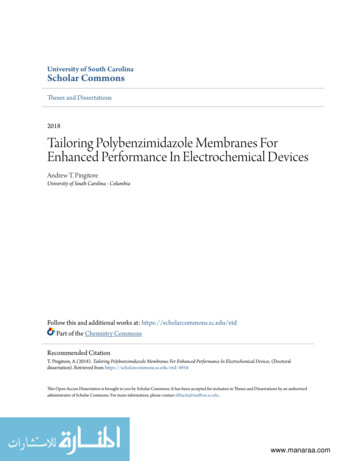
Transcription
University of South CarolinaScholar CommonsTheses and Dissertations2018Tailoring Polybenzimidazole Membranes ForEnhanced Performance In Electrochemical DevicesAndrew T. PingitoreUniversity of South Carolina - ColumbiaFollow this and additional works at: https://scholarcommons.sc.edu/etdPart of the Chemistry CommonsRecommended CitationT. Pingitore, A.(2018). Tailoring Polybenzimidazole Membranes For Enhanced Performance In Electrochemical Devices. (Doctoraldissertation). Retrieved from https://scholarcommons.sc.edu/etd/4934This Open Access Dissertation is brought to you by Scholar Commons. It has been accepted for inclusion in Theses and Dissertations by an authorizedadministrator of Scholar Commons. For more information, please contact dillarda@mailbox.sc.edu.www.manaraa.com
TAILORING POLYBENZIMIDAZOLE MEMBRANES FOR ENHANCEDPERFORMANCE IN ELECTROCHEMICAL DEVICESbyAndrew T. PingitoreBachelor of ArtsWashington & Jefferson College, 2014Submitted in Partial Fulfillment of the RequirementsFor the Degree of Doctor of Philosophy inChemistryCollege of Arts and SciencesUniversity of South Carolina2018Accepted by:Brian C. Benicewicz, Major ProfessorChuanbing Tang, Committee MemberJohn W. Weidner, Committee MemberAaron Vannucci, Committee MemberCheryl L. Addy, Vice Provost and Dean of the Graduate Schoolwww.manaraa.com
Copyright by Andrew T. Pingitore, 2018All Rights Reserved.iiwww.manaraa.com
DEDICATIONIn loving memory of my grandmothers, Irene Fischer and Margaret Snyder, andgrandfather, Gerred Snyder.iiiwww.manaraa.com
ACKNOWLEDGEMENTSFirst and foremost I would like to thank my research advisor, Dr. Brian C.Benicewicz, for all of his support and guidance on my research projects. It would take morethan one Ph.D. to acquire a fragment of his knowledge and creativity in polymer scienceand his enthusiastic approach and love of science helped to keep me motivated through allthose late nights in the lab. I am sincerely grateful for all the opportunities and experiencesI was given in his group.I would like thank Dr. John W. Weidner for the opportunity to collaborate with hisgroup and his support through my Ph.D. candidacy. I would also like to thank Dr.Chuanbing Tang for his guidance and interest in my research. I would also like to thankDr. Aaron Vannucci for his support and valuable suggestions on my research. - Thankyou all for being on my doctoral committee, as well.I would like to thank the entire Benicewicz Group, both past and present, for all ofthe support, suggestions and friendship. I would especially like to thank Kayley FishelHayat for mentoring me in beginning years and being a truly great lab mate and even betterfriend. The fun and laughs we shared were invaluable and truly missed. I’d also like tothank Julia Pribyl for our office vent sessions and for all of the support and ideas. I wouldalso like to thank Zachary Marsh for being a great friend and all of the support, fun, andbeers we had along the way. I would also like to thank Kayla Lantz for her friendship,support, listening to me vent.ivwww.manaraa.com
Next, I would like to thank my parents, siblings, and loving grandparents for theirunwavering support, availability to talk no matter what time of the day, and traveling tovisit me. I would also like to give my deepest gratitude to my girlfriend, Tori Fecteau, whohas been extremely supportive and helpful throughout my program, putting up with all ofthe late nights and weekends I’d spend in the lab, and subsequently taking care of my dogBill; who I would also like thank for always be so happy when I got home.Finally, I would like to acknowledge all of the funding support and researchopportunities I was given through my collaborations with BASF GmbH, UnitedTechnologies Research Center, Johnson R&D Co., and PBI Performance Products.vwww.manaraa.com
ABSTRACTAfter approximately 15 years of development, polybenzimidazole (PBI)chemistries and the concomitant manufacturing processes have evolved into commerciallyproduced membrane electrode assemblies (MEAs). PBI MEAs can operate reliably withoutcomplex water humidification hardware and are able to run at elevated temperatures of120-180 OC due to the physical and chemical robustness of PBI membranes. These highertemperatures improve the electrode kinetics and conductivity of the MEAs, simplify thewater and thermal management of the systems, and significantly increase their tolerance tofuel impurities. Membranes cast by a newly developed polyphosphoric acid (PPA) Processpossessed excellent mechanical properties, higher phosphoric acid (PA)/PBI ratios, andenhanced proton conductivities as compared to previous methods of membranepreparation. p-PBI and m-PBI are the most common polymers in PBI-based fuel cellsystems, although AB-PBI and other derivatives have been investigated. The workpresented in this dissertation demonstrates the chemical flexibility of PBI polymers whichenables the tailoring of specific membrane properties enhancing performance in new anddifferent electrochemical devices with diverse operating conditions.viwww.manaraa.com
TABLE OF CONTENTSDEDICATION . iiiACKNOWLEDGEMENTS . ivABSTRACT . viLIST OF TABLES .xLIST OF FIGURES . xiLIST OF SYMBOLS .xvLIST OF ABBREVIATIONS . xviiCHAPTER 1: GENERAL INTRODUCTION .11.1 ABSTRACT .21.2 INTRODUCTION TO POLYBENZIMIDAZOLE FUEL CELLSUSTAINABILITY .21.3 HISTORY OF PBI MEMBRANES.61.4 SYNTHESIS OF POLYBENZIMIDAZOLE .81.5 ELECTROCHEMICAL DEVICES .111.6 REFERENCES .22CHAPTER 2: DURABLE HIGH POLYMER CONTENT M/P PBI MEMBRANES FOREXTENDED LIFE-TIME ELECTROCHEMICAL DEVICES .242.1 ABSTRACT .252.2 INTRODUCTION .252.3 EXPERIMENTAL .282.4 RESULTS AND DISCUSSION .33viiwww.manaraa.com
2.5 CONCLUSION .442.6 REFERENCES .46CHAPTER 3: TAILORING PBI MEMBRANES FOR ELECTROCHEMICALHYDROGEN SEPARATION .483.1 ABSTRACT .493.2 INTRODUCTION .503.3 EXPERIMENTAL .563.4 RESULTS AND DISCUSSION .623.5 CONCLUSION .823.6 REFERENCES .843.7 PERFORMANCE SUMMARY .85CHAPTER 4: SULFONATED MEMBRANES FOR SO2 DEPOLARIZEDELECTROLYZERS .864.1 ABSTRACT .874.2 INTRODUCTION .874.3 EXPERIMENTAL .914.4 RESULTS AND DISCUSSION .964.5 CONCLUSION .1094.6 REFERENCES .110CHAPTER 5: ADVANCED MEMBRANES FOR REDOX FLOW BATTERIES .1135.1 ABSTRACT .1145.2 INTRODUCTION .1145.3 EXPERIMENTAL .1165.4 RESULTS AND DISCUSSION .121viiiwww.manaraa.com
5.5 CONCLUSION .1285.6 ACKNOWLEDGMENTS .1285.7 REFERENCES .129CHAPTER 6: SUMMARY AND OUTLOOK.1316.1 SUMMARY .1326.2 CONCLUSION .1346.3 REFERENCES .135APPENDIX A: PERMISSION TO PRINT .136ixwww.manaraa.com
LIST OF TABLESTable 1.1 Comparison of conventionally imbibed m-PBI vs m-PBI synthesized from thePPA Process .11Table 2.1 Polymer solution characteristics and membrane composition .34Table 3.1 Notable ex-situ membrane results.62Table 3.2 Membrane EHS performance and power consumption under variousconditions .85Table 4.1 s-PBI variants and acid loading technique on membrane composition andconductivity.99Table 5.1 Ex-situ properties of s-PBI gel membranes compared to densem-PBI films. .123Table 5.2 Oxidative stability of sulfonated PBI gel membranes in V5 solutions. .125xwww.manaraa.com
LIST OF FIGURESFigure 1.1 World electricity production by source. .3Figure 1.2 Global production of carbon dioxide annually from 1990-2015 .5Figure 1.3 Chemical structure of poly(2,2’-m-phenylene-5,5’-bibenzimidazole) .7Figure 1.4 State diagram of the PPA Sol-Gel Process .10Figure 1.5 Polymer electrolyte membrane fuel cell .12Figure 1.6 Polymer electrolyte membrane used for hydrogen electrolysis .14Figure 1.7 Initial hydrogen pump data with PBI membranes vs. theoretical .18Figure 2.1 Proton conductivities of m/p-PBI copolymers .36Figure 2.2 Creep deformation of m/p-PBI copolymers .37Figure 2.3 m/p-PBI 10(7:1) copolymer fuel cell performance .39Figure 2.4 Long-term steady state durability test of m/p-PBI 10(7:1) copolymer.40Figure 2.5 PA loss rates from the anode and cathode of m/p-PBI 10(7:1) Copolymer .41Figure 2.6 Electrochemical pump data for m/p-PBI 10(7:1) Copolymer, H2 feed .42Figure 2.7 Electrochemical pump data for m/p-PBI 10(7:1) Copolymer using a reformatefeed stream (30 % H2, 3 % CO, and 67 % N2).44Figure 3.1 Polymer electrolyte membrane for hydrogen electrolysis .51Figure 3.2 The cathodic flow rates of a hydrogen pump operated at 160 OC and 0%relative humidity and fueled by pure hydrogen (unfilled squares), a reformate gascomprised of 35.8% H2, 11.9% CO2, 1906 ppm CO, and 52.11% N2 (filledcircles), and a reformate gas comprised of 69.17% H2, 29.8% CO2, and 1.03% CO(filled triangles). The values are nearly identical, and thus, the symbols appearsuperimposed. The dotted line represents the theoretical flow rate at 100%efficiency.55xiwww.manaraa.com
Figure 3.3 Homemade burst testing apparatus .60Figure 3.4 DMA results of selected membranes .65Figure 3.5 Celtec-P performance in an EHS cell with pure H2 as the feed gas .69Figure 3.6 Celtec-P performance in an EHS cell. Test gas is reformate (30 mol %H2, 3 mol % CO, and 67 mol % N2). .70Figure 3.7 Celtec-P under EHS conditions. Test gas is pure hydrogen, 1.5 stoich. Celltemperature is 160 C with constant 45 C water bottle for humidification (1.6 %RH). Pressure was cycled as follows: 0 psi – 15 psi – 30 psi – 0 psi, once a day for3 days. .72Figure 3.8 Long-term durability of Celtec-P under a differential pressure of 30 psi onthe anode at 160 C, 0.2 A/cm2. .73Figure 3.9 Celtec-P crosslinked with paraformaldehyde under EHS conditions. Pure H2test gas, 1.5 stoich. (minimum of 50 SCCM), and constant 45 C water bottletemperature for humidification. .74Figure 3.10 M-r-p PBI gel membrane under EHS conditions using pure hydrogen. Closedsymbol 1.6 % RH and open symbols 2.6 % RH.76Figure 3.11 M-r-p based MEAs with the same pressure cycling previously shown onCeltec-P (0 psi – 15 psi – 30 psi – 0 psi using pure hydrogen). Black linesrepresent day 1, red day 3, blue day 5, and green day 10. At 0 Δp filled in squaresrepresent polarization curves at the beginning of the day and open circles arethose at the end of the day. The graph in the bottom right (m-r-p black lines,Celtec-P red lines) shows no back-pressure results at the beginning of the test(filled in squares) and no pressure at the end of 3 days (open circles). .77Figure 3.12 M-r-p compared to Celtec-P with a reformate feed stream of 30, 3, and 67mol % of H2, CO, N2 respectively. Black lines are at 160 C, red 180 C, and blue200 C .78Figure 3.13 Mrp-X under EHS conditions with pure H2 as the feed stream. Black lines are160 C, red 180 C, and blue 200 C. Water bottle temperatures were adjusted toreach desired % RH and allowed to equilibrate. .79Figure 3.14 Mrp-X in EHS mode, Celtec-P for comparison, with reformate test gascomprised of 30, 3, and 67 mol % H2, CO, and N2 respectively. Black linescorrespond to 160 C, red 180 C, and blue 200 C. Water bottle temperature wasmaintained at 45 C with 1.5 stoich gas flows according to H2.80xiiwww.manaraa.com
Figure 3.15 Long-term EHS performance of m/p PBI 10(7:1) under various conditions.Hydrogen stoichiometry was kept at a constant of 1.25 and a differential pressureof 30 psi was applied to the anode (except as noted in the red box).81Figure 4.1 The sulfuric acid concentration (top) and the cell voltage at 0.5 A/cm2(bottom) for Nafion at 80 C at two differential pressures (ΔP) and s-PBI at 110 C. .90Figure 4.2 a.) Predicted regioselectivity of an EAS reaction on a p-PBI r.u. Green circlesrepresent areas sites with free energies below 1 kcal mol-1 and red circles indicatefree energies below 3 kcal mol-1. b.) Location of the sulfonate group via the presulfonation technique. .98Figure 4.3 Anhydrous conductivities of sulfonated PBIs from room temperature to140 C. .100Figure 4.4 Specific area resistance as a function of sulfuric acid concentration for s-PBIobtained from multiple membranes compared to Nafion 115 and Nafion 212.ΔP 600 kPa for the Nafion membranes, and no pressure differential used for sPBI. .102Figure 4.5 Sulfuric-acid concentrations produced in the cell at 0.5 A/cm2 and either 80(circles) or 110 C (squares) as a function of water stoichiometry. The waterstoichiometry refers to the ratio of the moles of water fed to the cathode to thatrequired via Eqn. 4 at a given current. The lines are the acid concentrationspredicted from the Mixed Solvent Electrolyte Thermodynamics Framework(MSE) package in the OLI Systems, Inc. electrolyte software. .103Figure 4.6 Individual potential contributions towards the overall cell voltage for the HySelectrolyzer at 110 C and a constant water feed rate of 0.45 mL/min. Linesrepresent model predictions and the symbols are the cell voltages (filled symbols)and anodic overpotentials (open symbols) data. .106Figure 4.7 Contributions towards total operating voltage across a range of temperatures inthe HyS electrolyzer at a current density of 0.5 A/cm2 and a constantwater flow rate of 0.50 mL/min. Lines represent model predictions and thesymbols are the cell voltages (filled symbols) and anodic overpotentials (opensymbols) data. .107Figure 4.8 Model predictions compared to experimental data at three different currentdensities across a range of temperatures in the HyS electrolyzer at a constantwater flow rate of 0.50 mL/min. The solid lines represent model predictions anddotted line represents the model fit at 0.5 A/cm2 .108xiiiwww.manaraa.com
Figure 5.1 A.) Polarization curves with 80% state-of-charge electrolyte and cyclingefficiencies [B.) voltage efficiencies, C.) coulombic efficiencies, and D.) energyefficiencies] of s-PBI, s-PBI-x, and m-PBI (conventionally imbibed) in avanadium redox flow battery. .126xivwww.manaraa.com
LIST OF SYMBOLSENernst Nernst PotentialE Standard PotentialRUniversal Gas ConstantTTemperatureFFaraday’s ConstantioExchange Current Density𝐽𝑠0Creep CompliancedDistance between the two inner electrodes.lThickness of the membrane.wMembrane widthRmMembrane ohmic resistance𝑑𝐽 𝑑𝑡 Creep RateRASpecific Area ResistanceHFRHigh Frequency ResistanceiRAOhmic Resistance in the MembraneUeqEquilibrium PotentialMSE Mixed Solvent Electrolyte Thermodynamics Framework PackagekKinetic Term Dependent on Temperature in The Arrhenius Relationship𝜂𝑎Anodic Overpotentialxvwww.manaraa.com
cr(t)Receptor VOSO4 Concentration at Time t in Permeability Cellcr(0)Donor Initial VOSO4 Concentration in Permeability CellVVolume of the Donor and Receptor Permeability CelldThickness of Membrane used in Permeability TestingAActive Area of the Membrane used in Permeability TestingPsPermeability of Salt Through the Membranexviwww.manaraa.com
LIST OF ABBREVIATIONSPEM . Polymer Electrolyte MembranePEMFC . Polymer Electrolyte Fuel CellMEA. Membrane Electrode AssemblyPA . Phosphoric AcidSA . Sulfuric AcidTFA . Trifluoroacetic AcidPBI . PolybenzimidazolePPA .Polyphosphoric AcidRU . Repeat UnitPSA . Pressure Swing AbsorptionPFSA . Perfluorosulfonic AcidEHS . Electrochemical Hydrogen SeparationHyS . Hybrid Sulfur CycleHT-PEM . High Temperature Polymer Electrolyte Fuel CellLT-PEM . Low Temperature Polymer Electrolyte Fuel CellDMAc . N,N’-DimethylacetamideTPA .Terephthalic AcidIPA . Isophthalic AcidTAB. 3,3’,4,4’-tetraamine-(1,1’-biphenyl)R.H. . Relative HumidityGDE . Gas Diffusion Electrodexviiwww.manaraa.com
DCX . α,α’-Dichloro-p-xyleneDCB .4,4’-Bis(chloromethyl)biphenyls-PBI .Sulfonated PolybenzimidazoleSDE . SO2 Depolarized ElectrolyzerS-TAB . Sulfonylbis-1,2-benzenediamines-TPA .Monosodium 2-SulfoterephthalateSAXS .Small Angle X-Ray ScatteringWAXS . Wide Angle X-Ray Scatteringxviiiwww.manaraa.com
CHAPTER 1GENERAL INTRODUCTIONREPRINTED IN PART WITH PERMISSION FROM SPRINGERINTERNATIONAL PUBLISINGPingitore, A. T., Molleo, M., Schmidt T. J., Benicewicz B. C. Polybenzimidazole FuelCell Technology: Theory, Performance, and Applications; Springer InternationalPublishing, 2018.1www.manaraa.com
1.1 Abstract.After approximately 15 years of development, polybenzimidazole (PBI)chemistries and the concomitant manufacturing processes have evolved into commerciallyproduced membrane electrode assemblies (MEAs). PBI MEAs can operate reliably withoutcomplex water humidification hardware and are able to run at elevated temperatures of120-180 OC due to the physical and chemical robustness of PBI membranes. These highertemperatures improve the electrode kinetics and conductivity of the MEAs, simplify thewater and thermal management of the systems, and significantly increase their tolerance tofuel impurities. Membranes cast by a newly developed polyphosphoric acid (PPA) Processpossessed excellent mechanical properties, higher phosphoric acid (PA)/PBI ratios, andenhanced proton conductivities as compared to previous methods of membranepreparation. p-PBI and m-PBI are the most common polymers in PBI-based fuel cellsystems, although AB-PBI and other derivatives have been investigated. This chapterreports on the chemistries and sustainable usages of PBI-based high temperature protonexchange membrane fuel cells (PEMFCs).1.2 Introduction to Polybenzimidazole Fuel Cell Sustainability.Alternative energy is often defined as any energy derived from sources other thanfossil fuels or nuclear fission. These alternative energy sources, which include solar, wind,hydro, and geothermal energy, are considered renewable because they are naturallyreplenished and their supply is seemingly limitless. In contrast, the Earth’s supply of fossilfuels is constantly being diminished. Fossil fuels, which include crude oil, coal, and naturalgas, continue to be the dominating sources of energy in the world (Figure 1.1). Fossil fuelsprovide more than 86% of the total energy consumed globally.(1, 2) In 2009, the electrical2www.manaraa.com
power sector was the largest source of carbon dioxide emissions (40% of all energy-relatedCO2 emissions) and was followed closely by the transportation sector which was 34% ofthe total.(3) It is predicted that the global demand for fossil fuels will continue to increaseover the next 10-20 years due to economic growth. One may conclude that the importanceof renewable energy will steadily increase as the Earth’s supply of fossil fuels continues tobe depleted.Figure 1.1: World net electricity production by source, 2012-40 (trillion kilowatt hours).(1)Polymer electrolyte membrane (PEM) fuel cells, also known as proton exchangemembrane fuel cells (PEMFCs), are energy conversion devices that could provide theworld with clean and efficient energy. Due to their excellent energy production,inexpensive starting materials, and lack of pollutant byproducts, these cells haveexponentially gained in popularity over the past decade. Electricity is produced at the heartof the fuel cell by the membrane electrode assembly (MEA), a component that is comprised3www.manaraa.com
of a proton exchange membrane sandwiched between two electrodes. Fueled by ahydrogen-based source, a metal catalyst at the anode splits the hydrogen into protons andelectrons. As the protons are transported through the proton electrolyte membrane to thecathode, the electrons provide electrical work by traveling around the membrane throughan external circuit from the anode to the cathode. The protons and electrons react with anoxidant (typically air or pure oxygen) at the cathode to form water, thereby completing theelectrochemical cycle. Hydrogen gas is commonly used as a fuel source for the cells, butother fuels such as methane, methanol, and ethanol have been explored.PEM fuel cells provide multiple advantages over conventional fossil fuel energyproduction. Because water is the only byproduct of the electrochemical process, these fuelcells are clean and environmentally friendly. If one considers the tremendous amount ofcarbon dioxide created by energy production on the global scale (Figure 1.2), PEM fuelcells offer a method to significantly reduce hazardous gas emissions. Minimal moving partsreduces the amount of maintenance of each cell, and the lack of combustion significantlydecreases the amount of harmful pollutants such as sulfur oxides and nitrogen oxides. Inaddition, PEM fuel cells are much more efficient at producing energy and, much like acombustion engine, the cell can run continuously as long as fuel and oxidant are provided.Although fuel cells are an environmentally friendly energy conversion device, one mustconsider the way hydrogen is gathered. Both hydrogen production and conversion fromchemical to elec
www.manaraa.com University of South Carolina Scholar Commons Theses and Dissertations 2018 Tailoring Polybenzimidazole Membranes For Enhanced Performance In Electrochemical Devices