forbelow-drainage mines, and not highly in error for oxic mine discharges.3.2.3 Results and DiscussionThe samples listed in Table 3.2 include all samples previously reported in earlier phases ofthe project, from 1999-2002, as well as those sampled and analyzed in 2003. Correspondingsample locations are shown in Figure 3.2.3.2.3.1 Spatial distribution of chemistry3.2.3.1.1MetalsIron concentrations (Figure 3.3) range from less than 10 mg/L in a small number of minesalong the Monongahela River east of Waynesburg (small dots) to hundreds of mg/L in deeprecently-flooded mines. In the West Fork area south of Fairmont, and in the Irwin Basinsoutheast of Pittsburgh, iron concentrations are generally lower than 50 mg/L, in areas wheremines have been closed for a long period. In deeper mines iron concentrations tend to behigher. The distribution of iron concentrations is notable for its relative uniformity, exceptingselected areas of shallow mines that have been discharging for long periods
Manganese concentrations (Figure 3.4) are substantially lower than iron and show adifferent spatial pattern. Deep mines are relatively low in manganese, rarely exceeding 25mg/L. There is one cluster of higher concentrations, from shallow above-drainage mines in theMorgantown area. Manganese is most commonly a minor proportion of mine water acidity.Aluminum concentrations (Figure 3.5) in nearly all deep mine waters are very low, 10mg/L. The only cluster of elevated aluminum concentrations are in shallow and near-outcropmining near the West Virginia-Pennsylvania border north of Morgantown.3.2.3.1.2pH and AlkalinityMeasured field alkalinity – the sum of carbonate and hydroxyl alkalinity in mg/L of calciumcarbonate equivalents – is shown for the basin in Figure 3.6. A large number of minesdischarge water that has alkalinity (grey dots in Figure 3.6). These are the dark-blue circles inFigure 3.7. A number of above-drainage and near-outcrop mines have pH 4 and zero alkalinity(red dots on Figure 3.7). Only a few mines have discharges which lie between pH 4 and 5(yellow dots on Figure 3.7).It is not an unexpected result that shallow and above-drainage mines should show low pHand zero alkalinity. However, the magnitude of the alkalinity at a small number of mines(Jamison 9, as well as a series of mines on the east side of the Monongahela east ofWaynesburg) is in the very high range for typical groundwater, 600 mg/L. Even limestoneaquifers rarely attain this level of dissolved carbonate alkalinity.Net alkalinity – that is, measured field alkalinity minus hydrogen ion and metal acidity, all inmg/L as CaCO3 – is shown in Figure 3.8. This is similar data to Figure 3.6 for samples with verylow metals and pH 4.5, but adds the small orange circles, which represent the magnitude of netacidity for samples that contain both substantial metal concentrations and, in some cases,dissolved alkalinity. Thus the yellow symbols represent net alkaline chemistry, with themagnitude of residual alkalinity (after all metals are neutralized) represented by size of symbol,and the orange symbols represent scaled net acidity, the residual metal acidity after all alkalinityis consumed.3.2.3.1.3SodiumDissolved sodium (Figure 3.9) indicates that, in general, shallow-mine discharges have verylow sodium ( 100 mg/L). Deep mines, on the other hand, show generally higher values, from100-1500 mg/L. In several active mines, the values exceed 1500 mg/L, generally the highestobserved in the basin.It has been known that, early in flooding history, sodium concentrations are initially high,then exponentially decline once initial flushing of the mine is complete (Donovan and others,2003). Thus, much of the observed spatial variation may be related to this “temporal factor”,and sodium concentrations may be expected to decline in recently-closed operations. Capoand others (2001) have outlined an ion-exchange mechanism for mine waters by which highsodium concentrations are obtained soon after the roof-rock above the coal is exposed to water.This mechanism may be deemed to apply in cases where chloride is much lower in molarconcentration than sodium, as is the case for these samples; only three locations exceed 200mg/L.
While temporal variability is thought to be a key factor, there may also be some spatialand/or geologic factors involved in where the higher sodium values are observed. For example,the Clyde discharge (initial flooding 1997) had sodium of 2210 mg/L in 2003, while Arkwright(initial flooding 2001) varied between 380 and 481 in 2001. Apparently, some regions of thebasin contain overburden rocks over the coal that are higher in sodium than others. In bothcases it is expected that concentrations will become lower with time after initial dischargeoccurs.3.2.3.1.4SulfateSulfate concentrations (Figure 3.10) range from 200-9508 mg/L in the basin, a considerablevariance. The lowest concentrations are in areas of above-drainage and very old mining, suchas West Fork and the west Uniontown complex, as well as along Chartiers Creek and otherareas of outcrop mining. The highest concentrations are in recently-closed deep mines,especially in Greene County, Pennsylvania, and in Monongalia and Marion counties, WestVirginia, on the eastern side of the basin.Sulfate is by far the dominant anion in these mine waters, representing 93% of the totalequivalent anion concentration. Its distribution is therefore a good surrogate for total dissolvedsolids, as may be seen by comparing Figure 3.11 with Figure 3.10.As a result, it may be inferred that age of mine discharge is an effective control on theabsolute concentration of dissolved solids. Older mine discharges tend to be more dilute, andhence lower in dissolved solids and sulfate. The spatial pattern of Figure 3.10 is somewhatsimpler than that of the metals (Figures 3.3, 3.4, and 3.5), which have other factors than simplymine discharge age related to their occurrence.3.2.4 ConclusionSpatial variability of mine-water chemistry in the Pittsburgh mine basin is non-random andthought to be controlled by three principal factors: age of mine discharge post-flooding – the time duration for which the discharge hasexisted depth of mine and degree of exposure to oxygen, e.g. below drainage versus abovedrainage, and spatial variability between mine locations in factors such as overburdengeology/chemistry and closed-mine management (sludge injection, rock dustapplication, etc)It is possible, within limits of uncertainty, to quantitatively assess mine discharge age, althoughthis has not been attempted in this work. Depth of mining and degree of mine saturation maybe readily assessed. The third factor – spatial variability in geology and mine reclamationhandling – is not easily assessed and its role can only be speculated on the basis of empiricalobservation.
3.2.5 References citedCapo, R. C., Winters, W. R., Weaver, T. J., Stafford, S. L., Hedin, R. S., Stewart, B.W. 2001.Hydrogeologic and geochemical evolution of deep mine discharges, Irwin syncline,Pennsylvania. Proc. 22nd West Virginia Surface Mine Drainage Task Force Symposium, p.144-153.Donovan, J. J., L
Pittsburgh, PA 15235-0940 . Submitted by: National Mine Land Reclamation Center West Virginia University PO Box 6064 Morgantown, WV 26506-6064 Project personnel (alphabetical): Joseph J. Donovan, Ph.D., co-Principal . Mapping for mine extent, shafts, geology, and hydrogeology are presented in a series of the area of Pittsburgh seam mining .