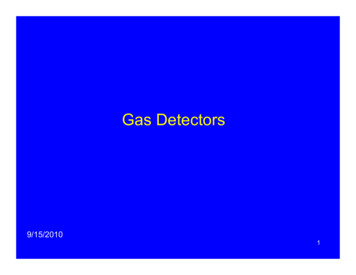
Transcription
Gas Detectors9/15/20101
ContentsIntroductionTypes of Gas DetectorsIonization of the Detector GasElectrodesBasic electrode ConfigurationsParallel Plate ElectrodesCoaxial ElectrodesMovement of Ion Pairs to ElectrodesBeta Particle Interaction with the DetectorAlpha Particle Interaction with the DetectorPhoton Interaction with the DetectorRadiation Type and Size of Detector SignalModes of Operation2
ContentsCurrent Mode of OperationGeneralExamplePulse Mode of OperationPulse Characteristics: Voltage (V)Pulse Characteristics: Current (I)Pulse Characteristics: Charge (Q)Making Pulse Height Related to the Collected ChargePulse ShapePulse Rise and the Maximum Voltage (Vmax)Pulse Tail and the Time Constant (J)3
ContentsThe Six (or five) Region CurveGeneralRecombination RegionIonization RegionProportional RegionRegion of Limited ProportionalityGeiger Mueller regionGeiger-MuellerContinuous DischargeKnow the Difference between These Curves4
Introduction5
IntroductionTypes of Gas DetectorsThere are three types of gas detectors:1. Ionization chamber2. Proportional counter3. Geiger Mueller counter6
IntroductionIonization of the Detector GasThey are referred to as gas detectors because the signal isproduced when charged particles ionize the gas in thedetection chamber.- - - - - - - - - - - - - If the gas is neon (the most common gas in a GM detector),the positive member of each ion pair is a neon ion (Ne )while the negative member of the pair is a freed electron (e-).If the detector gas is air, the positive member of the ion pairis usually a positively charged nitrogen molecule (N2 ) andthe negative member of the ion pair is the freed electron (e-).7
IntroductionIonization of the Detector GasThe term “ion” usually refers to the positive member of thepair. Sometimes the electron is also referred to as an ion.In the absence of an electric field, the ion pairs created in thegas of the detector simply recombinerecombine.However, if the ionization occurs between two oppositelycharged electrodes, and if the electric field is sufficientlystrong, the positive and negative members of the ion pairswill separate and be collected at the electrodes.8
IntroductionElectrodesAn electric field is established by creating an potentialdifference between two electrodes. The potential difference isthe voltage applied across the electrodes.Anode:the positively charged electrodeCathode:the negatively charged electrodeIn most systems, the cathode is grounded. That way anindividual touching the cathode won’t receive a shock. Thecathode is still negative with respect to the anode however.9
IntroductionBasic Electrode ConfigurationsThere are two general electrode configurations:1.Parallel plate electrodes2.Coaxial electrodesVariations on these configurations are common and thesituation in a given instrument might only approximate one ofthem.Calculating the strength of the electric field is straightforwardfor the parallel plate and coaxial configurations, but it can befar more complicated in a real instrument.10
IntroductionParallel Plate ElectrodesParallel plate electrodes are only used in ionizationchambers. Nevertheless, many ion chambers employ acoaxial configuration. Parallel plate configurations are mostcommon for the ion chambers used in radiology.As the name implies, the two electrodes are parallel platesarranged as shown in the following diagram.CathodeAnode 11
IntroductionParallel Plate ElectrodesThe strength of the electric field is:ξ is the strength of the electric field (e.g., volts /meter)V is the potential difference (e.g., volts)d is the distance between the two plates (e.g., meters)The strength of this field is uniform – it is the samethroughout the space between the electrodes.12
IntroductionCoaxial ElectrodesThe coaxial electrode configuration, or a variant thereof, isfar more common than the parallel plate configuration.In the most typical coaxial configuration, the cathode is acylinder that serves as the detector chamber wall. In somecases, the cathode is a conductive coating on the inside ofthe chamber wall.The anode consists of a central wire running that runs alongthe chamber’s axis.13
IntroductionCoaxial Electrodesξ is the strength of the electric field (e.g.,(e g voltsolts /meter)V is the potential difference (e.g., volts)r is the radial distance from the anode center to the point atwhich the electric field strength is calculated (e.g., meters)a is the anode radius (e.g., meters)b is the cathode radius (e.g., meters)14
IntroductionCoaxial ElectrodesCathode(-) rbAnode( )aThe strength of the electric field varies throughout thechamber volume. It increases rapidly as we move towardsthe central electrode and decreases as we move towards the15outer electrode.
IntroductionMovement of Ion Pairs to ElectrodesIf the electric field strength is sufficiently large, the ion pairswon’t recombine. The electrons move to the anode and thepositively charged gas ions move towards the cathode.The drift velocityy is the velocityy at which the ions movetowards the electrodes. It is given by the following formula:ξ is the electric field strength (e.g., V/m)v is the drift velocity (e.g., m/s)u is the mobility factor of the ion (e.g., m2 atm/V s)p is the gas pressure (e.g., atmospheres)16
IntroductionMovement of Ion Pairs to ElectrodesPositively charged gas ions take approximately 10milliseconds to reach the cathode in a typical detector - theirmobility constant is about 1.3 x 10-4 m2 atm/V s. Since themobility constant for electrons is roughly 1000 times larger,electrons reach the anode in microsecondsmicroseconds.As the positive ions and electrons move to the electrodes,they collide with various gas molecules and sometimestransfer their charge to those molecules (depending on thelatter’s electro-negativity). If an electron collides with a highlyelectronegative molecule (e.g., O2), the electron will becaptured and the now negatively charged gas moleculerather than the electron proceeds to the anode.17
IntroductionMovement of Ion Pairs to Electrodes over Time(-)(-)NeNeO2Ne- eO2(-)(-)Ne NeeO2NeNeO 2-( )( )A neon atom is ionized.The neon ion goes to thecathode and the electronheads towards the anode( )( )The electron collides withan O2 molecule and iscaptured. The negative O2ion goes to the anode.
IntroductionBeta Particle Interaction with the DetectorBetaThe average energy lost by a charged particle per ion pairproduced is approximately 34 eV. Since a typicalelectron/beta particle produces 100 ion pairs per cm of travel,a 1 Mev electron might have a range of 300 cm.Given the size of a typical gas detector, beta particles usuallytransfer a small portion of their energy to the detector gas. 19
IntroductionAlpha Particle Interaction with the DetectorAlphaThe average energy lost by a charged particle per ion pairproduced is approximately 34 eV. A typical alpha particleproduces 40,000 ion pairs per cm. As such, a 6 Mev alphaparticle will have a range of about 4.5 cm.Given the size of a typical gas detector, it is possible for somealpha particles to expend all their energy in the detector gas.20
IntroductionPhoton Interaction with the DetectorPhotons might interact directly with the detector gas, but theyare more likely to interact with the detector wall (the cathode).In some of these interactions, an electron is “knocked off” thedetector wall This electron then traverses the gas inside thedetector chamber. This electron might, or might not, have21sufficient energy to cause further ionizations.
IntroductionRadiation Type and the Size of the Detector SignalWith ion chambers and proportional counters, the magnitudeof the detector signal depends on the number of ion pairsproduced in the gas by the incident charged particle.In generalgeneral, but not alwaysalways, the largest to smallest signalwould be produced by:Alpha particles (largest signal)Beta particlesPhotons (smallest signal)22
IntroductionModes of OperationA gas detector can operate in one of two modes:1. Current Mode2 Pulse mode2.Ion chambers usually operate in the current mode becausetheir pulses are too small to be easily distinguished fromelectronic noise.Proportional counters and Geiger Mueller detectors usuallyoperate in the pulse mode.23
Current Mode ofOperation24
Current Mode of OperationGeneralIn the current mode, the system measures the current fromthe detector chamber rather than count the pulses.The detector calibration relates the current to a quantity suchas the exposure rate (e(e.g.,g R/hr)R/hr).Current (I) is measured in units of amperes (A).1 ampere 1 coulomb per secondThe current from a radiation detector is very small, often onthe order of picoamperes (pA).25
Current Mode of OperationGeneralCurrent is charge per unit time, e.g., coulombs per secondI Q/tI is current (amperes)Q is the collected charge (coulombs)t is the time (seconds)Q nen is the number of collected electronse is the charge on each electron (1.6 x 10-19 C)26
Current Mode of OperationExampleIf one million electrons are collected by the anode over a timeperiod of 10.7 ns (1.07 x 10-8 s), what is the current?I Q/tQ ne 106 x 1.6 x 10-19 C 1.6 x 10-13 CI Q/t 1.6 x 10-13 C/ 1.07 x 10-8 s 1.5 x 10-5C/s 1.5 x 10-5 amperes27
Current Mode of OperationExampleIf it took 8 nanoseconds (instead of 10.7) for the electrons toreach the anode, the current is:I 1.6 x 10-13 C/ 8 x 10-9 s 2.0 x 10-5 amperesIf it took 16 nanoseconds, the current is:I 1.6 x 10-13 C/ 16 x 10-9 s 1.0 x 10-5 amperesIn each of these three cases, exactly the same amount ofionization occurred in the detector gas and exactly the samecharge was collected at the anode.28
Current Mode of OperationExampleThe following figure shows the current as a function of timeduring these three “pulses.”The area of each is the charge. It is the same for all three.Charge (coulombs) current (amperes) x time (sec)Current(x 10-5 A)21Time (sec)29
Current Mode of OperationExampleWhen a detector operates in the current mode, theelectronics average the current over some period of time.In this example, we imagine that the detector integrationperiod is 100 nanoseconds (1 x 10-7 s).The averaged current from the detector is:I Q/t 4.8 x 10-13 C/ 1 x 10-7 s 4.8 x 10-6 amperes30
Pulse Mode ofOperation9/8/200831
Pulse Mode of OperationPulse Characteristics: Voltage (V)As long as the detector gas is not ionized, the potentialdifference between the anode and cathode is constant (e.g.,900 volts).During the movement of the electrons and positive ions to theelectrodes, the potential difference decreases.After some period of time, the voltage stops decreasing andreturns to the baseline voltage.This decrease and recovery in the voltage is the pulse. Themaximum change in the voltage is the pulse height(amplitude).32
Pulse Mode of OperationPulse Characteristics: Current (I)The movement of electrons to the anode induces a currentfrom the anode during each pulse.The magnitude of the current depends on the pulse chargeand the duration of the pulsepulse.I Q/tI is the current in amperesQ is the charge in coulombst is the time in seconds33
Pulse Mode of OperationPulse Characteristics: Charge (Q)The charge (Q) of the pulse is the charge on the electronsreaching the anode.Q neQ is the collected charge in coulombsn is the number of electrons reaching the anodee is the charge on an electron (1.6 x 10-19 coulombs)The charge is the only pulse characteristic that is alwaysrelated to the energy deposited in the detector gas.34
Pulse Mode of OperationAnalyzing the PulsesWhen a detector operates in the pulse mode, we might:1.Count the pulses, and/or2.Measure the pulse height (amplitude)For the pulse height to carry useful information about theenergy deposited in the detector gas, it must be directlyrelated to the collected charge.As the following discussion indicates, a proper choice of thetime constant (J) is necessary for the pulse height to berelated to the collected charge.35
Pulse Mode of OperationMaking Pulse Height Related to the Collected ChargeCurrent(x 10-5 A)21Time (sec)Here we see the detector current as a function of time forthree ionizing events. Each event deposits the same energyin the detector gas. The collected charge (number ofelectrons and ions reaching the electrodes) is the same ineach case. The area of each “pulse” (current x time) is the36collected charge.
Pulse Mode of OperationMaking Pulse Height Related to the Collected ChargeTime Constant (J) charge collection timeThe figure on the following slide shows pulse size (volts) as afunction of time when the time constant is much shorter thanthe charge collection time.The pulse width indicates the charge collection time.Since the time constant is small, the electronics present noresistance to the current. In addition, the system lacks thecapacitance to accumulate a charge.The potential difference (voltage) reflects the instantaneouscharge in the circuit rather than the total collected charge. 37
Pulse Mode of OperationMaking Pulse Height Related to the Collected ChargeTime Constant (J) charge collection timePulse Height(volts)Time (sec)The bottom line is that the pulse height doesn’t reflect theenergy deposited in the detector gas (total collected charge).In other words, the pulse height carries no useful information.38
Pulse Mode of OperationMaking Pulse Height Related to the Collected ChargeTime Constant (J) charge collection timeThe figure on the next slide also shows pulse size as afunction of time. But in this case, the time constant is muchgreater than the charge collection time.The large resistance to the current and the large capacitanceof the electronics means that the current is “held up” - thiscauses the potential difference (volts) to build up to thepotential maximum voltage.The maximum pulse height now reflects the energy depositedin the detector gas. This is potentially useful information!39
Pulse Mode of OperationMaking Pulse Height Related to the Collected ChargeTime Constant (J) charge collection timePulseHeight(Volts)TimeThe output pulse from the detector is negative, i.e., thevoltage decreases. Nevertheless, it is common to illustratethe pulse as if it were positive.40
Pulse Mode of OperationPulse ShapeTime Constant (J) charge collection timeRiseTailPulse Amplitude(volts)Time (sec)The pulse has two components: a rise and a tail.41
Pulse Mode of OperationPulse Rise and the Maximum Voltage (Vmax)Time Constant (J) charge collection timeThe time that the pulse takes to reach its maximum voltage(rise time) is determined by the charge collection time.When the maximum voltage is directly related to the collectedcharge (J charge collection time):Vmax is the maximum voltage decrease (volts)Q is the charge collected at the anode (coulombs)C is the detector capacitance (farads)42
Pulse Mode of OperationPulse Tail and the Time Constant (J)Time Constant (J) charge collection timeThe rate at which the pulse returns to the baseline voltage iscontrolled by the electronic characteristics of the system.Specifically,Sifi ll theth llengthth off ththe pulsel ttailil isi determinedd ti dbby ththetime constant. The time constant is calculated as follows:J RCJ is the time constant (seconds)R is the circuitry (e.g., preamplifier) input resistance (ohms)C is the circuitry and detector capacitance (farads)43
Pulse Mode of OperationPulse Tail and the Time Constant (J)Time Constant (J) charge collection timeThe following equation gives the voltage at any time after thepulse has reached its maximum amplitude:Vmax is the maximum voltage at time t 0Vt is the tail’s voltage at time tJ is the circuitry time constantThe time constant is analogous to a half life: If the timeconstant is short, the tail is short. If the time constant is large,the pulse has a long tail.44
The Six (or five)Region Curve45
Six Region CurveGeneralThe six region curve is a plot of pulse height as a function ofthe high voltage applied to a gas detector.It is sometimes depicted as a five region curve with theproportional regiongand the regiongof limited proportionalityymerged together.It indicates that theoretically a single detector can function asan ionization chamber, proportional counter or Geiger-Muellerdetector depending on the applied high voltage.When interpreting it, it can be helpful to consider the pulseheight as the number of electrons collected at the anode.46
Applied High VoltageContinuous nAlphasLimited ProportionalityyPulse Height(# electronscollected atanode)RecombinationThe Six Region Curve47
Six Region CurveGeneralThis curve, which applies to a single detector, shows theGeiger-Mueller region at a higher voltage than theproportional region.In practice, there are design differences between GeigerGeigerMueller tubes and proportional counters that cause mostGeiger-Mueller detectors to operate at lower voltages thanmost proportional counters.48
Six Region Curve1. Recombination RegionAt the lowest voltages, the strength of the electric field issufficiently low that many of the primary ion pairs created inthe gas by the incident charged particle (e.g., alpha, beta)simply recombine.If no electrons reach the anode - there is no pulse.49
Six Region Curve2. Ionization RegionIn the ionization region, the electric field is just strong enoughto prevent recombination of the ion pairs produced in the gas.One electron reaches the anode for each primary ion paircreated by the incident radiation. The curve (plateau) is flatbecause increasing the voltage doesn’t increase the numberof electrons reaching the anode.The resulting current is referred to as a saturation current.Since an alpha particle produces considerably more ion pairsthan a beta particle, the alpha pulses are correspondinglylarger.50
Six Region Curve2. Ionization RegionAlpha51
Six Region Curve2. Ionization RegionPulse Height(# electronscollected atanode)IonizationRegionAlphasBetasApplied High Voltage52
Six Region Curve3. Proportional RegionThe applied high voltage is greater and the electric field issubstantially stronger than in the ionization region.As the electrons of the primary ion pairs move towards theanode, they gain kinetic energy. When they get close enoughto the anode, the electric field is sufficiently strong (ca.106V/m), that these electrons gain enough kinetic energy tocreate secondary ion pairs.The electrons produced in these secondary ion pairs, alongwith the primary electrons, continue to gain energy as theymove towards the anode, and as they do, they produce moreand more ionizations.53
Six Region Curve3. Proportional RegionThe result is that each electron from the primary ion pairsproduces a cascade, or avalanche of ion pairs (Townsendavalanche).For everyy primaryy ion pair created in the chamber byy theionizing radiation, 102 to 104 electrons reach the anode. Theincrease in the number of electrons is referred to as themultiplication factor.As the high voltage increases, the distance from the anode atwhich the field strength exceeds 106 V/m increases. As theavalanches are initiated further away from the anode, themultiplication factor (and pulse size) increases.54
Six Region Curve3. Proportional RegionThe greater the numberof primary ion pairs thelarger the pulse.The higher the voltagevoltage,the larger the avalanchesand the larger the pulse.The figure illustrates a single pulse consisting of simultaneousfive avalanches.55
Six Region Curve3. Proportional RegionBetaParticleAnode ( )56
Six Region Curve3. Proportional RegionBetaParticleAnode ( )57
Six Region Curve3. Proportional RegionAnode ( )58
Six Region Curve3. Proportional RegionAnode ( )59
Six Region Curve3. Proportional RegionAnode ( )60
Six Region Curve3. Proportional RegionAnode ( )61
Six Region Curve3. Proportional RegionAnode ( )62
Six Region Curve3. Proportional RegionAnode ( )63
Six Region Curve3. Proportional RegionAnode ( )64
Six Region Curve3. Proportional RegionAnode ( )65
Six Region Curve3. Proportional RegionAnode ( )66
Six Region Curve3. Proportional RegionAnode ( )67
Six Region Curve3. Proportional RegionThe voltage at which the electric field strength becomes largeenough for avalanches to be produced marks the end of theionization region and the beginning of the region ofproportionality.The proportional region is sometimes referred to as theregion of true proportionality because there is a linearrelationship between the number of primary ion pairs and thenumber of electrons reaching the anode.68
Six Region Curve3. Proportional onPulse Height(# electronscollected atanode)AlphasBetasApplied High Voltage69
Six Region Curve4. Region of Limited ProportionalityThe high voltage and the multiplication factor are larger thanin the proportional region.What is now different is that pulse size is no longer linearlyrelated to the number of primary ion pairs produced by theincident radiation. In other words, doubling the number ofprimary ion pairs, doesn’t double the pulse size.This increases the uncertainty of using pulse size todistinguish different types of radiation (e.g., beta vs. alpha).70
Six Region Curve5. Geiger-Mueller RegionIn this region, the size of the pulse is totally independent ofthe number of primary ion pairs produced by the incidentradiation.In other wordswords, alpha particlesparticles, beta particles and photonsproduce pulses of exactly the same size.There is a complete loss of proportionality between theradiation energy deposited in the detector gas and the pulsesize.71
Six Region Curve5. Geiger-Mueller RegionAt the high voltages associated with the Geiger-MuellerRegion, the avalanche spreads along the complete length ofthe anode.The pulse shuts itself down when the accumulation of positiveions around the anode reduces the strength of the electricfield below that required for continued propagation.At a given voltage in the Geiger region, the size of the pulseis the same no matter how many primary ion pairs wereproduced by the incident radiation.72
Six Region Curve5. Geiger-Mueller RegionPulse Height(# electronscollected atanode)Geiger-MuellerRegionAlphasBetasApplied High Voltage73
Six Region Curve5. Geiger-Mueller RegionWhy does the avalanche spread along the anode? Becauseavalanches not only involve ionization, they also involve theexcitation of gas molecules. And when excited molecules inan avalanche de-excite, they can emit UV photons.In the Geiger-Mueller region enough UV photons areproduced for some to be absorbed by the gas molecules viathe photoelectric effect. This leads to further ionization of thegas and the creation of additional avalanches. By this means,a single avalanche becomes thousands of avalanches.The gases used in GM tubes are selected to promote thisgeneration and absorption of UV photons.74
Six Region Curve5. Geiger-Mueller RegionBetaParticleAnode ( )75
Six Region Curve5. Geiger-Mueller RegionAnode ( )76
Six Region Curve5. Geiger-Mueller RegionAnode ( )77
Six Region Curve5. Geiger-Mueller RegionPEAnode ( )78
Six Region Curve5. Geiger-Mueller Regione-Anode ( )79
Six Region Curve5. Geiger-Mueller RegionAnode ( )80
Six Region Curve5. Geiger-Mueller RegionThe result:- - - - - - - - - - - - - - - - - - - - - - - - - - - - - - - - - - - - - - - - - - - - - - - - - - - - - - - - - - - - - - - - - - - - - - - - - - - - - - - - - - - - - - - - - - - - - - - - - - - - - - - - - - - - - - - - - - - - - - - - - - - - - - - - - - - - - - - - - - - - - - - - - - - - - - - - - - - - - - - - - - - - - - - - - - - - - - - - - - - - - - - - - - - - - - - - - - - - - - - - - - - - - - - - - - - - - - - - - - - - - - - - - - - - - - - - - - - - - - - - -Anode( )The avalanche completely envelops the anode.81
Six Region Curve5. Geiger-Mueller RegionThe electrons in the avalanche are collected quickly, but notthe positive ions.This creates a positive “space” charge in the vicinity of theanodeanode.This positive charge reduces the strength of the electric fieldnear the anode.The reduced electric field strength shuts down the pulsesince further ionization is impossible.82
Six Region Curve5. Geiger-Mueller RegionThe result:- - - - - - - - - - - - - - - - - - - - - - - - - - - - - - - - - - - - - - - - - - - - - - - - - - - - - - - - - - - - - - - - - - - - - - - - - - - - - - - - - - - - - - - - - - - - - - - - - - - - - - - - - - - - - - - - - - - - - - - - - - - - - - - - - - - - - - - - - - - - - - - - - - - - - - - - - - - - - - - - - - - - - - - - - - - - - - - - - - - - - - - - - - - - - - - - - - - - - - - - - - - - - - - - - - - - - - - - - - - - - - - - - - - - - - - - - - - - - - - - -Anode( )The avalanche completely envelops the anode.83
Six Region Curve5. Geiger-Mueller Region- - - - - - - - - - - - - - - - - - - - - - - - - - - - - - - - - - - - - - - - - - - - - - - - - - - - - - - - - - - - - - - - - - - - - - - - - - - - - - - - - - - - - - - - - - - - - - - - - - - - - - - - - - - - - - - - - - - - - - - - - - - - - - - - - - - - - - - - - - - - - - - - -Anode( )The electrons are collected quickly, but not the positive ions.84
Six Region Curve5. Geiger-Mueller Region Anode( )This positive charge reduces the strength of the electric fieldnear the anode which shuts down the pulse. Furtherionization is impossible.85
Six Region Curve5. Geiger-Mueller RegionIn the Geiger-Mueller region, the plateau still slopes upwards.As the high voltage increases on the plateau, it takes morepositive ions to produce a large enough space charge toovercome the increased field strength.strength86
Six Region Curve6. Continuous DischargeIn this region, arcing will occur that can result in a completebreakdown of the gas.Any extended operation in this region will destroy the tube.ContinuousDischargePulseHeight(Volts)Applied High Voltage87
Six Region CurveWhere the Avalanche OccursWe want all the avalanches to be produced within a smallvolume around the anode, i.e., within a fraction of amillimeter.This way, the primary ion pairs produced by the radiation willbe created outside the region in which the avalanche isproduced. This is necessary if the pulse size is to reflect theenergy deposited in the gas.If primary ion pairs were produced inside the avalancheregion, their avalanches would be smaller than thoseproduced by primary ion pairs formed outside this region.This is because the avalanches would begin closer to theanode.88
Six Region CurveWhere the Avalanche OccursThis figure shows the effect of primary ion pair being formedinside the region where the avalanche occurs.The pulse on the right is smaller than it should be.89
Know the DifferenceBetween these Curves90
Know the Difference Between these erOfPulsesApplied High VoltageNumberOfPulsesApplied High VoltagePulse Height91
Know the Difference Between these CurvesPulseHeight(Volts)TimeThis is a single pulse, more or less as might be seen withan oscilloscope.92
Know the Difference Between these CurvesPulseHeight(Volts)Applied High VoltageThis is the five/six region curve.Is used to help explain the difference between ionizationchambers, proportional counters and Geiger Muellerdetectors.93
Know the Difference Between these CurvesNumberOfPulsesApplied High VoltageThis is a sometimes known as a characteristic curve.It is produced to help determine the appropriate operatingvoltage for a gas detector. They are occasionally generatedfor scintillation detectors as well.94
Know the Difference Between these CurvesNumberOfPulsesPulse HeightThis is a spectrum.The peaks on the spectrum are formed by many pulses ofsimilar height. The pulse height associated with the peakidentifies the energy of the radiation (gamma or alpha). Thenumber of counts in the peak (peak area) is related to theactivity of the source.95
Basic Characteristicsof Gas Detectors96
Basic Characteristics of Gas DetectorsIon Chambers operate in the ionization regioncan use both parallel plate and coaxial electrodesusually operate in the current modeprimarily used to measure exposure rates due to gammarays and x-raysusually poor response at low exposure rates ( 1 mR/hr)capable of measuring high exposure rates (1–1000 R/hr)energy independent (flat relative response curve)air often used as the fill gasmight be sealed or open to the atmosphereif open to atmosphere: they use a desiccant (silica gel) todry the air; their response is affected by ambientpressure and temperature97
Basic Characteristics of Gas DetectorsProportional Counters operate in the proportional/limited proportionality regionuse coaxial electrodes or variantalmost always operate in the pulse modeprimarily used to count alpha and beta sources andsurface contaminationcan distinguish small beta pulses from larger alpha pulsesmuch shorter dead time than GMs, therefore better at highcount ratesmight be sealed or operate in gas flow modegas flow mode allows operation at one atmosphere and theuse of larger an thinner window which improves efficiencyP-10 is common fill gas: 90% argon and 10% methane98
Basic Characteristics of Gas DetectorsGeiger-Mueller Detectors operate in the Geiger Mueller regionuse coaxial electrodesu
Ionization of the Detector Gas They are referred to as gas detectors because the signal is produced when charged particles ionize the gas in the detection chamber.-----7 If the gas is neon (the most common gas in a GM detector), the positive member of each ion pair is a neon ion (Ne ) while the negative member of the pair is a freed electron (e-).