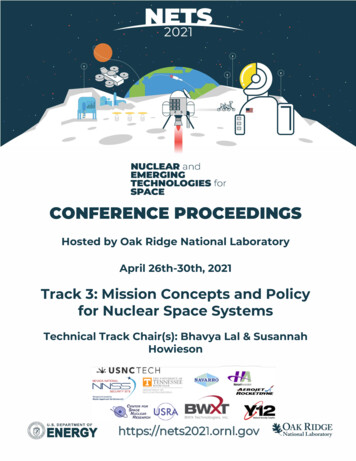
Transcription
NUCLEAR andEMERGINGTECHNOLOGIES forSPACECONFERENCE PROCEEDINGSHosted by Oak Ridge National LaboratoryApril 26th-30th, 2021Track 3: Mission Concepts and Policyfor Nuclear Space SystemsTechnical Track Chair(s): Bhavya Lal & SusannahHowiesonU.S. DEPARTMENT OFENERGYhttps://nets2021.ornl.gov
AuthorKokan, TimothyKumar, SorajKuperman, Alan J.Reynolds, Christopher B.Smith, ClaytonTitleIntroduction to a Human Mars Campaign Utilizing Nuclear Thermal PropulsionDecoding Mission Design Problem for NTP Systems for Outer Planet Robotic MissionsAvoiding HEU In Space Reactors: An Emerging ConsensusModeling of NTP Engine Start-Up, Shutdown, and Cooldown and their Impact on ΔVThe Case for a 50 Year Radioisotope Power System
INTRODUCTION TO A HUMAN MARS CAMPAIGN UTILIZING NUCLEAR THERMAL PROPULSIONTimothy Kokan 1, James Horton2, C. Russell Joyner II3, Daniel J.H. Levack2, Dennis E. Morris 2,Brian J. Muzek1, Rodney W. Noble 2, and Christopher B. Reynolds11Aerojet Rocketdyne, Huntsville, Alabama 35806, USA,Aerojet Rocketdyne, Canoga Park, California 91309, USA,3Aerojet Rocketdyne, West Palm Beach, Florida 33410, USA,2256-922-2579; timothy.kokan@rocket.comNuclear thermal propulsion (NTP) has beenextensively researched as a potential main propulsionoption for human Mars missions. NTP’s combination ofhigh thrust and high fuel efficiency makes it an ideal mainpropulsion candidate for these types of missions, providingarchitectural benefits including smaller transportationsystem masses, reduced trip times, increased abortcapabilities, and the potential for transportationinfrastructure reuse.LH2 MAV MEO MOI MSFC MTV NASA NRHO NTP OMS RCS SLS SNP SPS SOI STMD TDM TEI TMI V Since 2016, Aerojet Rocketdyne (AR) has beenworking with NASA and members of industry as part of theNASA Space Technology Mission Directorate. The initialgoal of this project was to determine the feasibility andaffordability of a low enriched uranium (LEU)-based NTPengine with solid cost and schedule confidence. Havingshown feasibility and affordability, the current projectfocus is on maturing NTP fuel and reactor technology.As part of this activity, AR has examined potentialNTP vehicle configurations to support both short-stay andlong-stay crewed Mars missions and potential vehiclesynergy to support both mission types.Liquid HydrogenMars Ascent VehicleMedium Earth OrbitMars Orbit InsertionMarshall Space Flight CenterMars Transfer VehicleNational Aeronautics and Space AdministrationNear Rectilinear Halo OrbitNuclear Thermal PropulsionOrbital Maneuvering SystemReaction Control SystemSpace Launch SystemSpace Nuclear PropulsionSurface Power SystemSphere of InfluenceSpace Technology Mission DirectorateTechnology Demonstration MissionsTrans-Earth InjectionTrans-Mars InjectionDelta-V, Velocity changeI. INTRODUCTIONThis paper presents an overview of an envisionedhuman Mars exploration campaign consisting of uncreweddemonstration flights, crewed short-stay missions, andcrewed long-stay missions preparing for the eventualpermanent human presence on the surface of Mars.Since 2016, AR has been working with NASA, theDepartment of Energy, and members of industry as part ofthe NASA Space Technology Mission Directorate(STMD)1,2. Initially under the Nuclear Thermal PropulsionProject within the Game Changing Development (GCD)Program, the goal was to determine the feasibility andaffordability of a low enriched uranium (LEU)-based NTPengine with solid cost and schedule confidence.NOMENCLATUREAR Aerojet RocketdyneCFM Cryogenic Fluid ManagementCLV Commercial Launch VehicleCONOPS Concept of OperationsDSH Deep Space HabitatDSM Deep Space ManeuverEME Earth-Mars-EarthEMVE Earth-Mars-Venus-EarthEOI Earth Orbital InsertionEVA Extra-Vehicular ActivityEVME Earth-Venus-Mars-EarthGCD Game Changing DevelopmentGRC Glenn Research CenterIsp Specific ImpulseLDHEO Lunar Distance High Earth OrbitLEU Low Enriched UraniumHaving shown feasibility and affordability, the projectfocus has evolved as part of the STMD Space NuclearPropulsion (SNP) Project within the TechnologyDemonstration Missions (TDM) Program. The currentfocus of the SNP Project is in three major areas:1. NTP fuel and reactor technology maturation;2. Identification of nuclear electric propulsion(NEP) subsystem capability gaps and needs;3. Advancement of critical cryogenic fluidmanagement (CFM) technologies needed forSNP.1
As part of this activity, AR has examined potentialNTP vehicle configurations to support both short-stay andlong-stay crewed Mars missions and potential vehiclesynergy to support both mission types3,4. A natural followon to this work is the definition of a multi-mission humanMars campaign utilizing NTP. An overview of a potentialcampaign is provided in the following section.vehicle systems, and deep space habitat on a dress rehearsalfor follow-on crewed missions.The first crewed mission to the Martian surface woulduse a short-stay opposition class trajectory and establish aminimal set of surface infrastructure to support a 30-dayMars surface stay.Following the initial crewed surface mission,subsequent missions would transition to long-stayconjunction class trajectories . These trajectories takeadvantage of optimal Earth-Mars planetary alignments toallow for a simpler Mars Transfer Vehicle (MTV) and alonger surface stay time of 400 days. These long-staymissions would build on previous infrastructure,establishing a more permanent presence in the form of anoutpost station. Following the first long-stay surfacemission, both the tempo of the missions and the massdelivered to the surface of Mars would increase until adesired steady cadence of surface missions is reached.II. HUMAN MARS CAMPAIGN OVERVIEWTable I provides a sequence of missions for a potentialhuman Mars campaign starting in the early 2030’s withinitial uncrewed flight demonstration missions, followedby a crewed short-stay Mars mission, and finally to crewedlong-stay Mars missions in the 2040’s preparing for theeventual permanent human presence on the surface ofMars.The first uncrewed missions in the envisionedcampaign would be used to test out the NTP system,TABLE I. Human Mars Campaign Mission SequenceMission Name#1a: Cislunar UncrewedFlight Demonstration#1b: Mars Uncrewed FlightDemonstration#2: Mars Crewed ShortStay Mission – 30-DayCrew Surface Stay#3: Mars Crewed LongStay Mission – 400 DayCrew Surface Stay#4 : Mars Crewed SteadyState Long Stay MissionsYear(EarthPrimary Mission GoalDeparture)Test NTP crew vehicle2032in cislunar spaceTest NTP crew vehicle2033on full Mars missionMission Details2-burn mission from MEO to LDHEOMars Opposition Class Mission – flyby or 50 dayMars SOI2037First humans on thesurface of MarsMars Opposition Class Mission – 50 day MarsSOI, 30 day Mars surface2041First human extendedMars surface stayMars Conjunction Class Mission – 400 dayMars SOI and Mars surface2045 Prepare for permanenthuman presence on thesurface of Mars.Series of Mars Conjunction Class Missions –400 day Mars SOI and Mars surface stays.Mission frequency is initially every otherconjunction opportunity (52 months), transitionsto every conjunction opportunity (26 months)Figure 1 provides the required roundtrip mission inspace V throughout the first 20 years of the envisionedcampaign 5 . Lines for four different mission types areprovided: opposition class Earth-Mars-Venus-Earth(EMVE), opposition class Earth-Venus-Mars-Earth(EVME), opposition class Earth-Mars-Earth (EME), andconjunction class EME.The campaign missions enumerated in Table I arehighlighted in Figure 1 as the optimal departure dates inorder to minimize round-trip V for each Earth-Marsmission opportunity selected. As can be seen in Figure 1,the initial short-stay opposition class missions requirehigher in-space V. This higher required V results in alarger, more complex MTV, but allows for shorter overallmissions, benefiting crew health and mission reliability,and limiting the amount of surface infrastructure needed tosupport the crew while on the surface of Mars.The following sections provide an overview of each ofthe missions within the envisioned campaign.2
Earth-Mars Roundtrip V vs. Departure DateLDHEO Earth Departure, 5-Sol Mars Orbit, LDHEO Earth ArrivalMission #4Mission #1Mission #2Mission #3Fig. 1. Roundtrip Mission V for Mars Conjunction and Opposition Missions from 2030-2050then returns back to Earth orbit. Upon return to Earth orbit,the MTV is inspected, refurbished as appropriate, and thepropellant tanks refueled (LH2 for main propulsion andnitrogen tetroxide / hydrazine for reaction control system(RCS) propulsion) for Mission #2.II.A. Mission #1a: Cislunar Uncrewed Flight DemoThe first mission in the envisioned campaign startswith an uncrewed flight demonstration in cislunar space in2032. The mission would start with the aggregation of theMTV in a medium Earth orbit (MEO) with a perigee of2,000 km and an apogee of 6,200 km. This orbit is chosento be above any high density orbital debris fields while alsoaligning launch vehicle payload mass and payload fairingvolume capabilities.After aggregation is completed in MEO, the MTVwould perform a two-burn transfer to a lunar distance highEarth orbit (LDHEO). The MTV would loiter in LDHEOfor a period of 3-6 months to allow for checkout of thevehicle performance and subsystem operation and toprepare for Mission #1b.Missions #1a and #1b provide an uncrewed test of theMTV through the full in-space mission concept ofoperations (CONOPS) to be used in Mission #2.II.C. Mission #2: Mars Crewed Short Stay Mission –30-Day Crew Surface StayThe second mission in the envisioned campaign is thefirst human mission to Mars. The MTV departs MEO withthe first Mars crew in 2037 for a round-trip short-stay Marsopposition mission. Prior to the 2037 crew departure, acargo mission in 2035 pre-deploys the required Marssurface assets to support the crew surface mission. Theseassets include a Mars Ascent Vehicle (MAV), MarsSurface Power System (SPS), and pressurized Mars surfacetransportation / habitat.II.B. Mission #1b: Mars Uncrewed Flight DemoThe second phase of Mission #1 is a round-trip shortstay Mars opposition mission which begins in 2033 afterthe 3-6 month LDHEO checkout period is completed. TheMTV travels to Mars, stays in Mars orbit for 50 days, and3
After the Mars surface assets are pre-deployed, theMTV with crew travels to Mars, descends to the Marssurface for a 30-day surface stay, returns back to the MTVin Mars orbit, and then returns back to Earth orbit. Uponreturn to Earth orbit, the crew rendezvous with a predeployed Orion crew vehicle and returns back to Earth.The MTV is inspected, refurbished as appropriate,elements replaced as needed, and propellants refueled forMission #3.Truss element is the structural backbone for the Drop TankStages to attach to. It provides structural rigidity along withfluid, power, and data transfer between the other elementsof the MTV. The Drop Tank Truss is launched in twosegments on two commercial launch vehicle (CLV)launches. The Drop Tank Truss element segments leveragea service module to assist with rendezvous and dockingwith each other and with the next element launched: theInline Stage.DeepSpaceHabitatII.D. Mission #3: Mars Crewed Long Stay Mission –400 Day Crew Surface StayThe third mission in the envisioned campaign is thefirst mission with an extended stay of humans on thesurface of Mars. The CONOPS of Mission #3 are similarto those of Mission #2 with the exception that the missionis a long-stay Mars conjunction class mission with a crewstay on the surface of Mars of over 400 days. Due to thelonger surface stay, additional Mars surface assets beyondanother MAV, including a surface habitat, logisticsmodule, and unpressurized surface transportation, are predeployed at the same Mars surface location as that used inMission #2.These additional Mars surface assets, along with thoseassets deployed in Mission #2, comprise the beginnings ofa Mars surface outpost. These surface assets set the stagefor the future expansion of the Mars surface infrastructure,and corresponding capabilities, in subsequent Marsmissions.Drop TankStages #1-789mDropTankTrussII.E. Mission #4 : Mars Crewed Steady-State LongStay MissionsInlineStageThe fourth mission in the envisioned campaign is thestart of a series of long-stay Mars conjunction classmissions preparing for the eventual permanent humanpresence on the surface of Mars. These missions, each withsimilar CONOPS to Mission #3, would initially land at theMission #2 / #3 landing site to continue to build up theMars surface outpost. Subsequent missions to additionalsurface locations to further explore various areas of interestare envisioned.CoreStageIII. DETAILS OF FIRST CAMPAIGN MISSION:CISLUNAR AND MARS FLIGHTDEMONSTRATION MISSIONSWith an overview of the envisioned human Marscampaign provided in the previous section, details of thefirst mission in the envisioned campaign are provided here.The Mission #1 MTV is shown in Figure 2. It consists of aCore Stage, Inline Stage, Drop Tank Truss with seven DropTank Stages, and a Deep Space Habitat (DSH).Fig. 2. Mars Transport Vehicle for Cislunar and MarsFlight Demonstration MissionsThe Inline Stage is launched on a NASA SpaceLaunch System (SLS) Block 2 launch vehicle to the sameMEO orbit. It uses its on-board storable RCS propulsion torendezvous and dock with the Drop Tank Truss. Aftermating is complete and mechanical, electrical, and fluidconnections are checked out, the next element is launched:the Core Stage.Figure 3 provides the CONOPS details for Mission #1.Aggregation of the MTV occurs in MEO and starts withthe launch of the Drop Tank Truss element. The Drop Tank4
The Core Stage is also launched on a NASA SLSBlock 2 launch vehicle. The Core Stage has two 25 klbfNTP engines. These engines have a nominal specificimpulse (Isp) of 900 sec. For Mission #1, these enginesoperate at an Isp of 873 sec, leaving significant thermalmargin in the reactor core allowing for future reuse. Similarto the Inline Stage, the Core Stage uses its on-board RCSsystem to rendezvous and dock with the rest of the MTV.Once the center stack is mated and checked out, aseries of seven Drop Tank Stages are launched on SpaceXStarship CLVs to the same MEO orbit. Each Drop TankStage uses its inboard RCS system to rendezvous and dockwith the Drop Tank Truss.With the Drop Tank Stages mated and theirconnections checked out, the MTV aggregation iscomplete. The MTV then performs the Mission #1amaneuvers which are two NTP main engine burns to raisethe orbit from MEO to LDHEO. Each of these burnsconsumes enough liquid hydrogen (LH2) to empty the LH2tanks of two Drop Tank Stages. Once those four Drop TankStage LH2 tanks are empty, the stages are jettisoned. Thisresults in the MTV stack having three Drop Tank Stagesremaining on the Drop Tank Truss after insertion inLDHEO.of 3-6 months to allow for checkout of the vehicleperformance and subsystem operation and to prepare forMission #1b.Mission #1b starts with the trans-Mars injection (TMI)maneuver to leave the Earth’s SOI. After TMI, twoadditional Drop Tank Stages are jettisoned, leaving a singleDrop Tank Stage remaining. The MTV then travels for 343days before performing a deep space maneuver (DSM)burn to align the MTV’s trajectory for intercept with Mars.197 days after the DSM, the MTV performs a Marsorbit insertion (MOI) maneuver to insert into a 5-sol Marsorbit. The MTV stays in Mars orbit for 50 days. During thattime, the MTV performs a series of maneuvers using itsorbital maneuvering system (OMS) to reorient the orbit toprepare for Mars departure. While in Mars orbit, the MTValso has opportunities for remote sensing of Mars and itssatellites, the potential deployment of robotic orbital orsurface payloads, and for the examination of MTVperformance and subsystem operation.After 50 days in Mars orbit, the MTV performs thetrans-Earth injection (TEI) maneuver and travels for 170days back to Earth. As the MTV approaches Earth, itperforms an Earth orbit insertion (EOI) maneuver to insertback in to LDHEO. The MTV then performs an OMSmaneuver to lower its orbit to a loiter orbit in preparationfor refueling and transfer back down to MEO forinspection, refurbishment as appropriate, and refueling forMission #2 of the human Mars campaign.Once in LDHEO, the MTV rendezvous and docks withthe DSH which was launched, assembled, and checked outat the NASA Gateway in the lunar near rectilinear haloorbit (NRHO), and transferred down to LDHEO. TheMTV, now with the DSH, loiters in LDHEO for a periodFig. 3. Concept of Operations for Cislunar and Mars Flight Demonstration Missions5
Figure 4 provides details of the MTV for Mission #1.The total round-trip heliocentric mission time for Mission#1 from Earth departure to arrival back at Earth is 760 days.The NTP engines are run at full power for 2.6 hours tocomplete the seven main engine burns. The MTV stack hasa mass of 393 mT upon completion of aggregation in MEO,and a mass of 287 mT at Earth departure.Mission #1 requires the 25 klbf NTP engines tooperate at an Isp of 873 seconds to close the mission. Theseengines have a nominal specific impulse of 900 sec,leaving significant thermal margin in the reactor coreallowing for future reuse.Fig. 4. Mars Transport Vehicle Details for Cislunar and Mars Flight Demonstration MissionsPrograms, and the Department of Energy engineers thatcontinue working NTP for Mars crewed missions and othermissions that can benefit from NTP.IV. CONCLUSIONS AND FUTURE WORKAn overview of an envisioned human Mars campaign,consisting of uncrewed demonstration flights, crewedshort-stay missions, and crewed long-stay missionspreparing for the eventual permanent human presence onthe surface of Mars, was provided. Details of the firstcampaign mission, an uncrewed cislunar and Mars flightdemonstration mission was also provided. Future work willfocus on the refinement of the campaign and detaileddefinition of the remaining crewed campaign missions.ACKNOWLEDGMENTSThe authors would like to acknowledge the support ofNASA Marshall Space Flight Center (MSFC) and GlennResearch Center (GRC), NASA STMD GCD and TDM6
REFERENCES13Levack, D.J.H., Horton, J.F., Jennings, T.R., Joyner,C.R., Kokan, T., Mandel, J.L., Muzek, B.J., Reynolds, C.,and Widman, F.W., “Evolution of Low Enriched UraniumNuclear Thermal Propulsion Vehicle and Engine Design”,AIAA 2019-3943, AIAA Propulsion and Energy Forumand Exposition, Indianapolis, Indiana, August 19-22, 2019.2Joyner, C.R., Eades, M., Horton, J., Jennings, T., Kokan,T., Levack, D.J.H., Muzek, B.J., and Reynolds, C.B.,“LEU NTP Engine System Trades and Missions”, Nuclearand Emerging Technologies for Space, Richland,Washington, February 25-28, 2019.Reynolds, C.B., Joyner, C.R., Kokan, T., Levack, D.J.H.,and Muzek, B.J., “Mars Opposition Missions UsingNuclear Thermal Propulsion”, AIAA 2020-3850, AIAAPropulsion and Energy Forum, Virtual Event, August 2428, 2020.4Reynolds, C.B., Joyner, C.R., Kokan, T., Levack, D.J.H,and Muzek, B.J., “NTP Robustness for Mars Conjunctionand Opposition Class Missions”, AIAA 2020-4124, AIAAASCEND 2020, Virtual Event, November 16-18, 2020.5Muzek, B.J., Horton, J.F., and Joyner, C.R., “MissionAnalysis for Mars Opposition Missions 2033 to 2048”,AAS 20-065, 43rd AAS Annual Guidance and ControlConference, Breckenridge, Colorado, 2020.7
Nuclear and Emerging Technologies for SpaceSponsored by Oak Ridge National Laboratory, April 26th-30th, 2021.Available online at https://nets2021.ornl.govDECODING MISSION DESIGN PROBLEM FOR NTP SYSTEMS FOR OUTER PLANET ROBOTIC MISSIONSSaroj Kumar 1, Dr. L. Dale Thomas1, and Dr. Jason T. Cassibry11University of Alabama in Huntsville, Huntsville, AL, 35899saroj.kumar@uah.eduThis paper discusses the current challenges ofexploration of outer planets and proposes a NuclearThermal Propulsion (NTP) system for future deep spaceexploration missions. The mission design problem withrespect to NTP system is presented where it is proposedthat NTP powered missions need to integrate therequirements and constraints of mission objective,spacecraft design, NTP system design and launch vehiclelimits into a self-consistent model. The paper presents aconceptual mission design to Jupiter based on the missionmodeling techniques in the paper.NOMENCLATUREIMLEO – Initial Mass in Low Earth OrbitJOI – Jupiter Orbit InsertionJPL – Jet Propulsion LaboratoryLH2 – Liquid HydrogenMIPS – Minimally-Intrusive Power generation SystemNASA – National Aeronautics and Space AdministrationNTP – Nuclear Thermal PropulsionRTG – Radioisotope Thermoelectric GeneratorsTCM – Trajectory Correction ManeuverTJI – Trans-Jupiter Injection V – change in velocity, km/sI. INTRODUCTIONThe robotic exploration of our solar system hasexpanded human knowledge on a diverse range of celestialbodies in our planetary system starting with its formationand evolution to search for life. The exploration of outerplanets in particular have made fascinating discoveries ofocean worlds in gas giant and Ice giant systems. Some ofthe successful rendezvous missions over the decades toouter planets include Galileo (Jupiter), Cassini-Huygens(Saturn) and Juno (Jupiter) [1]. NASA is also developingfuture missions such as Europa Clipper (Europa) andDragonfly (Titan) for the exploration of gas giant systems[2]. Table I mentions NASA’s robotic missions to outerplanets with details on trajectory and trip times.Designing missions to outer planets is extremelychallenging. Due to large distances of these planetarybodies, the V required for such missions is very high. Todate, chemical propulsion systems have been the go-tochoice for deep space exploration missions. However, itslow propellant efficiency has also been a challenge towardsdesigning a dedicated mission to Ice giants withoutrequiring a super heavy-lift launch vehicle [3]. Its limited V capability and struggle against trip time and distanceoften necessitates the use of multiple gravity assisttrajectories. The increased trip time due to gravity assisttrajectories is directly proportional to the Phase- E cost ofthe mission lifecycle which can be a significant number forcost capped planetary missions.TABLE I. Rendezvous robotic missions to outer CassiniEuropaClipper*[4]Dragonfly* [5]JupiterJupiterSaturnEuropaV-E-E-G-A2 dv-E-G-AV-V-E-JG-AM-E-G-ATrip e mission currently in development.I.A. Nuclear Thermal PropulsionNuclear Thermal Propulsion (NTP) can be the gamechanging technology for outer planet exploration missions.It’s high specific impulse, over twice that of the bestchemical rocket, combined with high thrust, enablemissions that require high V missions with short triptimes.The fundamental principle of NTP is remarkablysimple when compared with the traditional chemicalpropulsion systems. In NTP system, the heat energy fromfission reactor is transferred to the propellant which is thenejected through a de Laval nozzle. A schematic of a nuclearthermal propulsion engine is shown in Figure 1 below. Thefuel is injected into the reactor core where it is heated totemperatures of about 2,700 K or above and then ejectedvia nozzle [6]. The temperature of the propellant heating isactually limited due to the structural integrity of the NTPengine. A small amount of propellant is also used to run theturbopumps which feed the propellant to the reactor core.Fig. 1. Schematic of an NTP engine [7].
Another advantage of the NTP system is that it can beused for onboard electric power generation. A bi-modalnuclear engine for propulsive thrust and electric power forthe control of the spacecraft would eliminate therequirement of Radioisotope Thermoelectric Generators(RTGs) [8]. Alternatives to bi-modal nuclear engine arealso being explored to use NTP for electrical powergeneration such as Minimally-Intrusive Power generationsystem (MIPS) which can convert thermal energy from thereactor core at idle to an adequate amount of electricity topower the spacecraft, without compromising the reactordesign and without impacting engine performance [9].study, the spacecraft design will be only considered withrespect to its total mass and dimensions. The NTP systemconfiguration is then addressed which is based on theexpendable or non-expendable nature of the mission alongwith V requirements, engine thrust class (15klbf, 25klbf)and LH2 propellant tank sizing. The spacecraft and NTPsystem configuration such as Initial Mass in Low EarthOrbit (IMLEO) and payload fairing encapsulation areevaluated based on launch vehicle limitations. Because thisexercise is multidisciplinary in nature, the issues during thedesign of one system do not exist in isolation, but feed uponother systems as well. This problem is solved iterativelyuntil multiple cross dependent parameters starting fromspacecraft design to NTP systems and launch vehiclerequirements are satisfied.II. MISSION DESIGN FOR NTP SYSTEMSNumerous studies have been conducted towardsdemonstrating the feasibility of NTP powered roboticmissions for deep space exploration [10, 11]. Among manydevelopment challenges such as very high cost and longschedule of completing development, qualification, andproduction of these engines, the NTP systems for sciencemissions have also not been aggressively considered in thepast due to their large mass and inability to launch them ona single launch vehicle [12]. Requiring multiple launchesnot only increases the complexity of in-orbit assembly butalso increases mission costs. The present analysisaddresses the mass and launch vehicle challenges for NTPpowered robotic missions. The graphical representation ofthe mission design problem for NTP systems is shown inFigure 2.III. JUPITER RENDEZVOUS MISSIONWe demonstrate an NTP powered Jupiter rendezvousmission based on the mission design problem presented inthe previous section. The spacecraft design is based onexpendable mission mode which consists of the spacecraftand NTP system. In the expendable mission mode, the NTPsystem is used only during the TJI and TCM and is thenseparated from the spacecraft. The spacecraft’s orbitinsertion over Jupiter is conducted by the its onboardpropulsion system. Therefore, by limiting the use of theNTP systems to TJI and TCM we simplify the cryogenicLH2 storage problem significantly. NTP system parametersfor this study have been referred from Refs. 13, 14 and 15and are mentioned in Table II.TABLE II. NTP system parameters.ParameterThrust (vac.)Specific impulse (vac.)Nozzle area ratioNTP engine T/WLH2 mass flow rateFig. 2. Mission design problem for NTP powered missions.Values15000 lbf900 s300:12.657.56 kg/sEstimating the spacecraft dry mass was the first steptowards determining the configuration and total wet massof spacecraft and NTP system. Referring to JPL studies onfuture mission concept studies to gas giant system (Ref. 16,17), the dry mass of the spacecraft was estimated to be2300kg. This required about 2050kg of chemical storablepropellant for orbit insertion and trajectory correctionmaneuvers during the science mission operations. Based onthe total wet mass estimates of the spacecraft which is4350kg, the NTP system mass were calculated to be ofabout 21.36mT. This included NTP engine mass of 2560kgand LH2 propellant mass if 12650kg. The spacecraft andNTP system mass breakdown are provided in the table III.The problem tackles multiple areas such as spacecraftand NTP system design based on mission objectives. TheNTP system and spacecraft design parameters are alsoevaluated for launch vehicle constraints. This approachmakes sure that the overall design is within the limitsspecified by each system. The problem statement beginswith the science mission objectives which determineswhether a mission will be a flyby, rendezvous or roundtrip.This information is used towards the spacecraft and NTPsystem design. Based on the payload requirements thespacecrafts subsystems are designed and evaluated tocheck if the overall design meets multiple parameters suchas dry mass, power, and thermal etc. For the scope of this2
TABLE III. Spacecraft and NTP system mass breakdown.VehicleNTP EngineTank dry massSpacecraft wet massLH2 propellant (with 3% ullage volume)Total ‘wet’ mass at launchMass (kg)2,5602,2004,35012,65021,760Fig. 4. E-J direct transfer trajectoryAt the completion of JOI burn, the final captured orbitaround the Jupiter has a period of 48.5 days witheccentricity of 0.96. The final spacecraft mass delivered toJupiter is 3169 kg which includes about 869kg of fuel fororbit corrections during science phase of the mission. Theconverged trajectory shows that the trip time for thedesigned mission is 2.1 years using single high-classcommercial launcher. This demonstrates that the NTPpowered mission can reduce the trip time by a factor of twoor more for similar class of spacecraft. Further, the directtransfer capability also increases the launch window formissions when compared with traditional chemicalpowered missions which have to be dependent on planetaryalignments for trajectories requiring multiple planetaryflybys.The total NTP injection stage length is 16.53m whichincludes 6.63m long NTP engine, 9.4m long LH2 tank andinterstage between NTP engine and LH2 tank of about 0.5mlong. The dimensions of the spacecraft is about 3.5m x3.5m along with 0.5m long interstage between LH2 tankand payload. This makes the total length of the spacecraftto be of about 20.53 m which meets the launch vehiclerequirements with respect to IMLEO and payload fairinglimits for ULA’s Vulcan Heavy and Blue Origin’s NewGlenn [18, 19].IV. CONCLUSIONThe paper addresses the mission design problem bydemonstrating the capability of NTP system for Jupiterrendezvous mission.The integrated system designincludes spacecraft and NTP system which should meet therequirements of launch vehicle and missio
human Mars campaign starting in the early 2030’s with initial uncrewed flight demonstration missions, followed . opposition class Earth-Mars-Venus-Earth (EMVE), opposition class Earth-Venus-Mars-Earth . stay on the surface of Mars of over 400 days. Due to the longer surface sta