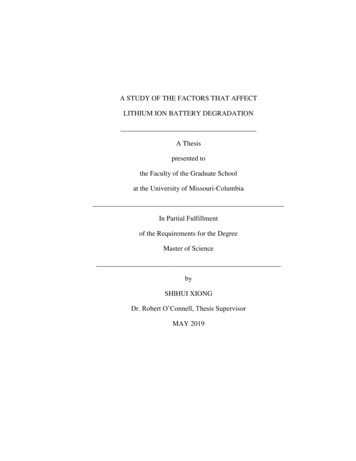
Transcription
A STUDY OF THE FACTORS THAT AFFECTLITHIUM ION BATTERY DEGRADATIONA Thesispresented tothe Faculty of the Graduate Schoolat the University of Missouri-ColumbiaIn Partial Fulfillmentof the Requirements for the DegreeMaster of SciencebySHIHUI XIONGDr. Robert O’Connell, Thesis SupervisorMAY 2019
The undersigned, appointed by the dean of the Graduate School, have examined thethesis entitledA STUDY OF THE FACTORS THAT AFFECTLITHIUM ION BATTERY DEGRADATIONpresented by Shihui Xiong,a candidate for the degree of Master of Science,and hereby certify that, in their opinion, it is worthy of acceptance.Professor Robert O’ConnellProfessor Justin LegarskyProfessor Lin Yuyi
ACKNOWLEDEMENTSI would like to thank Dr. Robert O’Connell for his instruction andcontinuous support throughout my Master’s study. I gained tremendous insight inscientific research as well as academic writing. I really appreciate his patience anddedication toward the completion of my master thesis as well as every single stepalong the way. It was his encouragement and trust that lead me through this program.I would also like to thank Dr. Justin Legarsky and Dr. Lin Yuyi for beingmy thesis committee members and their valuable advices.Last but not least, I would like to thank Ameren, St. Louis for theircontinuous support for this thesis project.ii
TABLE OF CONTENTSACKNOWLEDEMENTS . iiABSTRACT . vChapter One Introduction . 1Chapter Two Li-ion Batteries . 102.1 Li-ion batteries and how they work . 102.2 Terms and definitions of Li-ion batteries . 112.2.1 Battery conditions . 112.2.2 Battery specifications . 132.3 Charge and discharge of Li-ion batteries . 152.3.1 Charge process of Li-ion batteries . 152.3.2 Discharge process of Li-ion batteries . 172.4 Li-ion battery chemistries classification . 202.5 Li-ion battery degradation . 222.6 Other features of Li-ion batteries . 232.6.1 Self-discharge . 232.6.2 Recovery effect . 26Chapter Three Lithium Ion Battery Degradation . 273.1 Definition of battery life . 273.2 Li-ion batteries capacity degradation/loss . 283.2.1 Capacity loss overview . 283.2.2 Li-ion battery capacity degradation mechanisms . 303.3 Factors that affect Li-ion battery lifespan . 333.3.1 During storage . 333.3.2 During cycling . 353.4 Methods to prolong the Li-ion battery lifespan . 433.4.1 Cycle and storage environment . 433.4.2 Adding additives . 44Chapter Four Experiment Design . 454.1 Overview . 454.2 Equipment . 464.2.1 INR18650-25R and IMR 18650-HE2 Lithium ion rechargeable cells. 46iii
4.2.2 Synchronous Balance Charger/Discharger iCharger 1010B . 484.2.3 LogView Software . 504.3 Experimental procedures . 504.4 Variables and parameters design . 55Chapter Five Results and Analysis . 575.1.1 Degradation due to cycling effect . 575.1.2 Degradation due to aging effect . 58Chapter Six Conclusions and Recommendations for Future Work . 676.1 Conclusion. 676.2 Suggestions for future work . 69References . 70iv
A STUDY OF THE FACTORS THAT AFFECTLITHIUM ION BATTERY DEGRADATIONShihui XiongDr. Robert O’Connell, Thesis SupervisorABSTRACTSecondary batteries have been applied in every aspect of our life, from cell phones,to laptops, medical devices, satellites, and renewable energy power stations. Li-ionbatteries have shown superior advantages in their ability to store large amount of energy incompact spaces and their long battery life. However, battery capacity degradation, whichcauses battery failure, posts a serious concern to the economy and efficiency to individualsand industries. The degradation rate is affected by several factors including temperature,charge and discharge voltage, current, and the level at which the battery is charged ordischarged. Understanding these factors can largely help to reduce the speed of batteryfailure and allow batteries to better serve their purpose. First, this thesis project did a reviewof existing literatures addressing how conditions including temperature, state of charge,depth of discharge, charge voltage, and C-rate affect Li-ion battery degradation rate andthe conditions necessary to achieve optimal battery life. An experiment was done to studyhow battery chemistry, cycle frequency, and temperature affect battery degradation rateand observe how degradation affects battery performance. Results show that high and lowtemperature shorten battery life, cycle frequency is not consequential with Li-ion batterydegradation rate, and INR batteries might have shorter battery life than IMR batteries.These findings will help consumers and companies better understand proper usage of Liion batteries.v
Chapter OneIntroductionIn the 21st century, due to the public's concern over generating grid electrical powerby burning fossil fuels to avoid air pollution, energy imports, and global warming, therenewable energy industry has emerged and grown rapidly. Renewables have three mainapplications: renewable electricity, renewable heat, and biofuels in road transportation.According to the International Energy Agency, over the next five years, renewables in theelectricity sector will be the fastest growing among these three applications, providingalmost 30% of the power demand in 2023, up from 24% in 2017. During this period, 70%of global electricity generation growth is forecast to be met by renewable energies, withsolar contributing the most, followed by wind, hydropower, and bioenergy [1].Solar and wind energy are frequently used in grid-connected power stations togenerate electricity and reduce the production of carbon. Through power electronicequipment, energy from sun and wind can be transformed into electricity and be fed intothe power grid. One technology, the photovoltaic (PV) system, uses solar panels to collectlight from the sun and to convert it to direct current (DC) electricity. Another one isconcentrated solar power, which uses mirrors and lenses to collect heat from the sun andthen use the heat to drive generator systems. Although both methods have their advantagesand disadvantages, PV systems are far more popular than concentrators. Utility-scale solarcan be achieved by large-scale PV systems which are designed to supply utility level powerto the electric grid. Most solar parks are developed at a scale of at least 1 megawatt-peak(MWP). Until 2018, the world’s largest PV power stations operated over 1 gigawatt1[2].
Electricity generated by solar panels is fed into the power grid through solar inverters, apower conditioning unit, and grid connection equipment. Wind farms consist of manyindividual wind turbines, which are connected through capacitors, resistors or convertersto the electric transmission network. Blades of a wind turbine capture the kinetic energy inwind, and rotate. The rotor passes the energy through a shaft to a generator, which turnsthe rotational energy into electricity. The capacitors, resistors, and converters connected tothe wind turbines compensate the consumption of reactive power and control the speed ofthe wind turbine, which adjusts the voltage to match the grid level.However, when energy from renewable resources are passed to the grid, it causesproblems such as lower power quality, issues with voltage regulation, and many protectionrelated challenges, such as islanding. To be more specific, the traditional grid operatesunder one-way flow. When the electricity generated by the renewable power sources isinjected into the grid, the voltage increases, sometimes over the acceptable range (5%). Inaddition, renewables like solar and wind are intermittent, which cause fluctuations in thegenerated energy. Voltage fluctuations in the grid require frequent adjusting by voltageregulators and can cause voltage flicker. Moreover, without proper storage, generatedelectricity can be wasted during the low energy demand time and might be unavailablewhen needed. Thus, technology is needed to improve the energy quality and efficiency ofrenewable power stations.With the ability to improve the operating capacities of the electric gird through peakshaving and frequency control, battery energy storage systems (BESS) play an importantrole in integrating more variable renewable resources as well as enhancing the reliabilityof the power system. BESS consist of batteries, hybrid inverters, and battery management2
systems (BMS). In grid scale, hundreds and thousands of cells are connected in series andparallel to form battery modules for energy storage systems of megawatt level substations.The BESS are co-located with renewable energy plants, either to smooth the powersupplied by the intermittent wind or solar output, or to shift the power output into otherhours of the day when the renewable plant cannot produce power directly. Thesegeneration-plus-storage systems can either reduce the pressure on the grid when connectingrenewable sources or be used to work off the grid.Figure 1.1 System configuration of grid-connected PV with BESS [30]Figure 1.1 shows one system configuration of grid-connected PV with BESS [30].The BESS is interfaced to a DC bus via a DC/DC buck/boost converter. The PV arrays isinterfaced to the DC bus via a DC/DC buck converter with a maximum power point tracker(MPPT). DC/AC bi-directional converters with voltage source converters (VSC) are usedfor grid interfacing. The utility grid is ideally assumed to be an infinite AC system in thisconfiguration.BESS have gained increasing interest for various grid applications as they reduceintermittency of renewable energy, bring flexibility to power transmission and distribution,3
adjust power peaking, and restructure power markets. BESS is superior to most otherenergy storage techniques because of its fast response time, high efficiency, low selfdischarge, and scaling feasibility. The capacity of BESS in stationary applications isprojected to grow in the next decade from 11GWh in 2017 to between 100GWh and167GWh in 2030 [3].Among all the factors that can impact the efficiency and cost of the grid BESS, thebattery type plays an important role. When choosing the most suitable batteries for anenergy storage system, specifications including specific energy, or capacity that relates toruntime; specific power, or the ability to deliver high current; life-span reflecting cycle lifeand longevity; safety; performance at hot and cold temperatures; and cost all need to beconsidered. Other important factors are toxicity, fast-charge capabilities, self-discharge,and shelf life.There are four main types of batteries for grid-connected renewable energy storage:lead-acid batteries, sodium-sulfur (NaS) batteries, vanadium redox (VRB) batteries, andlithium-ion batteries. Each of these has reached a certain level of maturity in stationaryenergy storage applications. Table 1.1 compares these batteries in terms of their electricalspecifications, reliability, and cost.4
CompositionShelflife/usablelifeNaS15 years, grid-scale batterystorage10 years of commercialoperation.VRB20--30 yearsAdvantagesLong discharge time;Require minimalmaintenance;High energy density;Environmentally friendly.Chargecycles4500Store and release energythrough a reversibleelectrochemical reaction. 10,000Li-ion2-3 yearsLead-acid10 yearsHigh energy;Low self-discharging;High resiliency againstdeep and fast discharge;Low maintenance.Reliable and cheap;Long discharge time.200-3007000 before theyexperience loss.EfficiencyDrawbacks89-92%Need to be operated above300C temp;Material is metallic sodium,which is hazardous when incontact with water;Extra cost to preventleakage.75-80%Complicated system;Requirements of pumps,sensors, flow and powermanagement.TemperatureCost (dollar/kwhr) 300 C-5-50 C500300-50090%Require protection frombeing over charged anddischarged;Li-ion batteries age percharge or discharge. 80%Require highmaintenance; Deepdischarge significantlydamages lead acidbatteries; Short cyclelife.average 209186Table 1.1 Comparison of VRB batteries, NaS batteries, Lithium-ion batteries and Lead-acid batteriesWhile each type of battery has its own advantages and disadvantages, the NaSbattery is most suitable for peak shaving, transmission, and distribution networkmanagement and load-leveling; the VRB battery is best used in high capacity powersystems with a range of 100kW to 10MW; and both the Li-ion battery and the lead acidbattery are suitable in intermittent source power storage in renewable energy systems.Thus, more comparisons can be made between the Li-ion battery and the lead acidbattery. According to O’Connor [4], in 2016, lithium-ion batteries were just beginning to beused for large-scale solar power systems. Recently, lead-acid battery power grid energystorage systems are being replaced with Li-ion batteries. Li-ion batteries are superior to leadacid batteries for many reasons. First, Li-ion batteries have higher energy density, whichenables manufacturers to store high levels of power in compact spaces. A typical lithiumion battery can store 150 watt-hours of electricity in 1 kilogram of battery, while a lead5
acid battery can store only 25 watt-hours per kilogram [4]. In addition, Li-ion batteries havesuperior resiliency that allows for deep and fast discharge without being damaged. Li-ionbatteries can be discharged to about 80% State of Charge (SOC) and at a rate of C/2 (moreon that in Chapter 2) without any long-term damage, while lead acid batteries lose potentialcycles if they are discharged below 50% of their SOC or if discharged faster than C/8[4].What’s more, when cycle life is considered, Li-ion batteries lower the cost in the long termas they require lower cost per cycle. According to Table 1.2 [4], which compares two typesof lead acid batteries with one type of Li-ion battery, even though Li-ion batteries requirea higher initial price, because of their superior cycle life, low maintenance, deep dischargeand high energy efficiency, their price per cycle ( 0.19) is lower than the price of most leadacid batteries per cycle ( 0.71). Even though the flooded lead acid battery cost slightly lessper cycle than the Li-ion battery, it requires regular maintenance; provides significantlyless load power and specific energy; and takes four times longer to charge than the Li-ionbatteries. Thus in conclusion, for the highest cost-saving and superior performancepurposes, the Li-ion battery is most suitable for intermittent source power storage due toits long lifecycle, high energy density, low cost per cycle, and deep and fast dischargeability.6
Table 1.2 Cost comparison of flooded lead acid batteries, VRLA AGM batteries and Li-on batteries [4]Li-ion batteries are extremely popular worldwide in renewable energy BESSapplication because of their superior characteristics. The 36 MW BESS of Younicos’ 153MW Notrees wind farm in Texas, which originally used lead-acid technology, replaced thelead acid batteries with Li-ion batteries in 2017. According to Froese[5], the new Li-ionbattery system will increase the duration of discharge and have the capability to provideadditional fast-responding services. Other Li-ion battery BESS examples include the 32MWh BESS in the Tehachapi 4,500 MW Wind Energy Storage Project in California, US;The 32 MWh Ruien Energy storage project in Ruien, Belgium; The 4.3 MWh Alata projectin Corsica, France; The 10 MWh Smarter Network Storage project in England, UK; The 8MWh Laurel Mountain project in West Virginia, US; The 6.6 MWh Angamos project inMejillones, Chile; The 2 MWh BESS in Johnson City, US; and the three National Wind7
and Solar Energy Storage and Transmission Demonstration Projects in Hebei, China, withcapacities of 36 MWh, 16 MWh and 9 MWh.Meanwhile, more cost-effective Li-ion battery technologies are starting to appear.A lot of companies, including Tesla, LG, Sonnen, Simpliphi Power, and Lithionics all havereliable products at reasonable prices. In 2018, Tesla's Model 3 battery pack costs 190 perkWh. General Motors' 2017 Chevrolet Bolt battery pack is estimated to cost about 205 perkWh[6]. Predictions indicate that the record for solar-plus-storage deployments in 2018will be broken yet again in 2019, which is based on the forecast of falling solar and batteryprices as well as a continuation of the federal Investment Tax Credit for solar that caninclude the cost of batteries as part of the installation[7]. In addition, Schmidt presents amodel to estimate lifetime costs for 9 storage technologies in 12 applications from 2015 to2050 [8], and concluded that Li-ion batteries will be the most competitive technology in themajority of applications in the coming decades.Because of the high popularity of Li-ion batteries among grid-connected BESSapplications, I decided to focus my research on this spectacular battery. However, even withall those superior specifications mentioned above, Li-ion batteries still have a fewdrawbacks, such as self-discharging and aging, which shortens their lifespan. The purposeof my research is to study the factors that impact the Li-ion battery life, reasons behind them,and how to better prevent them in order to prolong Li-ion battery life and achieve betterprofits. My experiment is mainly focused on Li-ion battery degradation under deepdischarge ( 80%), extreme temperature, and the difference between two types of popularLi-ion chemistries.8
In Chapter 2, an overview of the features of Li-ion batteries will be introduced,including different types of Li-ion batteries and their applications, battery charge anddischarge mechanisms, and some terms and definitions related to batteries.Chapter 3 will explain the Li-ion battery degradation mechanism and introducefactors that affect the degradation process.In Chapter 4, an experiment with the purpose of observing the degradation patternof a Li-ion battery under various conditions will be described. The experiment was doneby repeating and recording data from charging and discharging several Samsung 2500mAhINR 18650 and LG 2500mAh IMR18650 Li-ion batteries under different temperatures andfrequencies. Information regarding the lab equipment, experiment steps, recorded valuesand variables will be described in detail.Chapter 5 contains the results of the experiment by plotting the cycle data ofdifferent sets of Li-ion batteries and discussing the analysis of the experimental data.Results show that cycling and aging cause Li-ion battery capacity degradation; high or lowtemperatures accelerate Li-ion battery degradation rate; cycle frequency does not affectbattery life; the INR battery sometimes degrades faster than the IMR battery under thesame cycling environment; and battery degradation shortens the battery discharge time andreduce the available energy and power.Conclusions and suggestions for further study are provided in Chapter 6.9
Chapter TwoLi-ion Batteries2.1 Li-ion batteries and how they workA Li-ion battery is a type of rechargeable battery that consists of a lithiumcompound cathode, a graphite (generally) anode, and electrolyte. Lithium ions move fromthe cathode to the anode when charging and move in the opposite way when discharging.Figure 2.1 is the demonstration of internal reactions and current change during batterycharge and discharge [31]. While charging, lithium compounds break into lithium ions,electrons, and other lithium compounds. Electrons go from the cathode to the anodethrough external conductors, lithium ions move from cathode to anode through theelectrolyte, and current goes from the anode to the cathode.Figure 2.1 Charge and discharge mechanism of li-ion batteries [31]10
Graphite is used in most Li-ion batteries as the anode because it has an intercalatedstructure to hold the lithium ions and increase battery capacity. The lithium ions andelectrons react with carbon on the anode and form a carbon lithium compound. Equation2.1 shows the chemical reactions inside Li-ion batteries when being charged. The processis reversed during discharge.Cathode/ Positive:LiXXO2 Li1-x XXO2 Li xe-Anode/ Negative:C xLi xe- LixCOverall:LiXXO2 C LixC Li1-xXXO2XX means various combining elements of the battery cathode material.Equation 2.1 Chemical reactions during charging li-ion batteries2.2 Terms and definitions of Li-ion batteriesBelow are definitions of the vocabulary used to describe the battery conditions,including C-rate, state of charge (SOC), depth of discharge (DOD), terminal voltage andopen-circuit voltage; as well as the battery specifications, including cut-off voltage,capacity, cycle life, specific energy, energy density, internal resistance, charge voltage, andcharge current.2.2.1 Battery conditions1) C-rateA C-rate is a measure of the normalization of the discharge current of a batteryagainst its maximum capacity. One C rate means that the discharge current will discharge11
the entire battery in 1 hour. The discharge current equals the C-rate times the maximumcapacity of the battery. For example, for a battery with a capacity of 100 Ah, 1C rate meansthe discharge current is 100 Amps. A 5C rate for the same battery would be 500A anddischarge the entire battery in 0.2 hour. The higher the C-rate, the shorter the dischargetime. A C/2 rate would be 50A discharge current and the discharge time would be 2 hours.2) State of charge (SOC)SOC (%) is the percentage of the present battery capacity over the maximumcapacity.3) Depth of discharge (DOD)DOD (%) is the percentage of battery capacity that has been discharged over themaximum capacity. 80% DOD or more is considered a deep discharge.4) Terminal voltage (V)The voltage between the battery terminals when connected to load. Terminalvoltage changes with battery SOC and charge/discharge current.5) Open-circuit voltage (V)The voltage between the battery terminals when no load is connected. The opencircuit voltage of the battery depends on SOC [9].6) Charge retentionThe remaining battery capacity that is available after the battery has been stored orused for a given amount of time.12
2.2.2 Battery specifications1) Cut-off voltage (V)Cut-off voltage is the lowest allowable voltage, at which the battery is considered“empty”. Some electronic devices powered by Li-ion batteries, such as cell phones andlaptops, will automatically shut down when the cut-off voltage (3V/cell) has been reached.2) Capacity (Ah)The battery capacity, also known as the coulometric capacity, is the total Amphours (Ah) available when the battery is discharged at a certain C-rate from 100 percentSOC (full) to the cut-off voltage (empty). Capacity can be calculated by multiplying thedischarge current by the discharge time to estimate the battery’s status. Capacity is used asa threshold for retiring batteries. Manufacturers suggest replacement of batteries whenbattery depth of charge drops to 80% of their maximum capacity.3) Cycle lifeCycle life is the number of discharge and charge cycles that the battery canexperience before it fails to meet specific performance criteria. The actual operating life ofa battery is affected by several factors, including C-rate, DOD, temperature, load size, andso on (more on that in Chapter 4).13
4) Specific energy (Wh/kg)Specific energy, also known as the gravimetric energy density, is the nominalbattery energy per unit mass. Along with energy consumption in different applications, itdetermines the weight of the battery required to achieve a certain total energy.5) Energy density (Wh/L)Energy density, also known as the volumetric energy density, is the nominal batteryenergy per unit volume. Along with energy consumption of different applications, itdetermines the size of the battery required to achieve a certain total energy.6) Internal resistance (IR) (Ω)The resistance within the battery. It is usually different when charging anddischarging, and it depends on the battery SOC. The higher the internal resistance, thelower the battery efficiency.7) Charge current (A)The constant current that charges the battery and increases the voltage (to about 70%SOC) before transitioning into constant voltage charging [9].8) Charge voltage (V)The voltage the battery is charged to when full capacity is reached. The chargevoltage is generally reached when the battery is charged to about 70% SOC by the chargecurrent, and then becomes stable until the charge process ends.14
2.3 Charge and discharge of Li-ion batteries2.3.1 Charge process of Li-ion batteriesFigure 2.2 shows the voltage, current and capacity change during charge of Li-ionbatteries [10]. In the beginning of the charge, the charge current is constant while the batteryvoltage increases until reaching a peak voltage. Then the battery enters saturation charge,during which the voltage stays the same while the current starts decreasing until the chargeis terminated when the current reaches 3% of rated current. Then the charge process ends,and the battery is considered fully charged. Some low-consumer users stop the charging assoon as the battery voltage reaches cut-off (constant) voltage to save time. The chargedbattery reaches about 85% SOC. Otherwise the capacity keeps increasing until thetermination.Figure 2.2 Voltage/capacity/current vs. time when charging Li-ion batteries [10]The charging process is shown with more details in Figure 2.3. Stage 1 is theconstant current charge stage; Stage 2 is the saturation charge, at the end of which the15
battery finishes charging. During Stage 3, which is after the charge ends, the battery voltagebegins to drop because the battery tends to release its stress. A Li-ion battery that has beencharged longer in saturation stage can keep the high voltage for a longer period of timethan one that has not. The open circuit voltage of a Li-ion battery will eventually settle tobetween 3.70V and 3.90V [10].Figure 2.3 Charge stages of lithium-ion [10]A higher charge current doesn’t necessarily fasten the charging process becauseeven though it accelerates the voltage increase to the peak voltage, it slows down thesaturation charge process. Higher charge rate can quickly fill a battery to about 70 percent.Extremely fast charge can cause serious damage to the battery, cause capacity loss, andlead to safety issues. The advised charge rate of an Energy Cell is between 0.5C and 1C.Battery manufactures recommend a charge rate of 0.8C or below to prolong battery life [10].16
Li-ion batteries with cathode materials combining cobalt, manganese, nickel andaluminum typically can be charged to 4.20V/cell with a tolerance of /- 50mV/cell. Somenickel-based Li-ion batteries can only be charged to 4.10V/cell while some high capacityones may go to 4.3V/cell or higher[10]. Overcharge Li-ion batteries can cause overheat,extreme damage, or even catch on fi
life/usable life 15 years, grid-scale battery storage 10 years of commercial operation. 20--30 years 2-3 years 10 years Advantages Long discharge time; Require minimal maintenance; High energy density; Environmentally friendly. Store and release energy through a reversible electrochemical reaction. High energy; Low self-discharging;