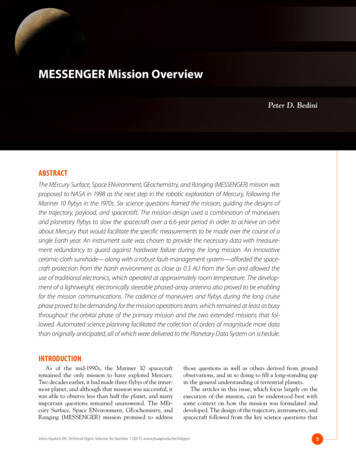
Transcription
MESSENGER Mission OverviewMESSENGER Mission OverviewPeter D. BediniABSTRACTThe MErcury Surface, Space ENvironment, GEochemistry, and Ranging (MESSENGER) mission wasproposed to NASA in 1998 as the next step in the robotic exploration of Mercury, following theMariner 10 flybys in the 1970s. Six science questions framed the mission, guiding the designs ofthe trajectory, payload, and spacecraft. The mission design used a combination of maneuversand planetary flybys to slow the spacecraft over a 6.6-year period in order to achieve an orbitabout Mercury that would facilitate the specific measurements to be made over the course of asingle Earth year. An instrument suite was chosen to provide the necessary data with measurement redundancy to guard against hardware failure during the long mission. An innovativeceramic-cloth sunshade—along with a robust fault-management system—afforded the spacecraft protection from the harsh environment as close as 0.3 AU from the Sun and allowed theuse of traditional electronics, which operated at approximately room temperature. The development of a lightweight, electronically steerable phased-array antenna also proved to be enablingfor the mission communications. The cadence of maneuvers and flybys during the long cruisephase proved to be demanding for the mission operations team, which remained at least as busythroughout the orbital phase of the primary mission and the two extended missions that followed. Automated science planning facilitated the collection of orders of magnitude more datathan originally anticipated, all of which were delivered to the Planetary Data System on schedule.INTRODUCTIONAs of the mid-1990s, the Mariner 10 spacecraftremained the only mission to have explored Mercury.Two decades earlier, it had made three flybys of the innermost planet, and although that mission was successful, itwas able to observe less than half the planet, and manyimportant questions remained unanswered. The MErcury Surface, Space ENvironment, GEochemistry, andRanging (MESSENGER) mission promised to addressthose questions as well as others derived from groundobservations, and in so doing to fill a long-standing gapin the general understanding of terrestrial planets.The articles in this issue, which focus largely on theexecution of the mission, can be understood best withsome context on how the mission was formulated anddeveloped. The design of the trajectory, instruments, andspacecraft followed from the key science questions thatJohns Hopkins APL Technical Digest, Volume 34, Number 1 (2017), www.jhuapl.edu/techdigest5
P. D. BediniTable 1. The key science questions that drove the design of MESSENGER’s primary missionGuiding QuestionScience ObjectiveMeasurement Objectives (Instruments)What planetary formationalprocesses led to the high ratioof metal to silicate in Mercury?Map the elemental and min Surface elemental abundances (GRNS and XRS)eralogical composition of Spectral measurements of surface [MASCS (VIRS)]Mercury’s surface Global imaging in color (MDIS wide-angle camera) Targeted high-resolution imaging (MDIS narrow-angle camera)Globally image the surfaceWhat is the geological historyat a resolution of hundreds of Global stereo imaging (MDIS)of Mercury?meters or better Spectral measurements of geological units [MASCS (VIRS)] Northern hemisphere topography (MLA) Mapping of the internal field (MAG)What are the nature and origin Determine the structure ofof Mercury’s magnetic field?the planet’s magnetic field Magnetospheric structure (MAG, EPPS)Measure the libration ampliWhat are the structure and Gravity field, global topography, obliquity, libration amplitudetude and gravitational fieldstate of Mercury’s core?(MLA, RS)structure Composition of polar deposits (GRNS)Determine the composition Polar exosphere [MASCS (UVVS)]What are the radar-reflectiveof the radar-reflective matematerials at Mercury’s poles? Polar ionized species (EPPS)rials at Mercury’s poles Altimetry of polar craters (MLA) Neutral species in exosphere [MASCS (UVVS)]What are the important volaCharacterize exosphere neu Ionized species in magnetosphere (EPPS)tile species and their sourcestrals and accelerated magne Solar wind pickup ions (EPPS)and sinks on and near Mercury? tosphere ions Elemental abundances of surface sources (GRNS, XRS)framed the mission. Subsequent sets of questions guidedthe concept of operations through MESSENGER’s twoextended missions.The best reference for the mission’s science results isMercury: The View After MESSENGER, to be publishedby Cambridge University Press. For more information onthe mission design, spacecraft, and instruments, pleasesee the dedicated articles in Space Science Reviews,Volume 131, 2007.SCIENCE GOALS AND OBJECTIVESin our understanding of Mercury beyond what wouldhave been achieved at the end of the primary mission.Several overarching themes for this first MESSENGERextended mission ensured that the second year of orbitaloperations would not simply be a continuation of those ofthe primary mission. The new themes included operationduring a period of greater solar activity, greater focus onobservations at low spacecraft altitudes, and more varietyof targeted observations. The extended mission enabledclose-in observations of Mercury near a maximum in thesolar cycle. The lower average altitude was accomplishedby decreasing the nominal 12-h orbital period of theprimary mission to 8 h by lowering the apoapsis of theorbit. The greater variety of instruments making targetedobservations was enabled by the fact that the global mapping objectives of the primary mission had been accomplished. The six new, more-focused questions that framedthe first extended mission are shown in Table 2.Similarly, before the completion of this first extendedmission, given the healthy state of the spacecraft and instru-The MESSENGER mission was designed to addresssix key science questions, the answers to which bear notonly on the nature of the planet Mercury, but also moregenerally on the origin and comparative evolution ofthe terrestrial planets as a class. These questions, whichguided the selection of the science payload and missiondesign, are shown in Table 1. The associated science andmeasurement objectives are also presented, along withthe instruments required toachieve them.Table 2. A new set of six science questions guided MESSENGER’s first extended missionBefore MESSENGER’sNo.First Extended Mission (XM1) Science Questionssuccessful completion ofXM1-Q1 What are the sources of Mercury’s surface volatiles?its primary mission, on theXM1-Q2 How late into Mercury’s history did volcanism persist?basis of propellant and powerusage projections, it was proXM1-Q3 How did Mercury’s long-wavelength topography change with time?posed to continue operatingXM1-Q4 What is the origin of localized regions of enhanced exospheric density on Mercury?the spacecraft for an addiXM1-Q5 How does the solar cycle affect Mercury’s exosphere and volatile transport?tional year, which wouldXM1-Q6 What is the origin of Mercury’s energetic electrons?permit a substantial advance6 Johns Hopkins APL Technical Digest, Volume 34, Number 1 (2017), www.jhuapl.edu/techdigest
MESSENGER Mission Overviewment payload and the ampleTable 3. The goals of the second extended mission were captured in seven scienceamount of power margin andquestionsusable propellant remaining,No.Second Extended Mission (XM2) Science Questionsa second extended missionXM2-Q1 What active and recent processes have affected Mercury’s surface?proposal was prepared, thisXM2-Q2 How has the state of stress in Mercury’s crust evolved over time?one for an additional 2 yearsXM2-Q3 How have the compositions of volcanic materials on Mercury evolved over time?of orbital operations. Theseven guiding questions forWhat are the characteristics of volatile emplacement and sequestration inXM2-Q4the second extended misMercury’s north-polar region?sion (Table 3) followed fromXM2-Q5 What are the consequences of precipitating ions and electrons at Mercury?discoveries made during theHow do Mercury’s exosphere and magnetosphere respond to both extreme andfirst, or anticipated specialXM2-Q6 stable solar wind conditions during solar maximum and the declining phase of theaspects of either the timingsolar cycle?of the observations or theWhat novel insights into Mercury’s thermal and crustal evolution can be obtainedgeometry of MESSENGER’sXM2-Q7with high-resolution measurements from low altitudes?orbit during its final 2 yearsin orbit. Characterize the exospheric neutral atoms andThrough the natural evolution of MESSENGER’s orbitaccelerated magnetospheric ionsin response to the gravitational attraction of the Sun,together with an optimized set of orbit-correction maneuThe seven instruments, depicted in Fig. 1, are thevers (OCMs) conducted with MESSENGER’s remainingMercury Dual Imaging System (MDIS), with widepropellant, the spacecraft orbit during its final year feaangle and narrow-angle cameras for imaging Mercury’stured a unique low-altitude campaign, which is describedsurface; the Gamma-Ray and Neutron Spectrometerby O’Shaughnessy et al. in this issue. Observations con(GRNS) and the X-Ray Spectrometer (XRS) for remotetinued at extraordinarily low altitudes until the spacecraftgeochemical mapping; the Magnetometer (MAG) tofinally impacted the planet in April 2015. These lowmeasure the planetary magnetic field; the Mercuryaltitude measurements are unmatched by any mission atLaser Altimeter (MLA) to measure the surface topogMercury, either in the past or planned for the future.raphy and planetary shape; the Mercury AtmosphericSCIENCE PAYLOADMESSENGER carried a lowmass science payload of seveninstruments and a radio science(RS) experiment. The sciencepayload was selected to achievethe following mission measurement objectives:Mercury Atmosphericand Surface r(GRNS/GRS)X-RaySpectrometerSolar Assembly(XRS/SAX)Fast Imaging PlasmaSpectrometer(EPPS/FIPS) Map the elemental and mineralogical composition ofMercury’s surface Globally image the surfaceat a resolution of hundredsof meters or better Determine the structure ofthe planet’s magnetic field Measure the libration amplitude and gravitational fieldstructure Determine the compositionof the radar-reflective materials at Mercury’s polesMercuryLaserAltimeter(MLA)Mercury DualImaging System(MDIS)X-RaySpectrometerMercury Unit (XRS/MXU)Energetic ParticleSpectrometer(EPPS/EPS)Data Processing Magnetometer (MAG)Unit (DPU)(at end of boom, not shown)Neutron Spectrometer(GRNS/NS)Figure 1. The placement of the MESSENGER science payload elements on the spacecraftfacilitated measurements of the planet while the instruments operated behind the sunshade.Johns Hopkins APL Technical Digest, Volume 34, Number 1 (2017), www.jhuapl.edu/techdigest7
P. D. Bediniand Surface Composition Spectrometer (MASCS),combining an Ultraviolet and Visible Spectrometer(UVVS) with a Visible and Infrared Spectrograph(VIRS) to make high-resolution spectral measurementsof the surface and to survey the structure and composition of Mercury’s tenuous neutral exosphere; and anEnergetic Particle and Plasma Spectrometer (EPPS) tocharacterize the charged particle and plasma environment of Mercury.Table 4. Key parameters of the science payload elementsInstrumentMassa (kg)Powerb (W)MDISGRNSXRSMAGMLAMASCSEPPSData processing .747.37.622.56.94.216.46.77.812.384.4a Massincludes mounting hardware and captive thermal controlcomponents. The mass for MDIS includes the calibration target.The MAG mass includes the boom.b Nominal average power consumption per orbit; actual values variedwith instrument operational mode and spacecraft position in orbit.c Powerincludes purge system, payload harnesses, and magneticshielding for the spacecraft reaction wheels.The payload was carefully chosen so that each instrument addressed more than one of the primary missionobjectives, and each objective was addressed by more thanone element of the science payload. This dual complementarity provided for important cross-checks betweensets of observations and ensured that mission sciencerequirements could be met even in the event of problemswith some of the payload elements. Although the instruments were of necessity single-string in design, the dataprocessing unit that controlled them was fully redundant.This redundancy was not ultimately needed, and the payload as a whole remained healthy throughout the mission.Table 4 summarizes the key parameters of the payload.With the exception of an early failure in one of thesubsystems of the Energetic Particle Spectrometer (EPS)in the EPPS instrument, and the expected failure of theGamma-Ray Spectrometer (GRS) cryocooler in theextended mission, the entire payload performed superblythroughout the primary mission year, plus three additional years of extended orbital operations.Although the loss of the EPS time-of-flight subsystemreduced its capability, the sensor was able to make definitive measurements of the energetic particle populationaround Mercury. In particular, it confirmed that thepopulation is primarily energetic electrons rather thanenergetic ions, as reported by Mariner 10, and was alsoable to characterize the distribution of these particlesand contribute to the conclusion that the electrons areaccelerated in the near-tail region of Mercury’s magnetosphere and injected onto closed magnetic field lineson the planet’s nightside.Table 5. The mission science objectives mapped into the design of the MESSENGER orbitMission ObjectivesGlobally image surface at 250-mresolutionDetermine the structure of Mercury’s magnetic fieldSimplify orbital mission operationsto minimize cost and complexityMission Design Requirements (Instrument)Provide 2 Mercury solar days at two geometriesfor stereo image of entire surface; near-polarorbit for full coverage (MDIS)Minimize periapsis altitude; maximize altituderange coverage (MAG)Choose orbit with period of 8, 12, or 24 hMap the elemental and mineralogiMaximize time at low altitudes (GRNS, XRS)cal composition of Mercury’s surfaceMission Design FeaturesOrbital phase of 1 Earth year (13 dayslonger than 2 Mercury solar days) withperiapsis altitude controlled to 200–505 km; 82.5 -inclination initial orbitMercury orbit periapsis altitude from200 to 505 km; apoapsis altitude near15,200 km; orbit period from 11.76 to12.07 hMinimize orbital-phase thrusting events (RS,Measure the libration amplitude and MLA)gravitational field structureOrbit inclination of 82.5 ; latitude of periapsisnear 60 N (MLA, RS)Determine the composition ofradar-reflective materials at Mercury’s polesInitial orbital inclination of 82.5 ; periapsis latitude drifts from 60 N to 74 N; primarily passive momentum management; first orbit-correction V afterOrbit inclination of 82.5 ; latitude of periapsis89 days and then one orbit-correction Vmaintained near 60 N (GRNS, MLA, MASCS, every 44 days for the next 6 monthsEPPS)Characterize exosphere neutrals and Wide altitude range coverage; visibility of atmo- Extensive coverage of magnetosphere;accelerated magnetosphere ionssphere at all lighting conditionsorbit cuts bow shock, magnetopause, andupstream solar wind8 Johns Hopkins APL Technical Digest, Volume 34, Number 1 (2017), www.jhuapl.edu/techdigest
MESSENGER Mission OverviewEarly in the first year of extended operations, theGRS cryocooler failed after 9500 h of trouble-free operation, which was 1500 h longer than its expected lifetime.Although the GRS could no longer measure gammarays, the instrument was repurposed through a softwarerevision and continued to make meaningful contributions to the science campaign. Specifically, the GRS’santi-coincidence shield offered neutron measurementsthat were complementary to those of the Neutron Spectrometer (NS), and because of its location on a differentdeck of the spacecraft than the NS, the measurementswere subject to smaller variability. The effect was toimprove substantially MESSENGER’s ability to characterize neutron populations about Mercury. In addition,the software reconfiguration enabled an improvementof two orders of magnitude in the temporal resolutionof energetic electron events compared to what had beenpossible with the NS alone.All full-mission-success criteria for the primary mission were met before the end of that first year in orbitabout Mercury, and the criteria for both extended missions were similarly met earlier than required.MISSION DESIGNeclipse last no longer than 65 min. This constraint,combined with an objective to avoid complex missionoperations scheduling, led to the choice of 60 N subspacecraft periapsis latitude and a 12-h orbital period forthe initial orbit. Results of design-phase thermal analysishelped determine that the right ascension of the ascending node must lie between 169 and 354 . This requirement effectively placed the spacecraft orbit periapsisnear the day/night terminator or on Mercury’s nightsidewhen Mercury was closest to the Sun. Several scienceobjectives, including determining the geometry of Mercury’s internal magnetic field, mapping the elementaland mineralogical composition of Mercury’s surface, andglobally imaging Mercury’s surface at a 250-m resolution, led to an orbit design that maintained periapsisaltitudes between 200 and 505 km for the first 3 of the4 years in orbit. The mapping of the various primarymission objectives into the initial science orbit design isshown in Table 5.Heliocentric TrajectoryAfter MESSENGER’s successful launch from CapeCanaveral, Florida, on 3 August 2004, the first phaseof its innovative trajectory was a 6.6-year interplanetarycruise that included six planetary flybys—one of Earth,two of Venus, and three of Mercury—as well as 17 maneuvers. This heliocentric trajectory is shown in Fig. 2 alongwith the flyby dates, locations, and closest-approach altitudes. Each flyby brought the spacecraft’s heliocentricAfter a 6.6-year journey through the inner solarsystem in a heliocentric orbit, the MESSENGER spacecraft executed a 15-min maneuver and became the firstspacecraft to orbit Mercury. The orbit achieved hadbeen designed carefully to facilitate science collection whilemaintaining the health of thespacecraft, and MESSENGERremained in a similar orbit forDSM 1the entire 1-year primary mission. During the first extendedmission, the orbit was adjustedto decrease the orbital periodfrom 12 to 8 h, thereby increasEarth at MOIing the amount of data collectedat lower altitudes. After 3 yearsDSM 2in this orbit, a low-altitude camVenus flybys 1 and 2paign was conducted just before(2987 and 338 km altitude)the end of operations. Trajectory planning and executionDSM 3DSM 4are discussed in the article byMcAdams et al. in this issue.DSM 1Orbital Trajectory DesignThe MESSENGER orbitdesign was influenced by a combination of constraints, requirements, and scientific objectives.The spacecraft’s battery capacity dictated that time in cury flyby 3(228 km altitude)Mercury flyby 2(200 km altitude)Mercury flyby 1(202 km altitude)SunMercuryorbitEarth flyby(2347 km altitude)DSM 5DSM 2 DSM 310/24/06VenusMOI V 0.862 km/s6/5/07Venus1/14/08MercuryLaunchC3 16.4 km2/s2DSM 410/6/08MercuryDSM 59/29/09MercuryMOI3/18/11MercuryFigure 2. MESSENGER’s heliocentric trajectory and deep-space maneuver (DSM) locations asseen from above the north ecliptic pole. A timeline at the bottom indicates the relative timingof planetary flybys and DSMs.Johns Hopkins APL Technical Digest, Volume 34, Number 1 (2017), www.jhuapl.edu/techdigest9
P. D. BediniTable 6. Orbit changes resulting from MESSENGER’s planetary flybysEventLaunchEarth flybyVenus flyby 1Venus flyby 2Mercury flyby 1Mercury flyby 2Mercury flyby 3Orbit aboutMercury (goal)Equivalent V(km/s)aLongitude Longitudeof Periheto Goallion ( )( )OrbitInclination ( )Inclination toGoal ( 3618147.00.00.8617777.0––(MOI)a Values apply to the spacecraft’s orbit after completion of the listed event.PerihelionDistance(AU)Distanceto Goal(AU)AphelionDistance(AU)Distanceto 30.1630.100–orbit closer to that of Mercury and lowered the spacesion. The interplanetary cruise phase ended with MOIcraft’s speed relative to Mercury, thereby decreasing theon 18 March 2011 (UTC).amount of spacecraft-originated velocity change ( V)required to insert the probe into the planned initialOrbit about Mercuryscience orbit around Mercury. Table 6 lists the effect ofA maneuver on 18 March 2011 at 00:45:15 UTCeach planetary flyby on key orbital parameters.markedthe beginning of the mission’s orbital phase.Early in the cruise phase, for trajectory-correctionLasting 15min and imparting a V of 862 m/s, themaneuvers (TCMs) 0.85 AU from the Sun, the sunMOImaneuverslowed the spacecraft’s Mercury-relativeshade was pointed away from the Sun so that sunlightvelocitybyusingvariable-direction thrust with thecould help warm fuel tanks and lessen the demand forthrustvectorremainingnearly opposite to the instanpower from the solar panels. For TCMs 0.85 AU fromtaneousspacecraftvelocityvector throughout thethe Sun, when solar power was plentiful, all maneumaneuver.MOIsafelydeliveredthe spacecraft into anvers were performed with the spacecraft sunshadeorbitwitha207-kmperiapsisaltitude,12.07-h orbitalcenter-panel normal direction within 12 of Sun pointperiod,82.5 inclination,and60.0 Nsub-spacecrafting in order to protect the spacecraft bus from directperiapsislatitude.sunlight exposure.Throughout the 4.1 years orbiting Mercury,Five of the 17 maneuvers during the interplanetaryMESSENGER’strajectory was perturbed by severalcruise phase were major course corrections or deepforces,ofwhichsolar gravity and Mercury’s smallspace maneuvers (DSMs), which imparted a total of1040 m/s in V and targeted subsequent planetary flybysgravitational oblateness, J2, were the dominant factors.and insertion into orbit aboutMercury. The remaining TCMsNtotaled 58 m/s and consisted ofOrbit prior to impact30 April 2015small course corrections thatOrbit after MOI55.7 N latitude atsubstantially reduced target18 March 2011lowest altitude60.0 N latitude at lowest altitudeing errors on approach to aplanetary flyby. After December 2007, these small TCMsyears after MOIwere no longer required because Orbit182March2013of the use of MESSENGER’s83.9 N latitude atlowest altitudesolar-panel tilt and sunshadeorientation as solar sail conLast orbittrols. This novel approach,described by Gold et al. in thisXM1 end/XM2 startissue, resulted in precise tarFirst orbitgeting of the second and thirdOrbit 1 year after MOIMercury flybys and MercuryXM1 start18 March 2012orbit insertion (MOI), as well as73.6 N latitude at lowest altitudepropellant savings that enabledFigure 3. MESSENGER’s changing orbit about Mercury.a final 6-week mission exten-10 Johns Hopkins APL Technical Digest, Volume 34, Number 1 (2017), www.jhuapl.edu/techdigest
MESSENGER Mission OverviewFigure 3 shows MESSENGER’s orbit at key times duringaltitudes from 5 to 35 km above Mercury’s terrain beforethe mission.the spacecraft’s inevitable final descent and impact ontoDuring its first year in orbit, MESSENGER performedMercury’s surface on 30 April 2015.six OCMs, counteracting the influence of a variety oftrajectory perturbations—including those attributable to solar gravity, solar radiation pressure, planetarySPACECRAFTradiation pressure, and variations in Mercury’s gravityThe MESSENGER spacecraft design was driven byfield—to maintain the desired periapsis altitude range ofthree critical mission challenges:200–500 km without altering the 12-h period substan1. Achieving orbit about Mercurytially. Beginning 89 days after MOI, the first five OCMswere executed approximately every 44 days, either to2. Surviving in the harsh environment at Mercuryreturn the spacecraft’s periapsis altitude to 200 km orto adjust the orbital period to an average of 12 h. The3. Returning all collected science data to Earthsixth OCM, conducted 88 days after OCM-5, loweredThe intricate trajectory design outlined in the Misperiapsis altitude to 200 km.sionDesign section required a lightweight vehicle withShortly after the start of MESSENGER’s second yearasubstantialamount of propellant to provide the necesin orbit about Mercury (i.e., early in the first extendedsary propulsive V. To save mass, a composite structuremission), on 16 April and 20 April 2012, respectively,was chosen over a less expensive and simpler convenOCM-7 and OCM-8 reduced the spacecraft’s orbitaltional aluminum design. A ceramic-cloth sunshadeperiod from 11.6 to 8 h. This period reduction waseliminated the need for a heavy, complex, active coolsplit between two OCMs to minimize risk and depleteing system. MESSENGER also used a novel high-gainremaining accessible oxidizer. Because of the rotationantenna design that obviated the need for a relativelyof the orbital line of apsides through its northernmostcostly and heavy gimbaled parabolic dish. InstrumentsMercury latitude of 84.1 N 12 days before the 18 Marchwere optimized for mass, resulting in a total payload2013 start of the second extended mission, solar gravitymass of 50 kg. Lightweight, custom-designed titaniumperturbed the orbit in such a way that no OCMs werepropellant tanks allowed for storage of nearly 600 kg ofrequired to maintain the desired periapsis altitude untilliquid propellant. In fact, careful optimization of theMESSENGER’s fourth and final year in orbit, or 1 yearvehicle design resulted in the liquid propellant outinto the second extended mission.weighing the remainder of the vehicle by 90 kg. ThisDuring the mission’s final year, the MESSENGERpropellant, coupled with a bipropellant main engineteam performed four OCMs (OCM-9 to OCM-12)that enabled a low-periapsis-altitude campaign consistand 16 additional monopropellant thrusters, allowed theing of orbits with periapsisaltitudes between 15 andLow-gain antennas200 km. OCM-9 to OCM-11SunshadeBatteryLarge velocityeach targeted times beforeadjust thrusterthe next OCM when periapHelium tanksis altitude settled with littleFront phasedvariation over many orbitsarray/fanbeamto 25 km above the closantennasest terrain feature beneathStartrackersthe spacecraft. OCM-12targeted an extended periodwhen periapsis altitudesettled with little variationover many orbits to 15 kmabove Mercury’s terrain.Back phasedDuring the mission’s finalarray/fanbeam/44 days, MESSENGERlow-gain antennasperformed seven OCMsLaunch vehiclePropellant tank(OCM-13 to -15, -15A, -16adapter(1 of 3)Solar array (back)to -18) as part of a low-periapsis-altitude hover camMagnetometerpaign (see O’Shaughnessyet al., this issue) to maintain Figure 4. This rear view of the spacecraft shows the elements harbored behind the ceramic-clothunprecedented minimum sunshade.Johns Hopkins APL Technical Digest, Volume 34, Number 1 (2017), www.jhuapl.edu/techdigest11
P. D. Bedinispacecraft to complete the necessary 2 km/s of V toachieve and operate from Mercury orbit. Figure 4 showsthe placement of the primary spacecraft components.The primary challenge to operating safely in theMercury environment was dealing with the extremetemperatures. The direct solar intensity at 0.3 AU is 11 times that at Earth, and the heat reflected off theplanet is 4 times the intensity felt at Earth. In response,the physical layout of the MESSENGER spacecraft wasdominated by the large ceramic-cloth sunshade, whichisolated the main spacecraft components and instruments from direct sunlight and high temperatures. Thesunward side of the sunshade routinely experiencedtemperatures in excess of 300 C, while the elementsharbored behind it operated at approximately room temperature. The latter conditions allowed the use of standard electronics, helping to reduce costs and increasereliability. The assembled sunshade is shown during testing at APL in Fig. 5.The sunshade was effective at isolating the bulk of thevehicle from high temperatures, but not all componentscould remain in its shadow. Solar arrays rely on sunlightfor power and, as such, required tolerance of high tem-Figure 5. MESSENGER engineers at APL adjust the ceramic-fabricsunshade in preparation for the spacecraft vibration test.12 peratures to ensure that sufficient power could be produced when in orbit about Mercury. To help managetemperatures, optical solar reflectors covered two-thirdsof the solar-array surface, in order to reflect the bulk ofthe sunlight incident on the panels. The solar arrayswere also mounted on articulated booms that allowedthe solar panel normal to be rotated away from the Sundirection, to further reduce temperatures. This arrangement allowed the arrays to generate sufficient power bypointing directly at the Sun early in the mission, whenthe spacecraft was near Earth. Late in the mission, whenthe spacecraft was in orbit about Mercury, the arrayswere routinely operated 70 from the Sun direction,simultaneously allowing sufficient electrical power production while managing temperatures.The development of an effective sunshade was critical to surviving Mercury’s harsh environment, butensuring continued safe and uninterrupted operationof the spacecraft also required a well-thought-out faultmanagement approach. A simple yet robust strategywas developed to ensure vehicle safety in the face ofonboard faults (e.g., environmental uncertainties and/or component failures). Faults were classified into threecategories: recoverable, serious, or critical. Recoverablefaults were addressed autonomously by the vehicle andallowed uninterrupted science data collection. Seriousfaults caused the vehicle t
Peter D. Bedini ABSTRACT The MErcury Surface, Space ENvironment, GEochemistry, and Ranging (MESSENGER) mission was proposed to NASA in 1998 as the next step in the robotic exploration of Mercury, following the Mariner 10 flybys in the 1970s. Six science questions framed the mission, guidin