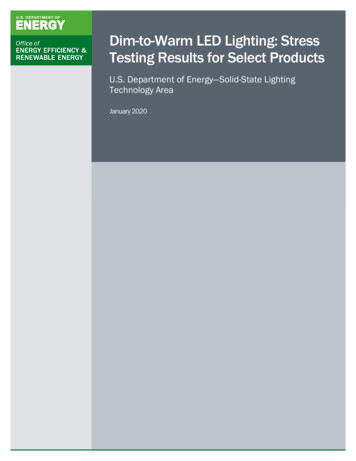
Transcription
Dim-to-Warm LED Lighting: StressTesting Results for Select ProductsU.S. Department of Energy—Solid-State LightingTechnology AreaJanuary 2020
(This page intentionally left blank)
RTI Project Number0215939.001.001Dim-to-Warm LED Lighting:Stress Testing Results forSelect Dim-to-Warm ProductsJanuary 2020Prepared forU.S. Department of EnergyThrough contract withKeyLogic Systems, Inc.3168 Collins Ferry RoadMorgantown, WV 26505Prepared byKelley Rountree, Lynn Davis, Michelle McCombs,Roger Pope, Jean Kim, and Karmann MillsRTI International3040 E. Cornwallis RoadResearch Triangle Park, NC 27709Monica HansenLED Lighting Advisors3047 Marilyn WaySanta Barbara, CA 93105RTI International is a registered trademark and tradename of Research Triangle Institute.iii
Dim-to-Warm LED Lighting: Stress Testing Results for Select ProductsAcknowledgmentsThis material is based upon work supported by the U.S. Department of Energy, Office of Energy Efficiencyand Renewable Energy (EERE), under Award Number DE-FE0025912.This work benefited greatly from input and discussions from members of the LED Systems ReliabilityConsortium: Morgan Pattison of SSLS, Inc, Jim Gaines of Signify, Steve Paolini of Telelumen, Wouter Soerof Lumileds, and Mark Duffy of Current by GE.DisclaimerThis report was prepared as an account of work sponsored by an agency of the United States Government.Neither the United States Government nor any agency thereof, nor any of their employees, makes anywarranty, express or implied, or assumes any legal liability or responsibility for the accuracy, completeness, orusefulness of any information, apparatus, product, or process disclosed, or represents that its use would notinfringe privately owned rights. Reference herein to any specific commercial product, process, or service bytrade name, trademark, manufacturer, or otherwise does not necessarily constitute or imply its endorsement,recommendation, or favoring by the United States Government or any agency thereof. The views and opinionsof authors expressed herein do not necessarily state or reflect those of the United States Government or anyagency thereof.iv
Dim-to-Warm LED Lighting: Stress Testing Results for Select ProductsNomenclature or List of Acronyms45OLoperational life test conducted at 45 C6590life test conducted at 65 C and 90% relative humidityαdecay rate constant in TM-28-14 modelΔuʹchange in the u’ coordinate of chromaticityΔuʹvʹchromaticity shift or the total change in chromaticity coordinatesΔvʹchange in the v’ coordinate of chromaticityλccentroid wavelengthAamperes or ampsacalternating currentANSIAmerican National Standards InstituteASTaccelerated stress testCCTcorrelated color temperatureCIEInternational Commission on IlluminationCOBchip-on-boardCRIcolor rendering indexCSMchromaticity shift modeCSPchip-scale packageD2Wdim-to-warmdcdirect currentDUTdevice under testhrshoursHzHertz or cycles per secondIEEEInstitute of Electrical and Electronics EngineersIESIlluminating Engineering SocietyICintegrated circuitKKelvinL70time required for the luminous flux to decay to 70% of the initial valueLEDlight-emitting diodev
Dim-to-Warm LED Lighting: Stress Testing Results for Select ProductsLFMluminous flux maintenancelmlumenLSRCLED Systems Reliability ConsortiumMP-LEDmid-power LEDNEMANational Electronics Manufacturing AssociationNISTNational Institute of Standards and TechnologyNGLIANext Generation Lighting Industry AlliancenmnanometerPstshort-term flicker indicatorPCBprinted circuit boardRffidelity index in IES TM-30-18Rggamut index in IES TM-30-18RTOLroom temperature operational lifeSPDspectral power distributionSSLsolid-state lightingSVMstroboscopic visibility measurettimeT95time required to reach 95% of equilibrium temperatureTLAtemporal light artifactu'chromaticity coordinate in the CIE 1976 color spaceVvoltv'chromaticity coordinate in the CIE 1976 color spaceWwattWTLwhite-tunable lightingW/nmwatts per nanometervi
Dim-to-Warm LED Lighting: Stress Testing Results for Select ProductsExecutive SummarySolid-state lighting (SSL) products that use light-emitting diodes (LEDs) offer numerous advantages overconventional lighting products including energy savings, longer lifetimes, and greater spectral flexibility of theoutput light from the device. Dim-to-warm (D2W) products are an emerging SSL technology for use inresidential and entertainment applications. D2W products are a type of multi-source LED product and asubclass of white-tunable lighting (WTL) that mimic the behavior of incandescent lamps as they are dimmed;D2W products provide a warmer lighting spectrum (i.e., the correlated color temperature [CCT] shifts to alower value) as the user dims the device. Since the CCT of LEDs does not change drastically with dimming,new electrical architectures and circuits are needed to provide this “warm-dimming” capability in LEDproducts. The effect of these architectures and dimming on the reliability of the D2W lamps and luminaires isnot well understood. Therefore, this report, which is the second report about D2W LED lighting products, usesaccelerated stress tests (AST) to study and understand the mechanisms of optical change of the D2W products.The earlier report (Initial Benchmarks) [1] on D2W products provided initial characterization of the lamps andLED modules studied in this current report. The earlier report also provided performance benchmarks relativeto an incandescent lamp for the lamps studied in this current report.The selected D2W products investigated in this report used different electrical architectures to control power totheir LED primaries, and the electrical architectures could be broadly categorized into two groups(Architecture 1 and Architecture 2). At low power and deep dimming, both architectures only supplied enoughpower to operate the lower CCT LED primary and at moderate dimming levels, the lower CCT LED primaryand at least one or more higher CCT LED primaries emit light. The electrical architectures differed at highpower (e.g., no dimming), where the lower CCT LED primary turned off in Architecture 1 but the lower CCTLED primary (and all other LED primaries) remained on for Architecture 2. The specific devices under test(DUTs) examined in this report include four lamps (referred to as Lamp A – Lamp D) and three integratedLED solutions or LED modules (referred to as Module E – Module G). Lamps A, B, and D used Architecture1 while Lamp C and Modules E – F used Architecture 2 to provide the warm-dimming behavior. In general,the DUTs examined provided spectral tuning in a warm CCT range (1,800 K – 3,000 K); Module G providedspectral tuning in a cooler CCT range (2,700 K – 4,000 K).The four lamps were either A-style (Lamps A and B) or candelabra-style (Lamps C and D). The A-style lampswere 60-watt equivalent and the candelabras were 40-watt equivalent. Both styles were intended for indoor oroutdoor use to promote the familiar warm-dimming characteristics of incandescent lamps. The LED primariesfor each D2W product investigated in this report were unique to each other by way of packaging (plastic midpower LEDs [MP-LEDs] or ceramic MP-LEDs), LED array format (chip-on-board [COB] or chip-scalepackage [CSP]), LED type, phosphor material, and size. Lamps A, B, and D used plastic MP-LEDs whileLamp C used ceramic MP-LEDs. Lamps A, C, and D had traditional LED lamp design, meaning that theirLED packages were mounted to a central printed circuit board (PCB) on a heat sink and the lamp relied onplastic lenses and reflectors for uniform light dispersion. Lamp B used a filament-style LED arrangement witha clear, glass globe, adding to its visual likeness of an incandescent lamp. The LED modules examined in thisstudy were composed of LED arrays in the COB (Module E) or CSP (Modules F and G) format.This report summarizes the overall findings from up to 11,000 hrs of AST on the D2W lamp DUTs and up to7,000 hrs of AST on the LED module DUTs. The AST procedures used in this study included roomtemperature operational life (RTOL) test, 45ºC operational life (45OL) test, and a wet high-temperatureoperational life test performed at 65ºC and 90% relative humidity (6590). During the ASTs described herein,separate populations of each lamp DUT were subjected to power cycling through a four-step process of maxpower (no dimming, 100% power level), low power (dimming the power level to 10% or 25%), medium power(dimming the power level to 50%), and no power (off) during RTOL, 45OL, and 6590 testing. The dwell timeat each power level for the lamp DUTs was determined by the thermal characteristics of each lamp. During theASTs for the LED modules, separate populations of each LED module DUT were subjected to power cyclingvii
Dim-to-Warm LED Lighting: Stress Testing Results for Select Productsthrough a 4-hr loop: 1 hr at max current, 1 hr at low current, 1 hr at moderate current, and 1 hr off duringRTOL, 45OL, and 6590 testing.This report provides details regarding the causes of chromaticity and radiant flux changes and the impacts thatthese changes have on the ability of the DUTs to maintain their designed warm-dimming behavior. This reportprovides key findings on the relative impact of the form factor (i.e., lamp style, COB, CSP); LEDs, includingLED chip, phosphors, and packages; secondary device optics if applicable (e.g., lenses, reflectors); andelectrical architecture on the long-term reliability of the D2W products. The impacts of the new electricalarchitectures and system components on product reliability may be different than other multi-source LED orWTL products, and therefore new challenges to reliability engineering must be identified and characterized tounderstand the performance of these new lighting technologies.The key findings from this report and the earlier report [1] include the following: The reliability of the LEDs for each DUT greatly impacted the long-term chromaticity and radiant fluxbehavior for the D2W products. It was found in this report that the stability of the LED chip wasgenerally good, but the stabilities of the phosphors were less reliable. In particular, the lower CCT LEDprimaries, which had higher red phosphor concentrations, were subject to larger chromaticity shifts in thegreen direction (CSM-2, where CSM stands for chromaticity shift mode). The secondary optics of the lamps in this study were found to have major impact on the chromaticitymaintenance. In particular, the enclosure of LED primaries in a glass globe under positive pressure(Lamp B DUTs) showed great chromaticity stability (Δu'v' 0.002 at all ASTs) relative to the traditionalplastic globes, likely caused by less moisture and air ingress (two major contributors to phosphoroxidation). Additionally, the lenses for Lamps A and C became discolored during AST which led toterminal chromaticity shifts in the yellow-green direction (though parametric failure did not occur). The form factor of the D2W products had significant impact on the reliability of the DUTs examined inthis report. At the more aggressive test conditions (i.e., 6590), the candelabra-style Lamps C and Dperformed the worst, with projected lifetimes to luminous flux maintenance (LFM) values below 0.70 aslow as 8,174 hrs (Lamp C) and abrupt failure occurring for all Lamp D DUTs. The DUTs with A-stylelamp and COB or CSP LED module form factors generally exceeded the maximum allowed lifetimeprojections by the TM-28-14 method. The lower performance of the candelabra-style lamps is thought tobe a consequence of the more stringent space limitations in these DUTs. Aging of the driver electronics for the lamp DUTs examined in this study did not induce large changes inpower consumption, LFM, or temporal light artifacts (TLA), and no correlation between driverarchitecture and device performance was found. However, the driver electronics did impact the overallreliability of the lamps, with four lamp DUTs failing abruptly due to electrical failure and two lampDUTs failing parametrically (a Lamp B DUT exhibited a LFM value below 0.70 while a Lamp C DUTunderwent chromaticity maintenance failure) due to improper regulation of current to the LED primariesby the electrical driver. The parametric failures caused by logic failures are unique to D2W products, andgreater emphasis on understanding the reliability of the logic circuits should be emphasized in futurestudies. The LED modules examined herein were also susceptible to logic failure modes, and intermittent orcomplete failure of these logic circuits produced chromaticity shifts in excess of Δu'v' 0.018 (oneModule E DUT, one Module F DUT, and two Module G DUTs). Three of these DUTs were operated atlow stress test conditions (RTOL or 45OL) and the failures appeared to be caused by manufacturingflaws (e.g., solder migration) or premature component failure as opposed to wear-out mechanisms.These tests show that the D2W products examined in this report can provide a suitable energy-savingsalternative to traditional incandescent lamps. The selected D2W products maintained the general robustnessviii
Dim-to-Warm LED Lighting: Stress Testing Results for Select Productsexpected of SSL products: the first abrupt failure did not occur until 3,500 hrs of operation in the 6590environment and only three parametric failures occurred before 3,500 hrs (there were 63 total DUTs). Inaddition, minimal changes to power consumption and TLA were observed. However, in addition to the failuremechanisms observed for other WTL products (e.g., secondary device optics changes), these tests revealedfailure mechanisms unique to D2W products. Particularly, parametric failures (i.e., chromaticity shift [Δu'v' 0.007], LFM 0.70) were produced by improper current regulation to one or more LED primaries (due tocontrol integrated circuit or logic failure modes) and degradation of red phosphors. As both current control anddeep red phosphors are needed to mimic the deep dimming behavior of an incandescent lamp, this informationis useful to future research aimed at reducing the impact of these failure modes on D2W device reliability.ix
Dim-to-Warm LED Lighting: Stress Testing Results for Select ProductsTable of ContentsExecutive Summary . viiIntroduction.1Experimental and Analytic Methods .4Samples .42.1.1 Lamp A – Lamp D .42.1.2 LED Modules .6Stress Testing Methods.62.2.1 Lamp Power Cycling.72.2.2 LED Module Power Cycling.8Measurement Methods.92.3.1 Luminous Flux .92.3.2 Flicker and Temporal Light Artifacts .92.3.3 Electrical Properties.9Results and Discussion . 10Lamp A – Lamp D . 103.1.1 Luminous Flux Maintenance . 113.1.2 Chromaticity . 153.1.3 Failure Analysis of Lamp A – Lamp D . 193.1.4 Electrical Analysis of Lamp A – Lamp D . 223.1.5 TLA Metrics. 22COB and CSP LED Modules . 253.2.1 Luminous Flux Maintenance of LED Modules. 263.2.2 Chromaticity Shifts of LED Modules . 283.2.3 Electrical Analysis of LED Modules . 30Conclusions . 33Appendix A . A-1Appendix B . B-1x
Dim-to-Warm LED Lighting: Stress Testing Results for Select ProductsList of FiguresFigure 1-1. Schematic showing the common architectures for WTL products and theirsubset D2W products. .1Figure 2-1. The D2W lamps examined during this study: (A) Lamp A, (B), Lamp B, (C), LampC, and (D) Lamp D.4Figure 2-2. The chromaticity points (u’ and v') of Lamps A – D are plotted on the 1976 CIEdiagram. The behavior approximates the black body curve as the lamps are dimmedfrom a starting point of approximately 2700 K to between 1800 – 2200 K, dependingon the product model. The relevant American National Standards Institute (ANSI) colorbins as defined by ANSI C78.377A are provided as a guide.5Figure 2-3. The D2W Integrated LED solutions examined in this study: (A) Module E, (B)Module F, and (C) Module G. .6Figure 2-4. Test configuration for the Module E DUTs examined in this study. .7Figure 2-5. Thermal profile of a Lamp D DUT showing (A) start times (vertical dashed bluelines), T95% (red X’s), time at T95% (vertical dashed red lines), and dwell time necessary toreach T95% (horizontal double-sided black arrows with time labels), and (B) Tstart, Tstable,and T95% for the transition from 25% to 50% dimming. .8Figure 3-1. Initial SPDs of the control DUTs for Lamp A – Lamp D at (A) low power and (B)maximum power. . 10Figure 3-2. Average LFM for the populations of A-style lamps: (A) Lamp A at dimming to10% power, (B) Lamp A at 100% power (i.e., no dimming), (C) Lamp B at dimming to25% power, and (D) Lamp B at 100% power (i.e., no dimming). . 11Figure 3-3. Average LFM for the populations of candelabra-style lamps: (A) Lamp C atdimming to 25% power, (B) Lamp C at 100% power (i.e., no dimming), (C) Lamp D atdimming to 25% power, and (D) Lamp D at 100% power (i.e., no dimming). . 12Figure 3-4. Luminous flux maintenance at low power for the Lamp A DUTs operated at RTOLshow two test populations based on purchase date. DUT 456 and DUT 457 werepurchased at the same time, and DUT 472 and DUT 473 were purchased two weekslater. . 13Figure 3-5. Chromaticity shifts for the A-style lamps in different ASTs: (A) Lamp A at lowpower, (B) Lamp A at maximum power, (C) Lamp B at low power, and (D) Lamp B atmaximum power. . 15Figure 3-6. Chromaticity shifts for the candelabra-style lamps in different ASTs: (A) Lamp Cat low power, (B) Lamp C at maximum power, (C) Lamp D at low power, and (D) Lamp Dat maximum power. The Lamp C DUTs were operated in 6590 test conditions for 8,000hrs. 16Figure 3-7. The centroid wavelengths of the higher CCT LED primary emitters of Lamp Asteadily shifted during 6590 testing: (A) the centroid wavelength of the blue LED shiftedto longer wavelengths and (B) phosphor emission steadily shifted to lower wavelengthsduring the first 3,000 hrs. 17xi
Dim-to-Warm LED Lighting: Stress Testing Results for Select ProductsFigure 3-8. Different degradation pathways likely dominated the emission behavior of theLamp C DUTs throughout testing, which led to varying ratios of blue to red emission atboth (A) low power and (B) max power. . 18Figure 3-9. SPD of Lamp C DUT 489 at 7,000 hrs shows increased radiant flux in the blueregion (450 nm – 500 nm) relative to the starting SPD (0 hrs) and SPD taken at 6,000hrs just prior to parametric failure. . 20Figure 3-10. A representative Lamp D DUT aged at 6590 showing (A) minimal PCBdiscoloration, (B) cracking of the exterior plastics encasing the DUT, and (C)discoloration of the MP-LED terminals relative to the control DUT. 21Figure 3-11. Average (A) power consumption and (B) luminous efficacy for Lamp B DUTs atmax power. 22Figure 3-12. TLA measurements for Lamp A. The control DUT (solid lines) and the averageTLA for DUTs operated in the 6590 conditions after 7,000 hrs (dashed lines) arecompared in the graph and the resulting flicker percentage, flicker index, flickerfrequency, SVM, and Pst are shown in the table. 23Figure 3-13. TLA measurements for Lamp B. The control DUT (solid lines) and the averageTLA for DUTs operated in the 6590 conditions after 7,000 hrs (dashed lines) arecompared in the graph and the resulting flicker percentage, flicker index, flickerfrequency, SVM, and Pst are shown in the table. 24Figure 3-14. TLA measurements for Lamp C. The control DUT (solid lines) and the averageTLA for DUTs operated in the 6590 conditions after 7,000 hrs (dashed lines) arecompared in the graph and the resulting flicker percentage, flicker index, flickerfrequency, SVM, and Pst are shown in the table. 24Figure 3-15. TLA measurements for Lamp D. The control DUT (solid lines) and the averageTLA for DUTs operated in the 6590 conditions after 7,000 hrs (dashed lines) arecompared in the graph and the resulting flicker percentage, flicker index, flickerfrequency, SVM, and Pst are shown in the table. 25Figure 3-16. Initial SPDs of the LED modules at (A) low current and (B) high current. . 26Figure 3-17. Average LFM for (A) Module E at low current, (B) Module E at high current, (C)Module F at low current, (D) Module F at high current, (E) Module G at low current, and(F) Module G at high current. 27Figure 3-18. Chromaticity diagram for (A) Module E at low current, (B) Module E at highcurrent, (C) Module F at low current, (D) Module F at high current, (E) Module G at lowcurrent, and (F) Module G at high current. . 29Figure 3-19. SPD of Module E DUT 487 at 6,000 hrs shows increased radiant flux in theblue-green region (450 nm – 550 nm) relative to the starting SPD (0 hrs) and SPDtaken at 5,000 hrs just prior to parametric failure. Pictures of the DUT show loss of lightemission from LEDs on the left side of the COB at 6,000 hrs. . 31Figure 3-20. (A) SPDs of DUT 589 post-failure (dashed lines) showed increases in blueemissions compared to the SPDs taken at 0 hrs (solid lines) at both low (black) andhigh (red) current settings. (B) The blue box shows an electrical short created betweentwo pins of DUT 591’s control IC. . 32xii
Dim-to-Warm LED Lighting: Stress Testing Results for Select ProductsFigure A-1. Characteristics of Lamp A. . A-1Figure A-2. Characteristics of Lamp B. . A-2Figure A-3. Characteristics of Lamp C. . A-2Figure A-4. Characteristics of Lamp D. . A-3Figure B- 1. Characteristics of Module E. . B-1Figure B- 2. Characteristics of Module F. B-2Figure B- 3. Characteristics of Module G. B-2xiii
Dim-to-Warm LED Lighting: Stress Testing Results for Select ProductsList of TablesTable 2-1. Initial D2W Lamp Performance Characteristics.5Table 2-2. Initial Characteristics of Integrated LED Solutions. .6Table 2-3. Dwell Times at Each Dimming Level for Lamp A – Lamp D and the Total Time ItTakes to Complete the Four-Step Process. .7Table 2-4. Current Levels Used During Power Cycling for the Integrated LED Solutions.9Table 3-1. Centroid Wavelengths of the Blue LED and Phosphors for Lamp A – Lamp D. 10Table 3-2. Experimentally Derived α Values for Lamp A – Lamp D at Maximum Power. 14Table 3-3. Average Time to Reach L70 at Maximum Power for Lamp A – Lamp D. 14Table 3-4. Lamp Failure Descriptions. 19Table 3-5. Power Delivered to the Low-CCT (2,200 K) and High-CCT (2,700 K) LED Primariesof a Lamp D Device (DUT 501) Post Abrupt Failure Compared to the Control Sample. 21Table 3-6. Centroid Wavelengths of the Blue LED and Phosphors for the Integrated LEDSolutions. 26Table 3-7. Experimentally Derived α Values for the LED Modules at High Current. 28Table 3-8. LED Module Failure Descriptions. 30xiv
Dim-to-Warm LED Lighting: Stress Testing Results for Select ProductsIntroductionThe adoption of solid-state lighting (SSL) by commercial and residential users has continued to climb in recentyears. The improved energy efficiency and reliability of SSL devices prompted the transition from traditionallight sources to light-emitting diode (LED) sources. In the United States from 2008 to 2015, the number ofresidential installations of LED A-style lamps (the common “light bulb”) increased from less than 400,000 tomore than 200 million [2]. As LED technology continues to develop, the cost reduction of SSL products andversatility of LEDs promote the adoption of LEDs in many new spaces. The ability to vary or tune the lightspectra from an LED device has made the technology attractive to
Roger Pope, Jean Kim, and Karmann Mills RTI International 3040 E. Cornwallis Road Research Triangle Park, NC 27709 Monica Hansen LED Lighting Advisors 3047 Marilyn Way Santa Barbara, CA 93105 RTI International is a registere