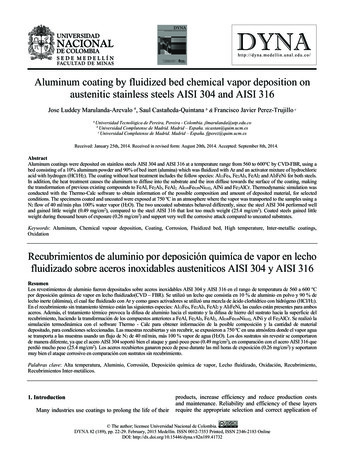
Transcription
Aluminum coating by fluidized bed chemical vapor deposition onaustenitic stainless steels AISI 304 and AISI 316Jose Luddey Marulanda-Arevalo a, Saul Castañeda-Quintana b & Francisco Javier Perez-Trujillo caUniversidad Tecnológica de Pereira, Pereira - Colombia. jlmarulanda@utp.edu.cobUniversidad Complutense de Madrid. Madrid – España. sicastan@quim.ucm.escUniversidad Complutense de Madrid. Madrid – España. fjperez@quim.ucm.esReceived: January 25th, 2014. Received in revised form: August 20th, 2014. Accepted: September 8th, 2014.AbstractAluminum coatings were deposited on stainless steels AISI 304 and AISI 316 at a temperature range from 560 to 600 C by CVD-FBR, using abed consisting of a 10% aluminum powder and 90% of bed inert (alumina) which was fluidized with Ar and an activator mixture of hydrochloricacid with hydrogen (HCl/H2). The coating without heat treatment includes the follow species: Al13Fe4, Fe2Al5, FeAl2 and Al5FeNi for both steels.In addition, the heat treatment causes the aluminum to diffuse into the substrate and the iron diffuse towards the surface of the coating, makingthe transformation of previous existing compounds to FeAl, Fe2Al5, FeAl2, Al0.99Fe0.99Ni0.02, AlNi and Fe2AlCr. Thermodynamic simulation wasconducted with the Thermo-Calc software to obtain information of the possible composition and amount of deposited material, for selectedconditions. The specimens coated and uncoated were exposed at 750 ºC in an atmosphere where the vapor was transported to the samples using aN2 flow of 40 ml/min plus 100% water vapor (H2O). The two uncoated substrates behaved differently, since the steel AISI 304 performed welland gained little weight (0.49 mg/cm2), compared to the steel AISI 316 that lost too much weight (25.4 mg/cm2). Coated steels gained littleweight during thousand hours of exposure (0.26 mg/cm2) and support very well the corrosive attack compared to uncoated substrates.Keywords: Aluminum, Chemical vapour deposition, Coating, Corrosion, Fluidized bed, High temperature, Inter-metallic coatings,OxidationRecubrimientos de aluminio por deposición quimíca de vapor en lechofluidizado sobre aceros inoxidables austeniticos AISI 304 y AISI 316ResumenLos revestimientos de aluminio fueron depositados sobre aceros inoxidables AISI 304 y AISI 316 en el rango de temperatura de 560 a 600 Cpor deposición química de vapor en lecho fluidizado(CVD – FBR). Se utilizó un lecho que consistía en 10 % de aluminio en polvo y 90 % delecho inerte (alúmina), el cual fue fluidizado con Ar y como gases activadores se utilizó una mezcla de ácido clorhídrico con hidrógeno (HCl/H2).En el recubrimiento sin tratamiento térmico están las siguientes especies: Al13Fe4, Fe2Al5, FeAl2 y Al5FeNi, las cuales están presentes para ambosaceros. Además, el tratamiento térmico provoca la difusa de aluminio hacia el sustrato y la difusa de hierro del sustrato hacia la superficie delrecubrimiento, haciendo la transformación de los compuestos anteriores a FeAl, Fe2Al5, FeAl2, Al0.99Fe0.99Ni0.02, AlNi y el Fe2AlCr. Se realizó lasimulación termodinámica con el software Thermo - Calc para obtener información de la posible composición y la cantidad de materialdepositado, para condiciones seleccionadas. Las muestras recubiertas y sin recubrir, se expusieron a 750 ºC en una atmósfera donde el vapor aguase transporta a las muestras usando un flujo de N2 de 40 ml/min, más 100 % vapor de agua (H2O). Los dos sustratos sin revestir se comportaronde manera diferente, ya que el acero AISI 304 soportó bien el ataque y ganó poco peso (0.49 mg/cm2), en comparación con el acero AISI 316 queperdió mucho peso (25.4 mg/cm2). Los aceros recubiertos ganaron poco de peso durante las mil horas de exposición (0.26 mg/cm2) y soportaronmuy bien el ataque corrosivo en comparación con sustratos sin recubrimiento.Palabras clave: Alta temperatura, Aluminio, Corrosión, Deposición química de vapor, Lecho fluidizado, Oxidación, Recubrimiento,Recubrimientos Inter-metálicos.1. IntroductionMany industries use coatings to prolong the life of theirproducts, increase efficiency and reduce production costsand maintenance. Reliability and efficiency of these layersrequire the appropriate selection and correct application of The author; licensee Universidad Nacional de Colombia.DYNA 82 (189), pp. 22-29. February, 2015 Medellín. ISSN 0012-7353 Printed, ISSN 2346-2183 OnlineDOI: http://dx.doi.org/10.15446/dyna.v82n189.41732
Marulanda-Arevalo et al / DYNA 82 (189), pp. 22-29. February, 2015.precursor gases and phases formed at equilibrium at thetemperature range 500 – 660 C provides an understandingof the reactions during the deposition. The schematicdiagram of the CVD-FBR system used in this work isdescribed in [18,19].The reactor was made of quartz tube and was heated byan external furnace. The bed used was composed of a mixof 10 wt% aluminum powder with 99.5% of purity and 400µm particle size as donor, plus 90 wt% alumina (Al2O3) asinert bed. The powder mixture was fluidized by Ar gas(99.999 % purity) with hydrogen chloride plus hydrogen(HCl H2). These gases are used as activators forgenerating aluminum precursor. The chamber reactiontemperature was controlled by a thermocouple introducedinto fluidized bed and the samples were suspended abovethe fluidized bed during the aluminum deposition. The bedwas fluidized with Ar between 300 and 3000 ml/min and asactivator was used a mixture of HCl/H2 at a ratio from 1/15to 1/20. These tests were performed at a temperature rangefrom 560 to 600 C with exposure times from 45 minutes to2.5 hours on different types of austenitic stainless steels.After deposition of the CVD-FBR coatings, a diffusion heattreatment was performed under argon flow at 750 ºC for 2 h,followed by cooling into the furnace under an atmosphere ofAr gas. The morphology study of the aluminum layers wascarried out using metallographic preparation followed byscanning electron microscopy (SEM-JEOL JM-6400). Thecomposition was studied by energy dispersed X-rayspectroscopy (EDAX) and the structure was studied by Xray diffraction (XRD-PHILIPS X (PERT MPD)) throughthe modes θ-2θ and grazing angle in order to identify phasecomposition of coated samples. Standard metallographicpreparation was performed on samples prior to cross-sectionobservations. The specimens were exposed at 750 ºC in anatmosphere where the vapor was transported to the samplesby a flow of N2 of 40 ml*min-1 plus 100% water vapor(H2O). The test was conducted up to 1000 hours, takingsamples at 200, 400, 600, 800 and 1000 hours. After eachexposure, the samples were cooled inside the furnace andthe samples were weighted on an analytical balance withprecision of 10-5g.these coatings for a successful operation. The above wouldincrease the useful life of machine components andfacilities, leading to savings on global consumption ofmaterials [1-3].Coatings obtained by chemical vapor deposition (CVD)are one of the alternatives for surface protection againstcorrosion and wear. CVD consists of the reaction of amixture of gases inside a vacuum chamber to deposit thinlayers of different materials [4, 5]. Inside a reactionchamber, the substrate is exposed to one or more volatileprecursor gases, which in turn react or decompose on thesubstrate surface and deposit a solid coating in a thin layer,and by-products of reaction can produce volatile compoundsthat are removed towards the outside by a flow of gaspassing through the reaction chamber [6,7].Chemical vapor deposition by fluidized bed (CVD-FBR)is a variant of the chemical vapor deposition that combinesthe advantages of thermal activation by heating and thefluidized bed. Advantages of fluidized beds, such as thehigh transfer mass and heat among the gas, the bed and thesamples immersed in the reactor, allowing a more uniformtemperature and very good mixture of reactive gases withfluidized particles. CVD-FBR achieves a high degree ofreaction for all species activated in bed, because of theexcellent contact between the solid particles and thefluidization gas [8-10]. This helps to reduce the temperatureand operation time compared to other processes such aspack cementation [5,9]. In addition, working at atmosphericpressure can save the purchase of expensive vacuumequipment, and cover large parts "in-situ", meaning anadvantage from the economic point of view.The use of aluminum layers is an effective way toincrease corrosion resistance of steels. This protection isobtained by forming a surface film of alumina (Al2O3). Thecoating grows by internal diffusion of aluminum; while ironand chromium diffuse outward [11-13]. The inherentproperty of aluminum to form stable layers of Al2O3 inatmospheres containing oxygen is well known and it hasbeen adapted for surface enrichment of steel componentsthat operate in aggressive environments at high temperature,since alumina has excellent mechanical properties and goodchemical stability at high temperature [14-16].3. Results and discussion2. Experimental procedure3.1. Aluminum coatingsSpecimens (20 mm x 6 mm x 2 mm) of austeniticstainless steels AISI 304 and AISI 316 were used. For thisstudy of aluminum deposition, the specimens weremachined and polished with emery paper from No. 100 toNo. 600 and then cleaned in ultrasonic ketone bath for 10minutes. Finally the aluminum coating was deposited byCVD-FBR. Thermodynamic simulations were performed toinvestigate the better conditions for deposition of aluminumcoatings in CVD-FBR using Thermo Calc software [17].The calculations are based on free Gibbs energyminimization method and mass conversion rule. To definethe gaseous species and solid species, the thermodynamicsimulation was performed using the software Thermo-Calc,combining two databases from Scientific Group Thermodata Europe: SSOL2 and SSUB3. The research of the mainReactions were studied in the aluminum bed and thegases activators HCl H2, to obtain information of thedeposition process of aluminum layers by CVD-FBR. In theresults obtained by the thermodynamics simulation, it wasfound that the precursors Al3Cl and AlCl were formed at thetemperature range from 500 – 660 C. It has also beenobserved that AlCl is the most important precursor in theformation mechanism of the aluminum layer, since Al3Cl isvery stable. Fig. 1a shows the thermodynamic simulation ofaluminum deposition process on AISI 304 stainless steel,and also reports the partial pressures of the gaseous phase asa function of temperature. It is clear that the aluminumdeposition is carried out mainly by the following chloridesAlCl3, AlCl2H, Al2Cl6 and AlCl and in smaller proportion23
Marulanda-Arevalo et al / DYNA 82 (189), pp. 22-29. February, 2015.PARTIAL PRESSURE 0.9939Cr0.0043Al0.0011Mn0.0004 and it is very small, alsodecreases slightly at the whole range of temperature.Fig. 2b shows the simulation thermodynamics of solidphases that might be encountered during the deposition ofaluminum based on the moles of aluminum on austeniticstainless steels. The same solid phases reported inthermodynamic simulation Fig. 2a are present at the beginning ofFig. 2b, namely BCC A2-2, Al2Fe and Al3Ni2. As theconcentration of aluminum moles increases, new solid phasesarise containing higher aluminum content such as Al5Fe2, Al8Cr5,Al3Ni, Al13Fe4, Al9Cr4 and Al13Cr2. BCC A2-2 phase consists ofFe5.01Ni3.83Cr0.55Mo0.008Mn0.004 in the coating on steel AISI 316and in the coating on steel AISI 304 is composed ofFe4.8Ni4Cr0.55Mn0.005.Pre-eliminate tests were conducted in the CVD-FBRreactor using a bed formed by a 10 % aluminum powder and90 % bed inert (alumina), it was fluidized with argon and amixture of gases HCl/H2 as activators. The argon flow wasvaried from 50 % to 80 % and the relation of gas activatorsfrom 1/15 to 1/20. For HCl/H2 relations under 1/20, uniformaluminum coatings were not obtained, since there is only asmall change in tone of the surface of the substrate and also thecross sections reveal "islands" of aluminum coating aftersurface analysis in the microscope. For relations greater than1/16.5 HCl/H2 begins to chlorinate the aluminum coating attemperatures above 580 C. This was done in order to find goodconditions of aluminum deposition on the two stainless steels.AlClAlClH 2AlClH 2520540560580600620640660TEMPERATURE ( C)PARTIAL PRESSURE (PASCALS)a)Formation of aluminum 00Cl2MoCl3CrClCr520540560ClFe5806006206400,6SOLID PHASES (MOLE)TEMPERATURE ( C)b) b) Formation of metal chloridesFigure 1. Thermodynamic simulation of the formation of precursors of thedeposition of aluminum by chemical vapor deposition in Fluidized bed reactors.Source: The Authorsby AlClH2 and AlCl2. The most stable species are AlCl2H andAlCl3. The only species whose partial pressure decreases withincrease of temperature is Al2Cl6 and above 575ºC the partialpressure is lower than that of AlCl. Fig. 1b shows thetemperature versus the partial pressure for chlorination of iron,chromium, nickel, molybdenum and manganese. This graphshows that the thermodynamic tendency of formation for thesechlorides is very low (less than 10-9).According to Fig. 1b, there are no chlorination problemsin the substrate and there should not be corrosive attack byHCl in the process of formation of aluminum coating underthe selected deposition conditions. The reactions offormation of aluminum chlorides are more favored thanchlorination reactions of the substrate. In thethermodynamic simulation were obtained the potential solidphases which are expected to take place during thedeposition of aluminum on both austenitic stainless steels.The simulation was carried out at a temperature range from500 to 660 C as shown in Fig. 2a, where the solid phasespresent in the deposition are BCC A2-2, Al2Fe and Al3Ni2in smaller proportion. The compound BCC A2-2, is formedby Fe0.554 Al0.262 Cr0.162 Mn0.012 Mo0.007. Al2Fe has a largemass decrease at the whole range of temperature. Thegreatest amount of molybdenum is in steel AISI 316 formthephaseBCC A2-1,thisphasecontaining0,5BCC A2-20,4G AS0,30,2Al 2 Fe0,1Al 3 Ni 20,0500BCC A2-1520540560580600620640660TEM PER ATUR E ( C )a) Solid species in function of temperature.Al 2 FeAl 3 N i 2SOLID PHASES (MOLE)Al 5 Fe 20,60,5BCC A2-2Al 13 Fe 4Al 2 FeAl 3 N iAl 13 C r 2Al 9 C r 4Al 8 C r 5F C C A1Al 5 Fe 2Al 13 F e 4BCC A2-20,40,30,20,10,00,0Al 3 N i 2Al 8 C r 5Al 9 C r 4Al 13 C r 2FC C A1Al 3 N i0,10,20,30,40,5M O LE Alb) Solid species in function of aluminum.Figure 2. Thermodynamic simulation of aluminum deposition process onaustenitic stainless steels. CVD-FBR deposition process.Source: The Authors24
Marulanda-Arevalo et al / DYNA 82 (189), pp. 22-29. February, 2015.almost 50 % aluminum, 25 % iron, 12 % chromium, 10 %nickel, 1% manganese and small amounts of molybdenum0.65 % for the AISI 316 stainless steel.The aluminum deposition is achieved with the followingrelations: concentration of active gas HCl/H2 from 1/20 to1/16.5; with gas flows as follows active gas (HCl/H2) from 44% to 34 % and argon from 56 % to 66 %. The best deposits areachieved in the early stages of fluidization of the bed, since ithas a fluid bed with a low flow gas. The best coatings wereobtained with 61% of argon, 37.1% hydrogen and 1.9% ofhydrochloric acid, in bed with 90% alumina and 10% aluminumpowders. Therefore, under these conditions temperature variedfrom 560 to 600 C and deposition times from 45 to 150minutes, to assess how the thickness of the deposited layervaries with the change of these variables.Fig. 3a shows the thickness changes during time ofexposure at 580 C, for specimens coated with andwithout heat treatment. Heat treatment was performed at750 ºC for two hours. Fig. 3b shows the thicknesschange versus exposure temperature for 1.5 hoursdeposition time, for specimens coated with and withoutheat treatment. Heat treatment was performed at 750 ºCfor two hours. The aluminum coating thicknessincreases when increasing temperature up to 580 C.For steel AISI 316, the thickness increase is slowerfrom 580 to 600 C, but for steel AISI 304 thicknessdecreases from 580 to 600 C. In addition, at 600 C isobserved the chlorine peak in EDAX analysis,indicating that the coating is starting to chlorinate; forthis reason the deposition temperature was fixed at 580 C from this point on.The aluminum coating thickness increases with increasedeposition time, in the first 90 minutes in an accelerated way,then the increase in thickness is slower, for this reason thedeposition time was fixed at 90 minutes from this point on, sincethe thickness gain is very small in the remaining 60 minutes ofexposure, thus it is advisable to stop CVD-FBR at this point,because the costs from the use of inert and reactive gases areavoided for 60 minutes of exposure. The coating characteristicslike the thickness and homogeneity depend strongly on the inputratio of active and inert gases (HCl/H2, Ar respectively), as wellas on temperature and time. The thickness of samples iscorrelated to increase of the equilibrium partial pressure ofgaseous precursors, due to the increase of the partial pressure ofprecursors, also cause more interaction of precursors with thesubstrate and thus forming a greater amount of aluminumcoating.Fig. 4 shows the surface of aluminum coatings on AISI 304stainless steels, in which there was a slight conical growth ofthis coating, also it is possible to see that the cones have someflake-like projections. In the early stages of deposition indifferent points of the sample, round nodules are formed, whoseupward growth is faster than their sideways growth, leading tothe formation of these conical forms and as time goes on, thesecones collide with each other. Subsequently they cover thewhole sample surface.Generally, the growth of the layer can be controlled by asurface process reaction-diffusion. The lateral growth ismore difficult on the base of cones, because there is morechromium on the substrate. Keep in mind that chromiumdoes not help the growth of inter-metallic aluminum; so it iseasier that the layer grow upwards than grows sideways.The coatings surface composition analysis by EDAX report:316 Al304 Al316 Al HT304 Al HTTHICKNESS (µ)3530316 Al HT2520304 Al304 Al HT15316 Al1050406080100120140160TIME IN MINUTEa) Deposition temperature at 580ºC.THICKNESS (µ)3228316304316304AlAlAl HTAl HT304 Al HT24316 Al HT20304 Al16316 Al128560570580590600TEMPERATURE ( C)b) Deposition time 1.5 hours.Figure 3. Thickness of aluminum coatings on both stainless steels.Source: The AuthorsFigure 4. Surface of the aluminum coatings on stainless steels AISI 304.Source: The Authors25
Marulanda-Arevalo et al / DYNA 82 (189), pp. 22-29. February, 2015.Concentration (At%)Al80 Ni60CrSubstrateFeConcentration (At%)Aluminum 4SubstrateCr8000Aluminum CoatingNi100481216202428Depth ( m)24c) Stainless steel AISI 316 aluminum coated and without heat treatment.Depth ( m)a) Stainless steel AISI 304 aluminum coated without heat treatment.Concentration (At%)NiFe2Al5FeAl2Concentration (At%)NiFeAl 0405080FeAl CoatingAluminumdiffusion epth ( m)d) Stainless steel AISI 316 aluminum coated and heat treated.Depth ( m)b) Stainless steel AISI 304 aluminum coated and heat treated.Figure 5. Cross section of aluminum coating on the stainless steel AISI 304 and AISI 316.Source: The AuthorsIn the process of aluminum deposition by CVD-FBRhomogeneous coatings were obtained with a variation of /5 microns in thickness. Cross sections presented in Fig. 5,show that the aluminum amount is higher in the coatingsurface and decreases slightly toward the substrate-coatinginterface. The iron and chromium have greater percentages26
Marulanda-Arevalo et al / DYNA 82 (189), pp. 22-29. February, 2015.in the interface coating-substrate than in surface-coating.Nickel is homogeneously distributed through the coating.Heat treatment was conducted in these coatings to improveoxidation resistance. It was observed that aluminum diffusesinto the substrate and the coating has a mixture of Fe2Al5,FeAl2 and FeAl among other compounds, this is shown inanalyses of X-ray diffraction (Fig. 6). Also it is observed azone of FeAl in the interface coating-substrate. Fig. 5 showsthe cross section for these aluminum coatings with andwithout heat treatment, to see how the coating compositionvaries depending on the heat treatment. Non-heat treatedcoating are composed approximately of 67% aluminum,20% iron, 8% chromium and 5% nickel and heat treatedcoating are composed approximately of 50% aluminum,34% iron, 10% chromium and 6% nickel.Fig. 5b shows that aluminum-coated AISI 304 steelpresents four zones: an external rich in FeAl2 and Fe2Al5,with some parts darker than the others, the second area richin FeAl, the third area rich in chromium, containing a bandof Fe2AlCr and finally the area of aluminum diffusion in thesubstrate. Fig. 5d shows that aluminum-coated AISI 316steel presents three zones: an external zone corresponding tothe coating that after heat treatment is formed mainly FeAl,this zone has 18 µm approximately, between the coating andthe substrate there is a chromium-rich band, which shouldcontain Fe2AlCr, even though this band cannot be noticedby naked eye, it was observed in the composition lineanalysis. The other zone is formed by diffusion ofaluminum on the material base, in this area we can see theKirkendal effect [9-19], since there are numerous poresgenerated by inter diffusion of aluminum and iron.In Fig. 6 are showed the analyses of X-ray diffraction(XRD); the coatings without heat treatment are formed byAl13Fe4, Fe2Al5, FeAl2 and Al5FeNi for the two steels. Inaddition, the heat treatment transformed the abovecomponents to form the FeAl, Fe2Al5, Al0.99Fe0.99 Ni0.02,AlNi3 and the Fe2AlCr. This is corroborated with the lineanalysis performed by EDAX to coatings with and withoutheat treatment, besides the heat treatment reduces theamorphous characteristic of the structure of the aluminumcoatings. Heat treatment favored the transformation ofaluminum-rich phases into others with greater iron content,due to inter diffusion of aluminum, iron and chromium. It isclear that aluminum diffuses into the substrate faster thaniron diffuses into the coating, since the diffusion coefficientof aluminum is an order of magnitude greater than that ofthe iron [19]. This aids to improve mechanical propertiesand corrosion resistance of the coating.160HEAT e0.99Ni0.02AlNi3Al5FeNiFe2AlCr400WITHOUT HEAT SITION 2 THETAa) Aluminum coating on AISI 304 stainless steelAl Fe413Fe2Al5800INTENSITYHEAT i3Fe2AlCr200INTENSITY0160WITHOUT HEAT TREATMENT12080400102030405060708090POSITION 2 THETAb) Aluminum coating on AISI 316 stainless steelFigure 6. Analysis of X-ray diffraction for the coatings on AISI 304stainless steel and AISI 316 stainless steel.Source: The Authors.measurements have confirmed that the application ofthese aluminium coatings on austenitic stainless steels byCVD-FBR allows to reduce the oxidation rate more than 80times under super critical conditions. When the corrosiveattack begins, the coated steel gained mass at higher speed,it happens in the first 400 hours and then mass gaindecelerates, because aluminum coating has good resistanceto oxidation in oxidizing environments; because it forms asurface layer of Al2O3, with oxides rich in chromium andnickel, this layer is compact and adherent and protects thecoating from corrosive attack [20,21]. Uncoated steels showa linear attack for the whole time. The corrosion rate of theAISI 304 steel has a tendency to increase over time,whereas the AISI 316 steel has a great weight loss, becausethe oxide layer formed in the early hours grows quickly andthen falls, leaving the substrate exposed to corrosive attackand the corrosion process continues.3.2. Steam oxidationThe specimens were exposed at 750 ºC in a steam loopto assess the degree of protection provided by these coatingsunder supercritical temperature and in an aggressiveenvironment. Both uncoated substrates behaved differently,since the steel AISI 304 performed well and gained lowmass, whereas steel AISI 316 lost too much weight. Coatedsteels gained little weight during the thousand hours ofexposure and are highly resistant to oxidation. Gravimetric27
Marulanda-Arevalo et al / DYNA 82 (189), pp. 22-29. February, 2015.coatings a good alternative to protect steels in super criticalconditions for future applications in power plants.The thermodynamic simulation is a good tool to selectthe working conditions and identify the aluminum halidesperforming the deposition of aluminum. In the simulation ofsolid compounds the only species present in the coating wasFeAl2. Furthermore, it is possible that Cr is present in solidsolution in the intermetallic phases, since the substratecontains Cr and the structures of these intermetallic phasesare not affected by the introduction of Cr [28]. On the otherhand, EDX analysis shows that chromium is also inaluminum coating. In the literature, X-ray diffractionreported that during the deposition of aluminum in stainlesssteels, Cr diffuses readily into the coating to form mainlyCr5Al8 on the outer surface [29], however XRD results ofthis study do not show the formation of this compound.TIME IN HOURS2 m/A (mg/cm )02004006008001000-5-10-15-20-25AISI 316AISI 304AISI 316-ALAISI 304-ALTEMPERATURE 750 Ca) The entire range of mass gain.4. ConclusionsTEMPERATURE 750 C2 m/A (mg/cm )0,50,4 The CVD-FBR is an effective technique to obtain veryuniform and homogeneous aluminum coatings onaustenitic stainless steels at low temperature, short timeand atmospheric pressure; furthermore aluminumcoatings of more than 20 µm of thickness can beobtained. To obtain aluminum coatings of 20 µm the depositionconditions by CVD-FBR were improved at temperaturesbelow 600 C, without affecting the microstructure ofaustenitic stainless steels. Furthermore, the behavior ofthese coatings in steam oxidation conditions issignificantly better than that observed on uncoatedsubstrates. CVD-FBR is an important alternative toimprove steam oxidation resistance of austeniticstainless steels. Yielding great chances to shorten timesand lower costs. The aluminum coating deposited by CVD-FBR withoutheat treatment forms inter metallic compounds with ironsuch as Al13Fe4, Fe2Al5, FeAl2 and Al5FeNi.Subsequently they are transformed by diffusion heattreatment into FeAl, Fe2Al5, Al0.99Fe0.99 Ni0.02, AlNi andFe2AlCr, and as a result oxidation resistance of thesubstrate is improved. Furthermore the coating expandsafter heat treatment due to the inter diffusions of ironand chromium outward and the aluminum into thesubstrate, thereby the coating can reach up to 35 µm ofthickness. Aluminum deposited on the coating form alumina on thesurface that acts as a barrier against oxidation. Therefore, thealuminum coating is able to extend the life of stainless steelAISI 304 and AISI 316 under steam oxidation at 750 ºC.AISI 304AISI 316-ALAISI 304-AL0,30,20,10,02004006008001000TIME IN HOURSb) Mass gain from 0 to 0.5 mg/cm2Figure 7. Mass gain of stainless steel with aluminum coated and uncoatedoxidized in the loop steam at 750 º C.Source: The Authors.During the initial oxidation, elements more active thaniron react and form oxides; as aluminum is the mostreactive, this will be the first to react with oxygen to formAl2O3, followed by the chromium forming Cr2O3, once thealumina layer is formed, the Cr2O3 is dissolved in the layeror evaporates. Moreover the corrosion resistance alsodepends on the thickness of the layer [22,23]. Degradationof the chromite layer by volatile compounds formation takesplace when the chromite layer (Cr2O3) interacts with H)2)(g) or CrO2(OH)(g) [24,25]. Aluminum coatingsare highly protective at high oxidation temperatures, incomparison to uncoated stainless steels substrates becausethe oxidation rate decreases several orders of magnitude, asit is shown in Fig. 7. Aluminum coating does not form theFe2O3 nor Fe3O4, thus the coating prevents the formation ofFeO. The aforementioned iron oxides have a very limitedprotective capacity [26,27]. The results showed thataluminum coating have a beneficial effect in the protectionof austenitic stainless steels in steam oxidizing conditions atsuper critical temperatures (over 700 C), as these coatingssignificantly reduce the corrosion rate, making theseAcknowledgementsThe authors would like to thank DepartamentoAdministrativo de Ciencia, Tecnología e Innovación COLCIENCIAS for his doctoral fellowship andUniversidad Tecnológica de Pereira for the studycommission. The research group would like to thank theSpanish Ministry of Science for its contribution to thispaper: Project MICINN ENE 2008-06755-C02-02 and28
Marulanda-Arevalo et al / DYNA 82 (189), pp. 22-29. February, 2015.CONSOLIDER CSD 2008-00023.5. 0257-8972(99)00305-9[19] Pérez, F.J., Hierro, M.P., Trilleros, J.A., Carpintero, C., Sánchez, L.,Brossard, J.M. and Bolívar, F.J., Iron aluminide coatings on ferriticsteels by CVD-FBR technology, Intermetallics, 14 (7), pp. 811-817,2006. 0] Kobayashi, S. and Yakou T., Control of intermetallic compoundlayers at interface between steel and aluminum by diffusiontreatment, Materials science and engineering: A, 338 (1-2), pp. 4453, 2002. http://dx.doi.org/10.1016/S0921-5093
se transporta a las muestras usando un flujo de N 2 de 40 ml/min, más 100 % vapor de agua (H2O). Los dos sustratos sin revestir se comportaron de manera diferente, ya que el acero AISI 304 soportó bien el ataque y ganó poco peso (0.49 mg/cm2), en comparación con el acero AISI 316 que perdió mucho peso (25.4 mg/cm2).