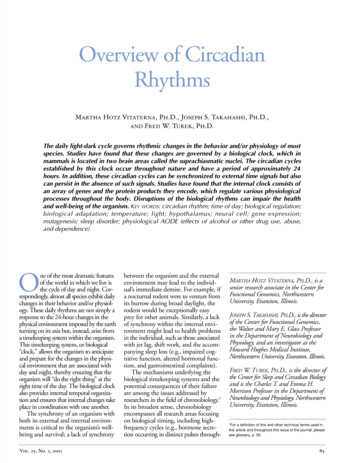
Transcription
Overview of CircadianRhythmsMartha Hotz Vitaterna, Ph.D., Joseph S. Takahashi, Ph.D.,and Fred W. Turek, Ph.D.The daily light-dark cycle governs rhythmic changes in the behavior and/or physiology of mostspecies. Studies have found that these changes are governed by a biological clock, which inmammals is located in two brain areas called the suprachiasmatic nuclei. The circadian cyclesestablished by this clock occur throughout nature and have a period of approximately 24hours. In addition, these circadian cycles can be synchronized to external time signals but alsocan persist in the absence of such signals. Studies have found that the internal clock consists ofan array of genes and the protein products they encode, which regulate various physiologicalprocesses throughout the body. Disruptions of the biological rhythms can impair the healthand well-being of the organism. KEY WORDS: circadian rhythm; time of day; biological regulation;biological adaptation; temperature; light; hypothalamus; neural cell; gene expression;mutagenesis; sleep disorder; physiological AODE (effects of alcohol or other drug use, abuse,and dependence)One of the most dramatic featuresof the world in which we live isthe cycle of day and night. Cor respondingly, almost all species exhibit dailychanges in their behavior and/or physiol ogy. These daily rhythms are not simply aresponse to the 24-hour changes in thephysical environment imposed by the earthturning on its axis but, instead, arise froma timekeeping system within the organism.This timekeeping system, or biological“clock,” allows the organism to anticipateand prepare for the changes in the physi cal environment that are associated withday and night, thereby ensuring that theorganism will “do the right thing” at theright time of the day. The biological clockalso provides internal temporal organiza tion and ensures that internal changes takeplace in coordination with one another.The synchrony of an organism withboth its external and internal environ ments is critical to the organism’s wellbeing and survival; a lack of synchronyVol. 25, No. 2, 2001between the organism and the externalenvironment may lead to the individ ual’s immediate demise. For example, ifa nocturnal rodent were to venture fromits burrow during broad daylight, therodent would be exceptionally easyprey for other animals. Similarly, a lackof synchrony within the internal envi ronment might lead to health problemsin the individual, such as those associatedwith jet lag, shift work, and the accom panying sleep loss (e.g., impaired cog nitive function, altered hormonal func tion, and gastrointestinal complaints).The mechanisms underlying thebiological timekeeping systems and thepotential consequences of their failureare among the issues addressed byresearchers in the field of chronobiology.1In its broadest sense, chronobiologyencompasses all research areas focusingon biological timing, including highfrequency cycles (e.g., hormone secre tion occurring in distinct pulses through-MARTHA HOTZ VITATERNA, PH.D., is asenior research associate in the Center forFunctional Genomics, NorthwesternUniversity, Evanston, Illinois.JOSEPH S. TAKAHASHI, PH.D., is the directorof the Center for Functional Genomics,the Walter and Mary E. Glass Professorin the Department of Neurobiology andPhysiology, and an investigator at theHoward Hughes Medical Institute,Northwestern University, Evanston, Illinois.FRED W. TUREK, PH.D., is the director ofthe Center for Sleep and Circadian Biologyand is the Charles T. and Emma H.Morrison Professor in the Department ofNeurobiology and Physiology, NorthwesternUniversity, Evanston, Illinois.1For a definition of this and other technical terms used inthis article and throughout this issue of the journal, pleasesee glossary, p. 92.85
out the day), daily cycles (e.g., activityand rest cycles), and monthly or annualcycles (e.g., reproductive cycles in somespecies). Among these interrelated areasof chronobiology, this article focuses onone frequency domain—the daily cyclesknown as circadian rhythms. (The term“circadian” derives from the Latin phrase“circa diem,” which means “about aday.”) Although virtually all life forms—including bacteria, fungi, plants, fruitflies, fish, mice, and humans—exhibitcircadian rhythms, this review is primar ily limited to the mammalian system.Other animals are discussed only incases in which they have contributed tothe understanding of the mammaliansystem, particularly in studies of themolecular genetic makeup of the timekeeping system. (For comparative dis cussions of other nonmammalian modelsystems that have contributed to thedepth of understanding of circadianrhythmicity in mammals, the reader isreferred to Wager-Smith and Kay 2000.)Overall, this article has the followingmajor objectives: (1) to provide a highlyselective historical overview of the field,(2) to review characteristic properties ofcircadian rhythms, (3) to define thestructural components and the molecu lar genetic mechanisms comprising thebiological clock, and (4) to explore thehealth effects of biological rhythms.Historical Overviewof ChronobiologyResearchers began studying biologicalrhythms approximately 50 years ago.Although no single experiment servesas the defining event from which todate the beginning of modern researchin chronobiology, studies conducted inthe 1950s on circadian rhythmicity infruit flies by Colin Pittendrigh and inhumans by Jürgen Aschoff can be con sidered its foundation. The area of sleepresearch, which also is subsumed underthe field of chronobiology, evolved somewhat independently, with the identifi cation of various sleep stages by NathanielKleitman around the same time (Dement2000). The legacies of these pioneerscontinue today with the advancementof the fields they founded.86The roots of the study of biologicalrhythms, however, reach back even fur ther, to the 1700s and the work of theFrench scientist de Mairan, who pub lished a monograph describing the dailyleaf movements of a plant. De Mairanobserved that the daily raising and low-Virtually alllife forms—including bacteria,fungi, plants, fruitflies, fish, mice,and humans—exhibit circadianrhythms.ering of the leaves continued even whenthe plant was placed in an interior roomand thus was not exposed to sunlight.This finding suggested that the move ments represented something morethan a simple response to the sun andwere controlled by an internal clock.Characteristic Propertiesof Circadian RhythmsDe Mairan’s apt observations illustrate onecritical feature of circadian rhythms—their self-sustained nature. Thus, almostall diurnal rhythms that occur undernatural conditions continue to cycleunder laboratory conditions devoidof any external time-giving cues fromthe physical environment (e.g., underconstant light or constant darkness).Circadian rhythms that are expressedin the absence of any 24-hour signalsfrom the external environment are calledfree running. This means that the rhythmis not synchronized by any cyclic changein the physical environment. Strictlyspeaking, a diurnal rhythm should notbe called circadian until it has beenshown to persist under constant envi ronmental conditions and thereby canbe distinguished from those rhythmsthat are simply a response to 24-hourenvironmental changes. For practicalpurposes, however, there is little reasonto distinguish between diurnal and cir cadian rhythms, because almost alldiurnal rhythms are found to be circa dian. Nor is a terminology distinctionmade among circadian rhythms basedon the type of environmental stimulusthat synchronizes the cycle.The persistence of rhythms in theabsence of a dark-light cycle or otherexogenous time signal (i.e., a Zeitgeber)clearly seems to indicate the existenceof some kind of internal timekeepingmechanism, or biological clock. However, some investigators have pointed outthat the persistence of rhythmicity doesnot necessarily exclude the possibilitythat other, uncontrolled cycles gener ated by the Earth’s revolution on itsaxis might be driving the rhythm (seeAschoff 1960).The hypothesis that such uncon trolled geomagnetic cues might play arole in the persistence of rhythmicitycan be refuted by a second characteris tic feature of circadian rhythms: Thesecycles persist with a period of close to,but not exactly, 24 hours. If the rhythmswere exogenously driven, they shouldpersist with a period of exactly 24 hours.The seeming imprecision is an impor tant feature of rhythmicity, however. AsPittendrigh (1960) demonstrated, thedeviation from a 24-hour cycle actuallyprovides a means for the internal timekeeping system to be continuouslyaligned by and aligned to the light-darkenvironment. This continuous adjust ment results in greater precision incontrolling the timing, or phase, of theexpressed rhythms, because little driftis allowed to occur before the rhythmis “reset” to the correct phase.A third characteristic property ofcircadian rhythms is their ability to besynchronized, or entrained, by externaltime cues, such as the light-dark cycle.Thus, although circadian rhythms canpersist in the absence of external timecues (meaning that they are not drivenby the environment), normally suchcues are present and the rhythms arealigned to them. Accordingly, if a shiftin external cues occurs (e.g., followingtravel across time zones), the rhythmsAlcohol Research & Health
Overview of Circadian RhythmsFigure 1 Circadian rhythm responses to light.A. Parameters of circadian uous DarknessDayTimeA representative circadian rhythm is depicted in which the level of a particular measure(e.g., blood hormone levels and activity levels) varies according to time. The differ ence in the level between peak and trough values is the amplitude of the rhythm.The timing of a reference point in the cycle (e.g., the peak) relative to a fixed event(e.g., beginning of the night phase) is the phase. The time interval between phasereference points (e.g., two peaks) is called the period. The rhythm shown persistseven in continuous darkness (i.e., is free running).B. Resetting the circadian rhythmLevelDelayContinuous DarknessAdvanceContinuous DarknessTimeThe effects of a rhythm-resetting signal, such as exposure to light by animals otherwise kept in continuous darkness, can shift the rhythm either back (upper panel) orahead (lower panel), depending on when during the cycle the signal is presented. Inthe case of a phase delay, the peak levels are reached later than they would be hadthe rhythm not been shifted. In the case of a phase advance, the peak levels arereached earlier than they would be had the rhythm not been shifted. The black lineshows how cycling would appear if the rhythm remained unchanged.C. Changes in circadian rhythm in response to changes in light exposurePhase Shift (hours) 6Subjective DaySubjective NightAdvances0Delays-6CT 0CT 12CT 0Circadian Phase of Light ExposureVirtually all species show similar phase-dependent-resetting responses to light, whichcan be expressed as a phase-response curve. Exposure to light during the early partof the animal’s night causes a phase delay, whereas exposure to light in the latter partof the animal’s night causes a phase advance. Light exposure during the animal’susual daytime period produces little or no phase shift.Vol. 25, No. 2, 2001will be aligned to the new cues. Thisalignment is called entrainment.Initially, it was unclear whetherentrainment was achieved by modulat ing the rate of cycling (i.e., whether thecycle was shortened or lengthened untilit was aligned to the new cues and thenreverted to its original length) or whetherentrainment was achieved by discrete“resetting” events. Experiments result ing from this debate led to fundamen tal discoveries. For example, researchersdiscovered that the organism’s responseto light (i.e., whether a cycle advances,is delayed, or remains unchanged) dif fers depending on the phase in thecycle at which it is presented (Pittendrigh1960). Thus, exposure to light duringthe early part of the individual’s “nor mal” dark period generally results in aphase delay, whereas exposure to lightduring the late part of the individual’snormal dark period generally results ina phase advance. This difference inresponses can be represented by a phaseresponse curve (see figure 1 for a schematicillustration of a circadian cycle as wellas a phase-response curve). Such acurve can predict the manner in whichan organism will entrain not only toshifts in the light-dark cycles but alsoto unusual light cycles, such as non-24hour cycles or different light:dark ratios.The existence of a phase-response curvealso implies that entrainment is achievedby discrete resetting events rather thanchanges in the rate of cycling.In addition to the timing of the lightexposure, the light intensity can modu late cycling periods when organisms areleft in constant light. Thus, exposure tobrighter light intensities can lengthen theperiod in some species and shorten it inother species. This phenomenon has beendubbed “Aschoff’s rule” (Aschoff 1960).Ultimately, both mechanisms of entrain ment appear to be aspects of the samething, because the consequences ofAschoff’s rule can be predicted or explain ed by the phase-response curves to light.Although the light-dark cycle clearlyis the major Zeitgeber for all organisms,other factors—such as social interactions,activity or exercise, and even tempera ture—also can modulate a cycle’s phase.The influence of temperature on circa dian rhythms is particularly interesting87
in that a change in temperature canaffect the phase of a cycle without sub stantially altering the rate of cycling.This means that the cycle may start atan earlier or later-than-normal time butstill have the same length. On the onehand, this ability of the internal clock’spacemaker to compensate for changesin temperature is critical to its abilityto predict and adapt to environmentalchanges, because a clock that speedsup
species. Studies have found that these changes are governed by a biological clock, which in mammals is located in two brain areas called the suprachiasmatic nuclei. The circadian cycles established by this clock occur throughout nature and have a period of approximately 24 hours. In addition, these circadian cycles can be synchronized to external time signals but also