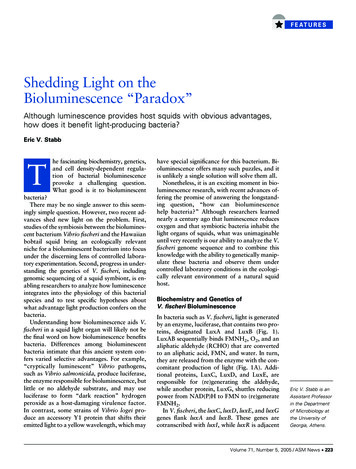
Transcription
Shedding Light on theBioluminescence “Paradox”Although luminescence provides host squids with obvious advantages,how does it benefit light-producing bacteria?Eric V. StabbThe fascinating biochemistry, genetics,and cell density-dependent regulation of bacterial bioluminescenceprovoke a challenging question.What good is it to bioluminescentbacteria?There may be no single answer to this seemingly simple question. However, two recent advances shed new light on the problem. First,studies of the symbiosis between the bioluminescent bacterium Vibrio fischeri and the Hawaiianbobtail squid bring an ecologically relevantniche for a bioluminescent bacterium into focusunder the discerning lens of controlled laboratory experimentation. Second, progress in understanding the genetics of V. fischeri, includinggenomic sequencing of a squid symbiont, is enabling researchers to analyze how luminescenceintegrates into the physiology of this bacterialspecies and to test specific hypotheses aboutwhat advantage light production confers on thebacteria.Understanding how bioluminescence aids V.fischeri in a squid light organ will likely not bethe final word on how bioluminescence benefitsbacteria. Differences among bioluminescentbacteria intimate that this ancient system confers varied selective advantages. For example,“cryptically luminescent” Vibrio pathogens,such as Vibrio salmonicida, produce luciferase,the enzyme responsible for bioluminescence, butlittle or no aldehyde substrate, and may useluciferase to form “dark reaction” hydrogenperoxide as a host-damaging virulence factor.In contrast, some strains of Vibrio logei produce an accessory Y1 protein that shifts theiremitted light to a yellow wavelength, which mayhave special significance for this bacterium. Bioluminescence offers many such puzzles, and itis unlikely a single solution will solve them all.Nonetheless, it is an exciting moment in bioluminescence research, with recent advances offering the promise of answering the longstanding question, “how can bioluminescencehelp bacteria?” Although researchers learnednearly a century ago that luminescence reducesoxygen and that symbiotic bacteria inhabit thelight organs of squids, what was unimaginableuntil very recently is our ability to analyze the V.fischeri genome sequence and to combine thisknowledge with the ability to genetically manipulate these bacteria and observe them undercontrolled laboratory conditions in the ecologically relevant environment of a natural squidhost.Biochemistry and Genetics ofV. fischeri BioluminescenceIn bacteria such as V. fischeri, light is generatedby an enzyme, luciferase, that contains two proteins, designated LuxA and LuxB (Fig. 1).LuxAB sequentially binds FMNH2, O2, and analiphatic aldehyde (RCHO) that are convertedto an aliphatic acid, FMN, and water. In turn,they are released from the enzyme with the concomitant production of light (Fig. 1A). Additional proteins, LuxC, LuxD, and LuxE, areresponsible for (re)generating the aldehyde,while another protein, LuxG, shuttles reducingpower from NAD(P)H to FMN to (re)generateFMNH2.In V. fischeri, the luxC, luxD, luxE, and luxGgenes flank luxA and luxB. These genes arecotranscribed with luxI, while luxR is adjacentEric V. Stabb is anAssistant Professorin the Departmentof Microbiology atthe University ofGeorgia, Athens.Volume 71, Number 5, 2005 / ASM News Y 223
ulated when intracellular AI concentration exceeds a threshold. Thus, light isproduced at high cell densities, such asduring growth in a squid light organ,but not when planktonic cells are growing at low densities.FIGURE 1A Biochemistry and physiologySubstrateoxSubstraterede– transportNAD LuxGH motive forceFMNH2O2NADHH H2OBioluminescence at First AnalysisAppears To Be a Drag on CellEnergyFMNDespite having a good understanding ofthe biochemical and genetic mechanFMNics of bioluminescence, researchers remain uncertain over what its selectiveLuxABLuxAB -FMNH2advantage is to bacteria. In particular,H2Othe apparent costs in energy to cellsO2from bioluminescence make its existence appear paradoxical.LightIn addition to the sizable biosynO2- LuxAB -FMNH2thetic cost in producing the Lux proLuxE LuxCteins, generating light consumes bothRCHORCOOHbiochemical reducing power and oxyNADP gen, seemingly competing for substratesNADPH ATPLuxD AMP PPiwith aerobic respiration, which recoversenergy through electron transport (Fig.O2- LuxAB -FMNH2acyl-ACP1A). Furthermore, energy stored as ATPRCHOis consumed in regenerating the aldehyde substrate (Fig. 1A).B Genetics and quorum sensingIndications that ders cultured cells date at least as farback as E. Newton Harvey’s work atPrinceton University in the early 1900s.Generate lightThose findings were extended by J.Woodland (Woody) Hasting at HarvardOOUniversity and his collaborators, whoshowed that some relatively darker muONtants outgrew their brighter parents. SimHilarly, Paul Dunlap, now at the UniversityOLuxRLuxIof Michigan, characterized bright muAI-dependentAutoinducer (AI)tants of V. fischeri that grew more slowlytranscriptional activatorthan do their relatively dim parent.In 1980, Ken Nealson and David Karl,V. fischeri bioluminescence. (A) Biochemistry and physiology. (B) Genetics and quorumsensing.currently at the University of SouthernCalifornia and the University of Hawaii, respectively, also found that cellsto the other lux genes but not transcribed withexpend appreciable energy for luminescence.them (Fig. 1B). Together LuxI and LuxR underHowever, these two researchers did not find thatlie a well-characterized quorum-sensing regulabioluminescence slows growth rates.tory circuit, in which LuxI generates an autoinThese apparently inconsistent findings are difficult to interpret. Part of the explanation forducer (AI) that interacts with LuxR to stimulatethe inconsistencies is that the best technologylux transcription. The expression of lux is stimLuxAB224 Y ASM News / Volume 71, Number 5, 2005ATPProducts
Stabb’s Science: from Insects and Owls to Bioluminescent Bacteria and SquidsEric Stabb’s parents, both chemistry teachers, encouraged his scientific interests from a very early age.When he became fascinated withinsects at age 8, his father madehim a butterfly net and mountingboard, and supplied him with glassslides, pins, and, soon, his own“knockout” jar plus a supply of carbon tetrachloride. “They wouldn’tlet us use that stuff in college, andI had a bottle at age 10,” Stabbsays. Soon those early insect-oriented interests shifted, and he developed a special liking for owls.He was so analytically minded asa youngster that he collected owlpellets, “looking for jawbones tosee what they had eaten,” he admits. “I’m sure I sometimes leftthem out, but I don’t remembermy mom ever complaining.”Instead, his parents happily supplied him with scientific kits, materials, and other support withoutbeing asked, he recalls. It was as if“all my early grants were funded,except most of the time I didn’teven apply for them,” he says. “Itwas like having a program manager who anticipated what youmight want—and got it for you.”Stabb, 37, now follows the conventional grant application process along with his teaching dutiesin his professional role of assistant professor in the departmentof microbiology at the Universityof Georgia, Athens (UGA). Hisintroduction to microbiologycame pretty much by accident. After he applied for a summer internship to do field biology inAlaska during his sophomore yearat the University of Wisconsin(UW), Madison, “they sent post-cards telling applicants when tobe on hand for a phone interview,”he says. But the postcard arrivedtoo late for him to hold such aninterview, scuttling his chances tospend a summer doing field studies in Alaska.In this case, zoology’s loss wasmicrobiology’s gain. Stabb hadsubmitted what he calls a “fallback” application to a programsponsored by the National Science Foundation to support undergraduate research. He was accepted into this program, run byASM-Carski Distinguished Teaching Award recipient Ken Todar,and worked with Tim Donohuestudying photosynthetic bacteria.“I had no idea what microbiologywas all about,” Stabb recalls. “Igot hooked by what you could doexperimentally with bacteria, especially genetically.” Once hooked,he signed up to do more research,another course, and became “thoroughly sold on prokaryotes.”Stabb and his older brother,who holds a doctorate in appliedphysics and co-owns an engineering consulting company, bothearned nearly straight A’s in theirhigh-school science courses, buteach with one exception. “He gota B in physics, and I got one inbiology,” Stabb says. “There isprobably some deeper significanceto that, but I’m not sure what.”Stabb, who received both hisB.S. and Ph.D. from the University of Wisconsin, is now studyinginteractions between bacteria andtheir hosts, specifically the lightorgan symbiosis between the bacterium Vibrio fischeri and the Hawaiian squid Euprymna scolopes.E. scolopes hatchlings lack symbionts but soon obtain V. fischerifrom their surroundings. Onceinoculated, an individual squidcarries V. fischeri cells in epithelium-lined crypts of a specializedorgan. Light produced by thosebacterial cells helps the squid eludepredators, while the host squidprovides V. fischeri with nutrients.“I think it’s cool,” Stabb says,referring to that symbiosis. “ I’mfascinated by bioluminescence andby the biology of the symbiosis.”It also has a “gee whiz” appeal forstudents, he adds. “This is something students can get fired upabout, and it’s something they canlearn experimental biology with. Idon’t know if my research willhave other practical benefits downthe road—maybe, maybe not. ButI surely hope that some good scientists will get a start here.”Stabb, who grew up in Janesville, Wis., near the Rock River, isan avid runner. He competed inhigh school and college, and stilltrains for regular track sessionswith a group of running buddies.He and his wife Janice Flory, whois a project coordinator for theGeorgia Coastal Research Council, live in a neighborhood nearthe UGA campus. “We both liketo garden, mostly flowers andornamentals,” he says. “Astransplants from the north, weappreciate the longer growingseason here.”Marlene CimonsMarlene Cimons is a freelance writerin Bethesda, Md.Volume 71, Number 5, 2005 / ASM News Y 225
FIGURE 2Squids Provide a Means forScrutinizing Bioluminescenceunder Controlled ConditionsA major advance for bioluminescenceresearch came when Margaret McFallNgai and Edward Ruby, who now areat the University of Wisconsin, Madison, brought the V. fischeri-E. scolopessymbiosis into the laboratory. Although several other hosts of bioluminescent bacteria cannot be bred incaptivity, E. scolopes is an exception.Symbiotic bioluminescence. Light organs of E. scolopes juveniles under white light (leftIn a series of ecological studies, Rubypanel) or lit from within by bioluminescent V. fischeri symbionts (right panel). It is thoughtthat adult E. scolopes use this ventrally directed luminescence to obscure theirand his collaborators found that V. fissilhouette. Solid bars are 100 m.cheri in Hawaii are specifically adaptedto E. scolopes, and they are more abundant in waters inhabited by the host.of the day required comparisons of nonisoLater,Karen Visick at Loyola University ingenic strains, analyses of undefined pleiotroChicago,Ill., provided evidence that bacteriapic mutants, or induction of luminescence byderiveanadvantage from bioluminescence inAI, which also regulates non-lux genes. Rethissymbiosis.Specifically, although a luxA mucently, however, Grzegorz Wegrzyn’s group attantcolonizesE. scolopes, this mutant (unlikethe University of Gdansk in Poland used deitsparentalstrain)does not persist well. Onefined strains to show that luminescence indeedpossibilityisthatthismutant is attenuated beslows the growth of bacteria in cultures. Specauseofdetrimentaleffectsfrom expressing thecifically, he finds that a luxA mutant of V. harfullsetofLuxCDBEGproteinsin the absence ofveyi outcompetes its isogenic parent. SimiafunctionalLuxA.However,wetested an inlarly, we find that a luxCDABEG deletionframe luxCDABEG deletion mutant and camemutant of V. fischeri outcompetes its isogenicup with a result equivalent to Visick’s, namelywild-type parent in mixed culture when AI ispoor persistence of this mutant in the squid.present. Thus, at least under some conditions,Thus, although bioluminescence slows V. fischlight production slows bacterial growth.eri growth in culture, it enhances the bacteriPresumably, however, luminescence confersum’s colonization of the squid light organ. How?an advantage to bacteria in some settings. Forinstance, bioluminescent bacteria naturally inhabit and illuminate the light-emitting organsof animals such as the Hawaiian bobtailedHow Bacteria May Benefitsquid, Euprymna scolopes (Fig. 2). Some biolfrom Being Luminescentogists argue that, in such associations,“what’s good for the host is good for theResearchers have proposed several hypothesessymbiont.” In this specific case, E. scolopesto explain how bioluminescence could conferapparently uses V. fischeri’s luminescence toadvantages to light-producing bacteria (see taelude its predators, suggesting that increasedble). Several of these ideas downgrade the imhost fitness offsets the cost of bioluminescenceportance of luminescence itself, describing it asto the bacteria, because the host “pays” thelittle more than an eye-catching distraction.bacteria back with nutrients.Rather, some researchers argue that the imporEven so, without other constraints, dark mutant event occurs when luciferase consumes oxytants theoretically still should outcompete theirgen or biochemical reducing power within thebright compatriots within a light organ, if formingbacterial cell. It may seem wasteful and thusonly a minority population. However, availablecounterintuitive to burn reducing power andevidence indicates that such dark “cheaters” areoxygen without maximizing proton motivenot found in symbiont populations, suggestingforce and ATP generation; however, this is notluminescence yields additional advantages.unprecedented. Respiratory chains that are rel-226 Y ASM News / Volume 71, Number 5, 2005
atively uncoupled from proton pumpinghelp maintain redox balance, and in inTable 1. How bioluminescence may benefit bacteria directlystances of “respiratory protection” theyPossibleRelevantconsume oxygen rapidly enough to protectbenefitprocessMode of actionoxygen-sensitive cytoplasmic proteins evenSuffocate the O2 consumption Luminescence deprives nearby host epitheliumwhen bacteria are in an aerobic environhostof O2, attenuating reactive oxygen nescencelowers bacterial intracellular O2,2This still leaves open the question ofbreathprotecting O2-sensitive enzymes andwhich is the more important reactant toincreasing resistance to oxidative stress“burn,” oxygen or biochemical reducingRedox sinkNAD Growth conditions lead to buildup of NADH,regenerationand luminescence scavenges O2 to burn thepower? Many researchers believe the releexcess reductantvant reactant is oxygen, although for difDNA repairLight production Luminescence stimulates light-dependentferent reasons. For example, animal-assophotolyase-mediated DNA repair in anotherwise dark environmentciated bioluminescent bacteria mayAvoidLightproductionHostdistinguishes light-producing cells fromconsume oxygen to keep it away from thesanctionsdark ones and punishes the latterhost. Depriving the nearby host epitheliumof oxygen could attenuate the animal’s ability to produce antimicrobial oxygen radiensures that it receives light from the symbiosis.cals, or it could generate a low-oxygen environHow animals could distinguish and selectivelyment that facultative bacterial cells are bettersanction dark bacterial cells that are mixed withsuited to cope with than are obligately aerobicbright ones is not known, but finding cryptohost cells. On the other hand, bioluminescencechromes in the light organ merits further invesmay depress intracellular oxygen concentrationstigation.in the bacteria, either to increase resistance toYet another proposal is that bacterial bioluoxidative stress in general or to protect specificminescence stimulates DNA repair mediated byoxygen-sensitive enzymes. Proponents of thesephotolyase, an enzyme that uses visible-lightexplanations point to the relatively high affinityenergy to fix pyrimidine dimers. According toof luciferase for oxygen as evidence for biolumiWegrzyn and his colleagues, when V. harveyi isnescence driving down oxygen levels.mutated by UV irradiation and then placed inOther scientists believe that the relevant rethe dark, the survival of lux mutants is attenuactant is reducing power. The reducing powerated. Whether this treatment reflects an ecologfor bioluminescence comes indirectly from theically relevant condition is unresolved, but DNANADH pool, and luciferase could thereforerepair by luciferase and photolyase could beserve to recycle NAD cofactor. Jean-Jacquesimportant even if something other than UV lightBourgois and his collaborators at the Universitédamages the cellular DNA.Catholique de Louvain in Belgium recently bolWegrzyn and his collaborators also report astered this argument with evidence that reconnection between the lux system and resisductant flows through luciferase only whentance to oxidative stress, although an alderespiration is saturated. They speculate that luhyde-deficient dark mutant is also resistant.minescence becomes important for symbioticLuciferase without aldehyde catalyzes a “darkbacteria when the other primary electron sink,reaction,” partially reducing oxygen to hydrobiomass production, becomes limited by spatialgen peroxide, which might prime oxidativeconstraints—for example, in the confines of astress responses in this mutant.light organ.Despite such plausible benefits from burningGenetics and Genomics Shedding Lightoxygen or reducing power, the importance ofon Role of Bioluminescence in Bacteriaproducing light itself cannot be dismissed.Cheryl Whistler at the University of New HampGenetic approaches should help us to evaluateshire and Margaret McFall-Ngai propose thatcryptochrome photoreceptors allow squid to dewhich among the proposed beneficial roles oftect bioluminescent symbionts and impose sancbioluminescence are valid. Bioluminescencetions on dark bacteria. This model has evoluhelps V. fischeri to colonize E. scolopes, and thistionary appeal in that it explains how the hostsymbiosis can be manipulated by establishing itVolume 71, Number 5, 2005 / ASM News Y 227
be attenuated in their ability to colonizeE. scolopes. Genome analyses revealone photolyase homologue, and we arenow generating a mutant to test its symbiotic phenotype. Although such questions could be posed before genomicsequence information was available,genomics streamlines the process immensely.Analysis of the V. fischeri genomealso allows us to broadly assess metabolic pathways and to determine howbioluminescence fits into an integratedphysiological matrix. In particular, wecan predict which pathways might complement luminescence under specificscenarios. For example, suppose thatsymbiotic V. fischeri are growing exclusively on peptides and building up excess NADH under low-oxygen conditions, depending on luminescence toscavenge remaining oxygen and regenerate NAD . Under these conditions,how would V. fischeri generate energy?What other pathways would be regenerating NAD ? If this “electron sink”model is correct, such pathways shouldbe expressed during colonization ofthe host, and a lux mutation wouldEnhanced bioluminescence in an arcA mutant of V. fischeri. Culture flasks of wild typestrain ES114 and its arcA mutant illuminated (top panel) or in the dark (bottom panel).make the cells more reliant on otherNAD recycling pathways.Genomic analyses sugges
referring to that symbiosis. “ I’m fascinated by bioluminescence and by the biology of the symbiosis.” Italsohasa“geewhiz”appealfor students, he adds. “This is some-thing students can get fired up about,andit’ssomethingtheycan learn experimental biology with. I don’t k