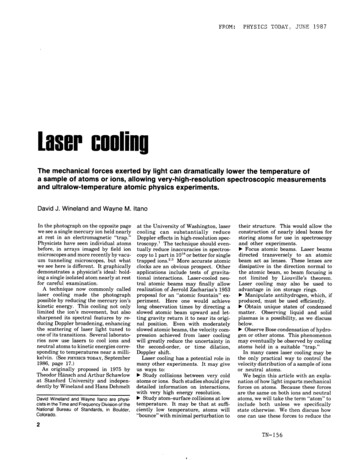
Transcription
FROM:PHYSICS TODAY, J U N E 1987The mechanical forces exerted by light can dramatically lower the temperature ofa sample of atoms or ions, allowing very-high-resolution spectroscopic measurementsand ultralow-temperatureatomic physics experiments.David J. Wineland and Wayne M. ltanoIn the photograph on the opposite pagewe see a single mercury ion held nearlyat rest in an electromagnetic “trap.”Physicists have seen individual atomsbefore, in arrays imaged by field ionmicroscopes and more recently by vacuum tunneling microscopes, but whatwe see here is different. It graphicallydemonstrates a physicist’s ideal: holding a single isolated atom nearly at restfor careful examination.A technique now commonly calledlaser cooling made the photographpossible by reducing the mercury ion’skinetic energy. This cooling not onlylimited the ion’s movement, but alsosharpened its spectral features by reducing Doppler broadening, enhancingthe scattering of laser light tuned toone of its transitions. Several laboratories now use lasers to cool ions andneutral atoms to kinetic energies corresponding to temperatures near a millikelvin. (See PHYSICS TODAY, September1986, page 17.)As originally proposed in 1975 byTheodor Hansch and Arthur Schawlowat Stanford University and independently by Wineland and Hans DehmeltDavid Wineland and Wayne ltano are physicists in the Time and Frequency Division of theNational Bureau of Standards, in Boulder,Colorado.at the University of Washington, lasercooling can substantially reduceDoppler effects in high-resolution spectroscopy.’ The technique should eventually reduce inaccuracies in spectroscopy to 1part inor better for singletrappedMore accurate atomicclocks are an obvious prospect. Otherapplications include tests of gravitational interactions. Laser-cooled neutral atomic beams may finally allowrealization of Jerrold Zacharias’s 1953proposal for an “atomic fountain” experiment. Here one would achievelong observation times by directing aslowed atomic beam upward and letting gravity return it to near its original position. Even with moderatelyslowed atomic beams, the velocity compression achieved from laser coolingwill greatly reduce the uncertainty inthe second-order, or time dilation,Doppler shift.Laser cooling has a potential role inmany other experiments. It may giveus ways to:b Study collisions between very coldatoms or ions. Such studies should givedetailed information on interactions,with very high energy resolution.b Study atom-surface collisions at lowtemperature. It may be that a t sufficiently low temperature, atoms will“bounce” with minimal perturbation totheir structure. This would allow theconstruction of nearly ideal boxes forstoring atoms for use in spectroscopyand other experiments.b Focus atomic beams. Laser beamsdirected transversely to a n atomicbeam act as lenses. These lenses aredissipative in the direction normal tothe atomic beam, so beam focusing isnot limited by Liouville’s theorem.Laser cooling may also be used toadvantage in ion storage rings.b Manipulate antihydrogen, which, ifproduced, must be used efficiently.b Obtain unique states of condensedmatter. Observing liquid and solidplasmas is a possibility, as we discussbelow.b Observe Bose condensation of hydrogen or other atoms. This phenomenonmay eventually be observed by coolingatoms held in a suitable “trap.”In many cases laser cooling may bethe only practical way to control thevelocity distribution of a sample of ionsor neutral atoms.We begin this article with an explanation of how light imparts mechanicalforces on atoms. Because these forcesare the same on both ions and neutralatoms, we will take the term “atom” toinclude both unless we specificallystate otherwise. We then discuss howone can use these forces to reduce the2TN-156
Single ion of mercury. The white dot atthe center of this false-color image is alaser-cooled Hg ' ion in an electromagnetictrap. This image was made with a photoncounting imaging tube sensitive to the 194nm fluorescence light scattered from theion and trap electrodes. The white overlayshows the position of the electrodes of theion trap. The circular ring electrode has aninner diameter of about 0.9 mm. Tophotograph such an ion requires aminimum exposure time of about 50 psec.The effective temperature of the ion wasapproximately 2 mK. The computerizedimaging system used here was developedby Charles Manney and John Bollinger atthe National Bureau of Standards. The firstphotographic images of single ions werereported in reference 15.kinetic energy of a sample. Finally, wediscuss what can be achieved in thelaboratory.Early history. The study of the mechanical forces that light exerts onmatter has a long history. In 1873James Clerk Maxwell used the theoryof electromagnetism to calculate theforce on a solid body due to the absorption or reflection of a beam of light. Inthe early 1900s quantitative measurements of the force exerted by light onsolid bodies and gases verified Maxwell's radiation pressure calculation . In 1917 Albert Einstein used quantumtheory to calculate the influence of theelectromagnetic radiation field on themotion of molecule . He showed thatthe light pressure causes molecules tocome into thermal equilibrium with aradiation field if that field has thePlanck spectrum. Aside from showingthe consistency of quantum theory andstatistical mechanics, this calculationwas important in establishing thequantum nature of light, because it wasnecessary to assume that the moleculeemits radiation as a discrete bundlewith a definite energy and momentum,and not as a spherical wave.In 1933 Otto Frisch did the firstexperiment to show directly the momentum transferred to an atom by theabsorption of a photom6 In this experi-ment, light from a sodium resonancelamp deflected a beam of sodium atoms.When tunable lasers became availablein the 1970s, experiments of this sortwere repeated. Due to the much higherspectral intensities available, an atomcould be made to absorb many photonsone at a time, but at a high rate,resulting in larger deflections. Aroundthis time physicists made proposals touse intense, resonant optical fields tomanipulate atoms in various ways,such as accelerating them or trappingthem in optical potential wells. Amongthose who first recognized the possibleapplications of resonant laser radiationpressure on atoms were Arthur Ashkinin the United States and several scientists in the Soviet Union.7 In his 1950paper on optical pumping, AlfredKastler suggested some ways of usinglight to cool or heat atoms.' Theseideas are related to laser cooling butare difficult to realize in practice.Optical forces on atomsThe force that light exerts on atomsis often conceptually divided into twop a r k g These are called the light pressure or scattering force and the gradient or dipole force. At least in somesimple cases, one can distinguish theseforces clearly. In the general case, andparticularly for intense fields, the sim-ple descriptions of the forces and theirfluctuations break down, and a morefully quantum mechanical descriptionis required.','"We can understand the scatteringforce as the momentum transferred tothe atom as it scatters a photon. Theaverage scattering force is in the direction of propagation of the light and isequal to the product of the momentumtik per photon and the photon scattering rate. The photon wavevector k hasmagnitude 27 //2,where A is the wavelength of the light. The average forcereaches a maximum when the light isresonant with an atomic transition.The scattering force fluctuates becausethe photons scatter at random timesand because the direction of the reemitted photon, and hence the direction of the recoil momentum due to thisre-emission, is also random.To see how large the scattering forcecan be, let us consider a specific example. Assume that the light from asingle laser beam is resonant with thelowest-frequency atomic transitionand that the light is intense enough tosaturate the transition, that is, thatthe rate for stimulated emission exceeds the spontaneous decay rate.When saturated, the atom spendsabout half of its time in the excitedstate. Therefore the average force onTN-1573
\- -- /"\Scattered photons-ck1)I .)0Incident photonsLaser cooling mechanism for free or weakly bound atoms or ions."Weakly bound" means that the oscillation period of the trapped atomor ion is longer than the lifetime of the upper state of the coolingtransition. The frequency q of incident photons is assumed to beless than the rest frequency o0of the atomic transition. At a velocitywhere the atom's transition frequency oois equal to (1 - k.v)o,, theatom resonantly scatters photons. When the photon wavevector k isantiparallel to the atom's velocity v, the average reduction in theatom's velocity is fik/m per scattering event, where m is the atom'smass.one atom is equal to the product of thephoton momentum fik, the rate y ofspontaneous decay from the excitedstate, and the probability of being inthe excited state, or fiky/2. Note thatabsorption followed by stimulatedemission imparts no net momentum tothe atom because the photon resultingfrom stimulated emission also has momentum fik.If the wavelength is 500 nm and thedecay rate y is 108/sec,then the scattering force is about 40 000 times the forceof gravity for an atom of mass 100 u,oratomic mass units. This force is thesame as the electric force on a singlycharged ion in a field of about 5 x lo-'V/cm. Therefore, the scattering forcecan be much greater than the gravitational force, but is weak compared withtypical electric forces. In practice,about lo4 scattering events are required to change atomic velocities by asmuch as room temperature thermalvelocities. The basic idea of lasercooling, then, is to have the atompreferentially scatter photons when itsmomentum and the photon momentumare antiparallel.The origin of the gradient or dipoleforce is somewhat less obvious, although certain of its properties do havea classical interpretation. The atomcan be thought of as a polarizable body.The optical electric field induces anelectric dipole moment in the atom andthen acts on that dipole moment. If theoptical intensity is spatially inhomogeneous, the dipole interaction causes aforce along the gradient of the intensity. Like the scattering force, the dipoleforce has a strongly resonant character, but unlike the scattering force, it isdispersive in nature. Its sign is suchthat it attracts an atom to a region ofhigh light intensity if the frequency ofthe light is below the atomic resonanceand repels an atom if it is above. Thatis, the electric polarizability is positivebelow resonance and negative aboveresonance. To this extent, the atom islike a charged harmonic oscillator-anelectron bound to a positive core by aspring, in an oscillating electric field.The charge oscillates in phase with theexternal force if the frequency is belowresonance, and 180"out of phase if thefrequency is above. If the electric fieldis spatially inhomogeneous, then averaged over one cycle of the opticalradiation a net force on the atom canresult. Some proposals for trappingatoms with optical fields are based onthe use of the dipole force.Laser cooling of free atomsLaser cooling based on the use of thescattering force can be explained asfollows: Consider an atom with astrongly allowed resonance transition.For simplicity, let this be the lowestfrequency transition, so that if thistransition is excited, the atom mustdecay to the ground state. A laserbeam whose frequency is close to butlower than the atomic resonance frequency irradiates the atom. If theatom is moving against the laser beam,then the frequency of the light in therest frame of the atom is Dopplershifted toward resonance. Hence, thescattering force is higher for an atommoving against the laser beam, and theatom's velocity is damped. In this way,the velocity of an atomic beam can bereduced substantially.If only one laser beam is used and theatom is otherwise free, the atom willeventually turn around and move awayparallel to the beam. Therefore, for agas of atoms, if another laser beam ofequal intensity and frequency but opposite direction is introduced, atoms withtheir velocities in the other directionalso have their speeds reduced. Theintensities must be equal for the average scattering force on a motionlessatom to be zero; if the intensities arenot equal, the atoms will have a netdrift velocity. One can obtain coolingin all directions by using three orthogonal pairs of counterpropagating laserbeams. However, because of the inherent fluctuations of the scattering force,the velocity is not damped to zero.The theoretical minimum temperature that can be obtained is given by abalance between the dissipation andfluctuations.'." Assume the laser intensity is below saturation, even whentuned to resonance. We also assumethat the recoil energy R, given by (fik)'/2 m , is less than fiy. The recoil energy isthe kinetic energy that an initiallymotionless atom of mass m would havedue to its recoil after emitting a photonof wavevector k . The minimum temperature Tmin is achieved when thelaser frequency is tuned below theatomic resonance frequency by anamount equal to y / 2 , in whichkTmin V2fiyFor a decay rate y of 10s/sec, theminimum temperature is about 0.38mK.4TN-158
20ka:0fflm oo- 20,.t of laserFrequencyO0OV00t,,Carrier,,for coolingLASER FREQUENCY w LAbsorption as a function oflaser frequency In the sideband coolinglimit, the oscillation frequency 0, of the atom in the trap is assumed tobe larger than the radiative decay linewidth y Therefore theabsorption spectrum consists of a “carrier,”or recoilless line, at theatom’s rest frequency ma plus Doppler-effect sidebands separated bythe frequency 0, If the laser is tuned to oa - o,,the atom absorbsphotons of energy fi(o, - 0,)and re-emits photons of average energyha- R, where the recoil energy R is (fik)’/2m When R IS much lessthan h,there is an energy deficit of approximatelyper scatteringevent, causing a decrease in the kinetic energy of the atomThe laser cooling effects just described rely only on the scatteringforce. There is another cooling effect,called “stimulated cooling,” which isdue to the dipole force in a strongstanding wave.’2 It is effective only forlow atomic velocities and requires thatthe laser frequency be higher than thetransition frequency. In a high-intensity, nearly resonant field, the simplestdescription of the atom-field system isin terms of dressed-atom states, whichare coherent superpositions of theground state atom with n 1 photonsin the field and the excited state atomwith n photons. The dressed-atomstates depend on the light intensity,and hence on spatial position. Thevarious dressed-atom states are eitherattracted to or repelled from regions ofhigh light intensity. The rate of spontaneous decay, which leads to transitions between different dressed-atomstates, is proportional to the admixtureof excited atomic states in the dressedatom state. It turns out that forpositive laser detuning, the time-averaged dipole force opposes the velocity ofthe atom. It should be possible todecelerate or accelerate beams of atomsby changing the relative frequencies oftwo counterpropagating laser beams tocreate a moving standing wave. Atomswith velocities close to that of thestanding wave could be swept alongwith the standing wave. An advantageof the dipole force is that it continues toincrease as the intensity increases,while the scattering force approaches alimiting value when the scattering rateis about y / 2 . However, the minimumachievable temperature using stimulated cooling is no lower than thatgiven by equation 1.Laser cooling of trapped atomsFor trapped atoms, the theoreticallimit to cooling is the same as for freeatoms when the natural linewidth y ofthe atomic transition used for cooling ismuch greater than the motional frequency a,.of the atom in the trap. Thereason for this is that the atom isessentially free during the time required to scatter a photon. This is theusual experimental case for a stronglyallowed transition. For trapped atomsit is not necessary to have opposedbeams with equal intensities, becausethe average light pressure just displaces the equilibrium position of theatom slightly. It is possible to cool witha single laser beam as long as none ofthe normal modes of oscillation in thetrap are perpendicular to the directionof propagation of the beam.In the opposite limit, where thenatural linewidth of the transition ismuch less than the motional frequencies, the cooling limit is different.””The absorption spectrum of the atomconsists of an unshifted resonance lineat frequency a,,,called the carrier, anda series of discrete lines on both sides ofthe carrier, each having the naturallinewidth and separated by multiplesand combinations of the motional frequencies. These extra lines, calledmotional sidebands, are due to theperiodic frequency modulation of thelight owing to the Doppler effect asobserved by the moving atom.To cool a trapped atom, one tunes anarrow-band laser to a sideband on thelow-frequency side of the unshiftedresonance, for example, to a frequencywo -PO,, where p is an integer. Theatom makes transitions to the upperelectronic state, decreasing its vibrational energy, by absorbing photons ofenergy fib, -pa,). When the atommakes a transition back to its groundelectronic state, it may, in general,either increase or decrease its vibrational energy, but the average changein the vibrational energy is equal to therecoil energy R. When R is less thanp h , ,, cooling occurs.Consider a particular case. A singleion is trapped in a nearly isotropicthree-dimensional harmonic potentialwell, a situation that is approximatedI3by an ion in an rf trap, or Paul trap,with normal-mode vibrational frequencies all approximately equal to a(,.Aquantum state of the atom in the well isidentified by its internal quantumnumbers and the set of harmonic oscillator quantum numbers 1 n, ,n, ,nz 1 corresponding to the well. Three laserbeams propagate along the x , y and zaxes, and each is tuned to the corresponding first lower sideband. Weassume that the recoil energy R ismuch less than the energy tUL)(,, acondition that is not hard to satisfy inpractice. In the steady state, the meanvalues of the quantum numbers are“(n, 2 nv ( n , 5/,6(v/c0,.2) 1(2)There are two reasons that the meanvalues do not go to zero with lasercooling. First, the average change R inthe atom’s vibrational energy after it5TN-159
Stopping sodium atoms with light. A beam of sodium atoms approaching the camera fromthe upper right at speeds exceeding 1000 m/sec is brought to a virtual standstill by a laserbeam aimed in the opposite direction. In this experiment at NBS, Gaithersburg, the atomstravel down the axis of a solenoid (whose opening appears as the dark circle at the center ofthe photograph) and spread out as they come to a virtual halt near the opening.'"emits a photon is positive. Second,there is some probability of driving atransition that leads to an increase invibrational energy on absorption rather than a decrease, because the neighboring sidebands, although far fromresonance, still have finite intensities.The simple theory just outlined applieswhen the cooling transition is notsaturated. Markus Lindberg at theUniversity of Frankfurt and Juha Javanainen and Stig Stenholm at theUniversity of Helsinki have calculatedthe steady state for a n arbitrary ratioof natural linewidth to motional frequency and also for arbitrary laseri n t e n i t y . ' As in the case of freeatorris, the lowest temperatures areachieved in the limit of low intensity, sothe simple theory is usually adequate.'.' IExperiments on trapped ionsIt is perhaps not surprising that thefirst laser cooling experiments were ontrapped ions, because ions can be heldfor long periods in high vacuum with afairly high degree of thermal isolation.13 Therefore, unlike in the case ofneutral atoms, a long time is availableto do the cooling.The first laser cooling experimentswere done in 1978. At the NationalBureau of Standards in Boulder, Robert Drullinger, Fred Walls and Wineland demonstrated cooling by a directobservation of ion temperature, whichthey determined from the currents thation motion induces in trap electrodes.They observed the cooling of Mg ionsto 40 K in a Penning trap.".'3 At thesame time in Heidelberg, Werner Neuhauser, Martin Hohenstatt, Peter Toschek and Dehmelt demonstrated cooling of Ba ions in a Paul trap byobserving the ions' increased storagetime in the trap.I5In subsequent experiments a t theseand other laboratories, physicists havemeasured temperatures on the order of10 mK or less. They have typicallydetermined the temperatures by measuring the contribution of Dopplerbroadening to spectral lines. Forstrongly allowed electric dipole transitions, this method is not very sensitivea t low temperatures because Dopplerbroadening contributes only a smallfraction of the overall linewidth. Forpositive magnesium-24 ions a t 1 mK,for example, the Doppler broadening ofthe first resonance line (at 280 nm) isonly 0.5 MHz, while the natural radiative linewidth y/2v is 43 MHz. Onerealizes a more sensitive measurementof temperature by probing a transitionwith a linewidth y that is much lessthan the motional oscillation frequencyo, of an ion in the trap.15 Here one canuse the strength of the motional sidebands to determine the ion temperature.I6 So far, only cooling in the limity o , (equation 1) has been demonstrated for ions, but if cooling in thelimit o, y is used, it should be possibleto reduce the kinetic energy to nearlythe zero-point energy (equation 2). Laser cooling of trapped ions is now donein laboratories at NBS in Boulder,Hamburg University, the University ofWashington in Seattle, the Universityof Paris in Orsay, the National Physical Laboratory at Teddington in England and the Max Planck Institute at,Garching in West Germany. Otherlaboratories are setting up similar experiments.Single ions. Single, laser-cooled,trapped ions are interesting becausethey provide a simple system for study.First, the oscillation frequencies of anion in a trap are nearly harmonic,which makes spectra of single ionsparticularly simple. In contrast, if twoor more ions are stored together in atrap, the oscillation frequencies of theindividual ions are dominated by ionion interactions at low temperature.Second, the lowest possible kinetic energies, given by equations 1 and 2, areobtained for single ions. This is because part of the motion in the Paultrap (the rf-driven micromotion) or inthe Penning trap (the magnetron orrotation motion) is nonthermal and notaffected in the same way by laser ooling.' The kinetic energy in thesenonthermal motions can be minimizedfor single ions. Finally, the simplicityof single trapped ions allows a straightforward comparison of laser coolingtheory and experiment.Single ions are also interesting fromthe standpoint of spectroscopy becausethe perturbations in spectral measurements can be extremely small or wellcontrolled.'-" Historically, the mostdifficult problem in high-accuracy iontrap spectroscopy has been minimizingthe second-order Doppler frequencyshift, which is due to relativistic timedilation.'*'Reducing this systematic effect requires low temperatures.Experimenters have achieved temperatures of about 10 mK or less with singleBat ions at Hamburg University, withsingle Mg and Ba' ions at the University of Washington, with single Mg'and Hg ions at NBS in Boulder andwith single Mg' ions a t Garching. At atemperature of 10 mK, an ion withmass 100 u has a fractional second order Doppler shift of - 1 . 4 10-17,which is almost negligible. Single la-6TN-160
ser-cooled ions have also allowed detailed studies to be made of the interaction between atoms and radiation, asseen, for example, in quantum “jumps”and in photon “antibunching,” an effect in which the distribution of arrivaltimes of fluorescence photons at adetector is nonclassical because atomscan emit only one photon at a time.Liquid a n d solid plasmas. Largenumbers, or “clouds,” of trapped ionsare more properly referred to as nonneutral ion 1 a s m a s . IThese plasmasare interesting because, unlike fusionplasmas, they can reach a global thermal equilibrium. When these plasmascan be laser cooled, the densities arehigh enough-above 1O7/cm3-and thetemperatures are low enough-lessthan 10 mK-that the plasmas becomestrongly coupled and should show liquid and solid b e h a i 0 r . lIt should bepossible to obtain non-neutral plasmaswhose dynamics are dominated byquantum effects-for example, a positron plasma “sympathetically” cooledwith laser-cooled ions of beryllium-9, asdescribed below.Even though single ions give thelowest temperatures, larger samples oflaser-cooled trapped ions have alreadyyielded interesting spectroscopic results. Spectroscopy“ in the rf andmicrowave regions has featuredlinewidths of 0.01 Hz and fractionalinaccuracies as small as 1 part in IO’,’.Sympathetic laser cooling. Unfortunately, direct laser cooling of ions andneutral atoms is relatively easy onlyfor a very few elements. Sodium isoften the practical choice for neutralatom researchers; singly ionized magnesium, which is isoelectronic withsodium, is a favorite choice for iontrappers. So far both groups haveavoided working with molecules. Thereason is simply that if one drives asuitably allowed transition at a convenient wavelength in a molecule, themolecule quickly becomes opticallypumped into a state of different vibrational or rotational quantum numberand cooling stops.One can extend laser cooling to otherspecies of ions by storing two ionspecies in the same trap. The speciesthat is easy to laser cool will cool thesecond species through Coulomb collisions, making the second species available for high-resolution spectroscopicinvestigation or other experiments.Under typical conditions, ion-ion thermalization by Coulomb coupling takesplace in less than a second. As ademonstration of this technique, Hg ’ions have been cooled to less than 1 Kby laser-cooled ions of beryllium-9 in aPenning trap.I7 It should be possible toextend sympathetic cooling in order tocool neutral atoms or molecules by ion-‘Optical molasses’ holding approximately 2 x 1O5 sodium atoms at a temperature of about240 p K The atomic sample, which IS the orange ball in the photograph, is at the intersectionof SIX collimated laser beams that form the optical molasses It is made visible by the lightthat the atoms scatter out of the laser beams The “ball” of atoms is roughly 7 mm indiameter The green light is from a pulsed laser used to evaporate sodium atoms from thesolid (Photograph courtesy of Bell Laboratories )this requires about 50 cm. The firstneutral-atom cooling experiments wereExperiments on neutral atomsreported by S. V. Andreev, Victor BalyIf neutral atoms could be trapped kin, Vladilen Letokhov and Vladimireasily and held in thermal isolation Minogin in Moscow and by Williamfrom the surroundings, then laser cool- Phillips and Harold Metcalf at NBS ining them would be similar to laser Gaithersburg, Maryland. The Moscowcooling ions. Unfortunately, neutral- group saw beam slowing and velocityatom traps with the required thermal compression due to a counterpropagatisolation are not very deep. For exam- ing fixed-frequency laser beam. If aple, magnetic traps, which convert the fixed-frequency laser beam is used,atom’s kinetic energy into internal, atoms with velocities that put them inZeeman energy, at best have a depth of resonance with the laser through theabout 5x10 - 4 eV, or 8 K. This as- first-order Doppler frequency shift aresumes that an atom with a .nagnetic efficiently slowed. However, thesemoment equal to one Bohr magneton is atoms are soon slowed enough thatcaptured in a magnetic well that is 10 T they are Doppler shifted out of resodeep. Ion traps, in contrast, can be nance with the laser beam and theslowing is greatly reduced. Similarly,kilovolts deep.Most neutral-atom laser cooling ex- faster atoms are slowed only veryperiments start with an atomic beam of slightly. The result is that a hole isan alkali such as sodium and slow and carved out of the atoms’ velocity districool the beam with a counterpropagat- bution at a velocity corresponding toing laser beam.IH Because sodium the Doppler-shifted laser frequency,atoms are emitted from an oven source and the affected atoms tend to bunch atwith a velocity of about 1000 m/sec, the slightly lower velocity.slowing and cooling must be done veryTo make the cooling more efficient,efficiently to stop the atoms before they one needs a way to keep the atoms instrike some portion of the apparatus. resonance with the laser while they areEven at maximum cooling efficiency, slowed down. The NBS group accomatom or atom-atom collisions7TN-161
plished this by continuously tuning theatoms’ frequency. Investigators fromthe group directed the atomic beamdown the bore of a solenoid whosemagnetic field varied with position.The field varied in such a way as tokeep the slowed atoms, whose Zeemanfrequency shift depended on position inthe magnet, continuously in resonancewith the fixed-frequency laser beam.This enabled more of the atoms to beslowed to lower velocities. Recent experiments at MIT have also used thistechnique.Another way to keep the atoms tunedto the frequency of the laser is to sweep,or “chirp,” the frequency at a n appropriate rate. The first demonstration ofbeam slowing by this technique wasmade in 1983 at NBS, Gaithersburg.Physicists at the Joint Institute forLaboratory Astrophysics later used thesame technique to slow and stopatoms.” Subsequent experiments atBell Laboratories,” Bonn University,the State University of New York atStony Brook, the Ecole Normale Superieure in Paris and by another group atJILA2’ have employed laser chirpingfor cooling. The latter experiments atJILA accomplished slowing and coolingusing diode
tion of propagation of the light and is equal to the product of momentum tik per photon and the photon scatter- ing rate. The photon wavevector k has magnitude 27 //2, where A is the wave- length of the light. The average force reaches a maximum when the light is resonant with an atomic transition. The scattering force fluctuates because