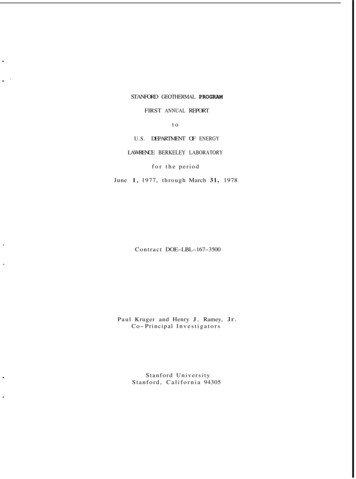
Transcription
STANFORD GEOTHERMAL PROGRAMFIRST ANNUAL REPORTtoU.S.DEPARTMENT OF ENERGYLAWRENCE BERKELEY LABORATORYf o r the periodJune 1, 1977, t h r o u g h March 3 1 , 1978C o n t r a c t DOE-LBL-167-3500P a u l Kruger and Henry J . Ramey, J r .Co- Principal I n v e s t i g a t o r sStanford UniversityS t a n f o r d , C a l i f o r n i a 94305
TABLE OF CONTENTS1.INTRODUCTION12.ENERGY TRANSFER EXPERIMENTS43.RADON ANALYSIS PROJECTS234.BENCH-SCALE FLOW EXPERIMENTS355.WELL TEST ANALYSIS496.CONCLUDING PPENDIX A : STANDARD GEOTHERMAL PROGRAM WEEKLY SEMINARii65
.1.INTRODUCTIONThis report is the first annual progress report under the Depart-ment of Energy Contract DOE-LBL-167-3500 with Lawrence Berkeley Laboratory.It covers the pteriod June 1, 1977, to March 31, 1978.At the close of tihe first year of support from the Department ofEnergy, the Stanford Geothermal Program has maintained momentum built upunder the previous National Science Foundation support.A number ofcurrent projects have been completed during the year, and others havebegun and are underway.The central thrust of the program has been the development of techniques of geothermal reservoir engineering.This crucial field holds agreat deal of importance in the comparatively new industry of geothermaldevelopment, and it is through the greater understanding brought aboutin reservoir engineering that the geothermal energy business will reachmaturity.The program has investigated applications over quite a broadarea in two major categories: fundamental studies and field applicationstudies.Among the fundamental studies are the laboratory experiments examining the behavior of rocklwater systems under geothermal conditions.The large geothermal reservoir model, built at Stanford by the program,has been used to investigate the specific energy extraction capabilitiesof various production methods.This model has a l s o been used to deter-mine the rate of heat loss from various rock formations, with a particular view to providing an empirical representation of heat extractionfrom rock.During the year, the large reservoir model was also packedwith sand (in the interstices of the rock matrix) to provide a morerealistic permeabilitylporosity formation.These results are presentedin Section 2 .A parallel laboratory and field study in progress in the program isthe radon analysis.Radon collections from geothermal fields at the1
2G e y s e r s , L a r d a r e l l o , H a w a i i , and t h e h o t , d r y r o c k system a t Los Alamoshave p r o v i d e d a v a r i e t y of r e s p o n s e s t h a t a r e now b e i n g e v a l u a t e d as ameans of i l l u s t r a t i n g r e s e r v o i r p r o p e r t i e s .Radon i s a p a r t i c u l a r l yu s e f u l t r a c e f o r t h i s k i n d of a p p l i c a t i o n s i n c e i t h a s a v e r y u s e f u lh a l f - l i f e of a b o u t 4 d a y s .The h o t , d r y r o c k radon samples a p p e a r t oshow a n i n c r e a s e i n s u r f a c e area of t h e h y d r a u l i c a l l y produced f r a c t u r e ,s u g g e s t i n g t h e u s e of radon m o n i t o r i n g as a n i n d i c a t o r of t h e r m a l stressI n o r d e r t o q u a n t i f y t h e e m i s s i o n r a t e s of radon from reser-cracking.v o i r r o c k s , a n e x p e r i m e n t a l a p p a r a t u s i s under c o n s t r u c t i o n t o p r o v i d ee x a c t i n f o r m a t i o n on t h e t e m p e r a t u r e , p r e s s u r e , and r o c k p r o p e r t y depende n c e of t h e radon p r o d u c t i o n .These v a r i o u s s t u d i e s are p r e s e n t e d i nSection 3.Another s e t of fundamental i n v e s t i g a t i o n s i n v o l v e t h e u s e of l a b o r a t o r y c o r e s i n bench- scale experimental r i g s .Three s t u d i e s have beenc a r r i e d o u t : f i r s t , i n p u r s u i t of steamlwater r e l a t i v e p e r m e a b i l i t yc u r v e s f o r permeable f o r m a t i o n s ; s e c o n d , i n v e s t i g a t i n g t h e p r o p e r t y o fvapor p r e s s u r e l o w e r i n g of water c o n f i n e d i n p o r o u s media a t l o w s a t u r a t i o n s ; and t h i r d , t h e d e t e r m i n a t i o n o f t h e e f f e c t s of h i g h c o n f i n i n gp r e s s u r e s and t e m p e r a t u r e s on t h e a b s o l u t e p e r m e a b i l i t y of porous r o c k s .These i n v e s t i g a t i o n s c o l l e c t i v e l y p r o v i d e an e x a c t d e s c r i p t i o n of t h ef l o w t h r o u g h a g e o t h e r m a l f o r m a t i o n on a m i c r o s c o p i c s c a l e .A beneficials i d e l i n e of t h i s r e s e a r c h i s t h a t i t p r o v i d e s c a r e f u l l y c o n t r o l l e d models i t u a t i o n s t h a t can b e used a s a b a s i s f o r c a l i b r a t i o n o f n u m e r i c a lmodels t h a t may t h e n b e a p p l i e d w i t h g r e a t e r c o n f i d e n c e t o t h e l a r g e runknown of a g e o t h e r m a l r e s e r v o i r .S e c t i o n 4 d e s c r i b e s t h e work i n t h i sfield.F i n a l l y , a major i n t e r e s t of t h e program i s i n t h e area of w e l lt e s t analysis.Although t h e l a b o r a t o r y d a t a p r o v i d e s i m p o r t a n t d a t a onfundamental p r o p e r t i e s of g e o t h e r m a l o p e r a t i o n s , a t l e a s t an e q u a l emp h a s i s must b e p l a c e d on i n f o r m a t i o n p r o v i d e d by t h e g e o t h e r m a l f i e l ditself.The c o n d u i t f o r t h i s i n f o r m a t i o n i s t h e g e o t h e r m a l w e l l b o r e ,and t h u s t h e p r o j e c t s f o c u s on t h e i n t e r p r e t a t i o n and d e s i g n of w e l ltests.The most i m p o r t a n t d i r e c t i o n i n t h i s r e g a r d i s i n t h e f i e l d o fp r e s s u r e t r a n s i e n t a n a l y s i s , and t h r e e s e p a r a t e s t u d i e s have been p e r formed d u r i n g t h e y e a r .I n t h e p a r a l l e l area of d i s c h a r g e a n a l y s i s ,
3t h e program h a s a d d r e s s e d t h e problem of e v a l u a t i n g w e l l b o r e h e a t andp r e s s u r e l o s s e s , as w e l l as d i s c h a r g e v a r i a t i o n s due t o r e l a t i v e permeab i l i t y effects.These and t h e bench s c a l e s t u d i e s w i l l b e t h e s t a r t i n gp o i n t of new developments i n p r e s s u r e t r a n s i e n t a n a l y s i s of two-phasewells--a p r e s s i n g need i n t h e geothermal i n d u s t r y t h a t h a s y e t t o b efilled.Well t e s t a n a l y s i s i s d e s c r i b e d i n S e c t i o n 5.The S t a n f o r d Geothermal Program i s a mobile and e x p a n s i v e groupt h a t f u l f i l l s o t h e r s e c o n d a r y r o l e s i n t h e geothermal community.Othert h a n f i l l i n g t h e U n i v e r s i t y ' s t a s k of p r o v i d i n g t r a i n i n g f o r new geot h e r m a l p e r s o n n e l , t h e program h a s p r o v i d e d s e v e r a l o r g a n i z e d s e r v i c e st o t h e n e a r b y geothermal c o n c e r n s .The Annual S t a n f o r d GeothermalR e s e r v o i r E n g i n e e r i n g Workshop was h e l d f o r t h e t h i r d time i n December1977, and w a s a t t e n d e d by r e p r e s e n t a t i v e s from t h e U.S.,Zealand, Mexico, and Japan.I t a l y , NewThe weekly s e m i n a r s c o n t i n u e d t o b e p o p u l a rt h i s y e a r , and w e r e h e l d throughout t h e f a l l , w i n t e r , and s p r i n g q u a r t e r s .These s e m i n a r s have produced many f r u i t f u l i n t e r c h a n g e s of i n f o r m a t i o nb o t h i n t o and from t h e S t a n f o r d program.T h i s involvement of t h e programi s d e s c r i b e d i n t h e c o n c l u d i n g remarks, S e c t i o n 6.
2.ENERGY TRANSFER EXPERIMENTS2.1 Energy Extraction ExperimentsMuch of the immense quantity of geothermal energy stored in theearth's crust is widely dispersed and occurs as hot igneous rock withpermeabilities that are too low for adequate fluid circulation.Fracture-stimulation of such systems is needed to improve fluid circulation andexpose new heat transfer surface in the hot rock.Hydrothermal resourceswhich may need fracture stimulation are those with inadequate fluid content for heat removal flow rates or those in which the transit time ofreinjected fluids is too rapid for adequate reheating. Fracturestimulation techniques proposed to enhance the energy recovery includehydraulic or explosive fracturing and thermal stress cracking.Experi-mental methods needed t o evaluate the thermal extraction effectivenessof such stimulation practices and of hydrothermal reservoirs in generalare a part of the Stanford Geothermal Program (SGP).Experiments are being conducted in the SGP large geothermal reservoir model utilizing rock systems with several characteristics resemblinghigh permeability, fracture-stimulated systems.The broad objective ofthese experiments is to evaluate nonisothermal fluid production and heattransfer processes and to analytically model these for such rock systems.Three nonisothermal energy extraction and production processes, referredto here as in-place boiling, sweep, and steam-drive, were consideredduring the early phases of this study.The general production, injection,and reservoir conditions maintained during the three different experimentsare listed in Table 2.1.This work has been reported in previous reports and papers (Hunshedt, Kruger, and London, 1975, 1977, and 1978).The results showedthat all three processes are feasible in the experimental systems considered.However, the effectiveness of the process, as illustrated in,Table 2.2, varied widely.4
5TABLE 2.1: TYPES OF ENERGY EXTRACTION gPressure reduction and boiling in formation. Production of steam from a top producing zone with or without fluid recharge at the bottom.SweepInjection of cold water at bottom. Hot water producedfrom a top producing zone. Compressed liquid reservoir.St eam-Dr iveProduction of hot water from the bottom and no recharge. Steam and noncondensable gases above liquidsteam interface providing "steam-drive." Slightlysubcooled reservoir conditions.TABLE 2.2 RESULTS OF ENERGY EXTRACTION EXPERIMENTSExperimentTypeSpecific EnergyExtraction(Btu/ lb,)In-PlaceBoiling36Energy ExtractionFraction(Dimensionless) 0.75Sweep 62 9.80*Steam-Drive 0.229*Based on the steady-state water injection temperature as the lower reference. The others are based on the saturation temperature corresponding to the end pressure.The specific energy extraction (energy extracted per pound of rock)was greatest for the sweep process and smallest for the steam-drive process.The fraction of thermal energy stored in the rock between theinitial temperature and reference lower temperature that was actuallyextracted, referred to as the energy extraction fraction, i s also seento vary widely.The question of which of these energy extraction pro-cesses is practical in large-scale field development will depend on theparticular conditions that prevail at the site.
6The simple analytic models developed for the model reservoir andfor the heat transfer from the rock successfully predicted the experimental results as long as the assumptions inherent in the models werenot seriously violated.However, it was recognized that more detailedexperimental and analytic studies of the heat transfer aspects were required, and such studies have since been performed by Iregui (1978).The final report on these results is in preparation and the highlightsare given below.Rock Heat Transfer StudiesPrediction of heat transfer from a collection of irregularly shapedrocks is complicated because the rocks vary in size and shape. The effectof rock shape was investigated by Kuo, Kruger, and Brigham (1976).Theresults showed that a rock with an irregular shape can be treated analytically as a sphere with equivalent radii used in the Fourier and Biotnumbers determined by a single parameter referred to as the sphericity ofthe rock.The sphericity is defined as the ratio of the surface area ofthe equivalent spherical rock having the same volume to the actual surface area of the rock.Additional work was performed utilizing thisconcept to predict the thermal behavior of a collection of rocks withgiven size distribution and shape for artibrary boundary or cooldownconditions.The basis for the rock temperature transient prediction for a singlerock was the one-lump, spherical solution presented by Hunsbedt, Kruger,and London (1975) for constant cooldown conditions.This solution wasmodified to variable cooldown conditions by superposing constant cooldown rate solutions, a procedure frequently used in heat transfer analyses.The validity of this model was verified by comparing the predictedrock temperature to the measured rock temperature.An illustration ofsuch a comparison is given in Fig. 2.1, where the predicted and measuredtemperatures for instrumented Rock No. 1, located at the bottom of thereservoir model, are shown as functions of time.Another illustrationis given in Fig. 2 for Rock No. 2, located near the center.Two in-placeboiling experiments and one sweep experiment were conducted to providethe data for the comparisons. The rock used in these experiments (thirdrock loading) consisted of granitic rock fragments with an arithmeticmean diameter of 1.62 inches.It was obtained from the piledriverchimney produced by a nuclear explosion at the Nevada Test Site.
7600III/500IIMEASURED UATER TEHP.II’400-.ILW@a23005wLE200.-ROCK I1RADIUS3 . 1 7 INCHESS P E R l C l T Y * 0.92IO0I1O OFIGURE 2 . 1I2I3I4TIME ( H R )C W P A R I S O N OF MEASURED AN0 CALCULATED TEMPERATURES FOR ROCK II600III-I7IMEASURED ROCK TEMP.F//’’’400L.W3005ROCK 12W0,RADIUS 3.9LcFIGURE 2 . 2\ \\bINCHESSPHERICITY 0 . 0200IMEASURED WATER T M P .CALCULATED ROCK T M P .s0SWEEP EXPERIMENTI/500IIII6I5TIHE (HR)C M P A R I S O N OF MEASURED AN0 CALCULATED TEflPERATURES FOR ROCK 12-SWEEP EXPERlMENT
8The results of the temperature transient comparisons showed thatthe one-lump thermal model utilizing an equivalent radius predicts the rocktemperature transients satisfactorily over a wide range of conditionsand is preferred over exact solutions because of its relative simplicity.The transient model for a single rock was subsequently used to formulatean energy extraction model for a collection of rocks with a given sizedistribution.This model was applied to the laboratory system with knownsize distribution and average rock shape.The predicted energy extrac-tion was compared to the measured energy extraction for one experiment.The results showed that the prediction was of the same order as themeasured, but the model verification was not conclusive because of relatively large uncertainties in the measured energy extraction.Furtherwork is needed to assess the uncertainties in the measurements.The energy extraction model was used to determine the sensitivity ofparameters such as mean rock size, average sphericity, cooldown history,rock size distribution, and the dispersion about the mean for hypotheticallarge-scale systems.These parameters will generally not be known pre-cisely and in many cases will have to be assumed.The effect of rocksize distribution and the sphericity are given in Figs. 2.3 and 2 . 4 , respectively, where the rock energy extraction fraction is plotted as afunction of total time to deplete the reservoir.These results show thatthe fraction of energy that can be extracted from the rock decreases whenthe reservoir is produced over a shorter time period.The energy extrac-tion a l s o decreases when the proportion of large rocks increases. Thisis the case when the dispersion about their mean increases or the shapeof distribution changes (e.g., from exponential to normal).The heat transfer model was also used as a basis for formulating amathematical model for rock energy extraction by the cold water sweepprocess in fracture-stimulated reservoirs.It predicts the water tempera-ture profiles that develop in the rock matrix when cold water is rechargedat one end of the reservoir and hot water is produced at the other.Theformulation of this model showed that the energy extraction process wasgoverned by a non-dimensional parameter referred to as the number of . It is defined as the ratio of the water residue timetuto the time constant of a rock with a size corresponding to the meantransfer units ( N
9IIIIIIIIINORMAL D I S T R I B U T I O NDI STRl BUT IONEXPONENTIAL.-STANDARD D E V I A T I O I I31l EFI4EAN ONIII102030I40I50100TIME (YEARS)ENERGY EXTRACTION FRACTIOII AS A FUNCTION OF TOTAL PRODUCTION T I I I E FOR TU0 DIFFERENT ROCK S I Z EFIGURE 2.3DI STRIBUT IONS.IIIIIIIIEOUIVALEHT RADIUS 100 FEET ( A L L SHAPES)STANDARD DEVIATION 31 FEETNOPAAL ROCK S I Z E DISTRIEUTIONS1.00.80.60.4Spherlcltles:1.0III450.20 -II1230.420:70III10203040100PRODUCTION T I M E (YEARS)FIGURE 2.4 ENERGY E X T M C T I O I I FRACTION AS A FUNCTION OF TOTAL PRODUCTION TIME FOR THREE DIFFERENT ROCK SHAPES
10effective rock radius.The mean effective rock radius (Re) is a type ofmean radius for a collection of unequal size rocks that represents theentire rock collection when heat transfer rates are calculated.It isalways greater than the arithmetic mean radius for the collection, butsmaller than the maximum rock size.A sensitivity analysis was performed to determine the effect of thenumber of transfer units parameter on the sweep energy extraction process.The computations were performed for an assumed rock porosity of 10%.Thenumber of transfer units parameter was varied from 5 to 200. The resultsshowed that the energy extraction is very ineffective for the lower N tuvalues (smaller than lo). The physical explanation for this is that thefluid flow through the rock matrix is too fast for effective heat transferto take place.Alternatively, tne rock sizes are too great and the rockLarger Nvalues (greater than 30) resultedtuin slow enough fluid flow through the rock matrix (or rock sizes noneheat transfer rate too slow.small enough) to affect reasonably effective heat transfer.The cold water sweep model was applied to a hypothetical frncturestimulated geothermal reservoir with parameters and conditions listedin Table 2.3.Two energy extraction rates were assumed which corresponded(case 1) and 50 MW (case 2).eeThe water residence times for the two cases were fixed at 30 and 6 years,to nominal net power plant outputs of 10 MWrespectively, for these conditions.Changes in Nvarying the mean effective rock radius Rwere affected bytufrom 25 to 400 feet.eThe produced water temperature and the energy extraction fractionsare given as functions of time in Fig. 5 for the two cases.The effectof rock size (and of N) on the produced water temperature are seen intuthe left-hand diagrams. The water temperature generally drops earlierand at a faster rate for the larger rocks (smaller Ntu) values in bothcases.Moreover, the rock water temperature starts to drop at earlier production times for the higher energy extraction rate of case 2.Theenergy extraction fractions for the two cases seen as functions of timeis the right-hand diagram of Fig. 2.5, are seen to be generally higherfor Case 2.The main reason for this is the generally higher rock-to-watertemperatures as a resu t of the faster cooling of the fluid. A l s o , therock sizes are smaller in Case 2 to achieve comparable N tu values forthe two cases.
11TABLE 2 . 3 : HYPOTHETICAL RESERVOIR PARAMETERSInitial Temperature (OF)550Initial Temperature (OF)703Reservoir Volume (km )Specific Power Plant Flow Rate (lb /kWhr)mPower Generation Rates (MW )eCase 1Case 2185*1050Distance Between Injection Production Wells (km)1Reservoir Porosity (dimensionless)0. 1**Water Residence Time (years)Case 130Case 26Mean Effective Radius (ft)Number of Transfer Units (dimensionless)25-4001-55*Power generation rate is based on average specific steam flow rategiven above.**Reservoir permeability assumed adequate to allow uniform water flowas required rates in both cases.
12hcnn0LA- 6oor-l---lW.- 'pU'*or--l0.80a3n0200-R e 400-(N L O )tuIIIII.FXW (3aWzwTIME (YEARS)1we 1LLIOaaIXW (3TIME (YEARS)wTIME (YEARS)CASE 2 ( 5 0 MW,)FIGURE 2 . 5 PRODUCED WATER TEMPERATURES AND ENERGY EXTRACTION FRACTIONS ASFmCTIONS OF TIME
13The results generally indicate that the energy stored in the rock ofgiven sizes can be extracted effectively when the reservoir is producedat corresponding low rates.If higher energy extraction rates are de-sired, the rock has to be fractured more extensively to achieve anacceptable reservoir life.Further evaluation of the energy extractionprocess will continue in the future.Current ExperimentsThe experiments performed in the SGP reservoir model have utilizedrock systems with porosities between 35 and 44% and essentially infinitehorizontal and vertical permeabilities. Thus, these systems are notvery representative of naturally or artificially fractured geothermalreservoirs where a typical porosity may be in the range of5 to 20% andthe permeabilities in the range of 5 to 500 md.Experiments with a morerepresentative rock system are being conducted.This fourth rock systemconsists of the granitic rock utilized in the third rock system piledriver rock having an average rock size of 1.62 inches), but the voidspaces are filled with 80 to 100 mesh sand.The porosity has beendetermined to be about 21%; the vertical permeability is being measured.Two energy extraction experiments of the in-place type have been conductedwith this rock system. A preliminary evaluation of the results are presented.A summary of experimental conditions and parameters for the twoexperiments (denoted by 4-1 and 4-2) are given in Table 2.4.Additionaltape heater capacity was added prior to conducting experiment 4-2 toprovide higher initial pressure and temperature and a more uniform initialtemperature distribution in the rock matrix relative to that of experiment 4- 1.Measured axial rock and steam/l quid temperature distributions forexperiment 4-2 are given in Fig. 2.6 f o r different times during the production process.The initial temperature distribution (t O) in Fig. 2.6is seen to be uniform in the upper portions, but i s slightly lower thanthe average at the bottom.Additional tape heater capacity may be addednear the bottom to provide essentially uniform initial conditions.
14TABLE 2.4: EXPERIMENTAL CONDITIONS AND PARAMETERS FOR TWO RECENTIN-PLACE BOILING RUNSExperiment No.4-14-2Initial Pressure (psia)3025003915416467356449Average Initial Temperature ( F)405465End Temperature270212195187130189End Pressure (psia)0Initial Temperature - Top ( F)0Initial Temperature - Bottom ( F )0(OF)Initial Mass of Water in Matrix (lbm Total Mass of Steam Produced (lb )mProduction Time (hrs)Mean Steam Production Rate (lbm/hr)External Heat Transfer Parameter (Btu/lbm)l.9 03 .54454172217TABLE 2.5: SUMMARY OF RESULTS FOR TWO RECENT IN-PLACE BOILING EXPERIMENTSExperiment No.4-14-2Specific Energy Extraction (Btu/lb )mEnergy Extraction Fraction (dimensionless)28.943.50.990.82Fraction of Steam Produced (dimensionless)0.671.0Recovery Factor (dimensionless)4.53.8
15-ecSIZEmLARGE.-0anMEDIUM SIZEc0E-.-SMALL RE ( O F )FIGURE 2.6 AXIAL FLUID AND ROCK TEMPERATURE DISTRIBUTIONS AT DIFFERENTPRODUCTION TIMESIISYMBOLROCK CENTER ( T O P )STEAM (TOP)ROCK CENTER (BOTTOM)LIQUID / STEAM ( BOTTOMSATURATION TEMPERATURE000I200;IIDESCRIPTIONI2I34PRODUCTION TIME ( hours)FIGURE2.7 FLUID AND ROCK TEMPERATURE' HISTORIES AT TOP AND BOTTOM O FROCK MATRIX
16The data in Fig. 2.6 indicate that saturated reservoir conditionsare maintained uniformly throughout the reservoir during most of theproduction process.However, near the end, superheated steam conditionsdeveloped indicating that liquid water is being depleted and dryout initiated.The dryout and superheating effect is also noted in Fig. 2 . 7 ,which gives fluid and rock temperatures at the top and bottom of the rockmatrix as functions of time.These data show that the superheating effect started near the top2 . 5 hours and near the bottom at t 3 . 2 5 hours.at 5of the rock matrix had occurred at this time.Complete dryoutThe steam production, seenin Fig. 2 . 8 , leveled off sharply at this time, and the reservoir pressuremeasurements showed a rapid pressure decline.The high temperature zonein the lower half of the rock matrix is believed to be caused by heatingof the steam/rock from the residual energy contained in the high heatcapacitance level flanges located at this elevation.The results for experiment 4- 1 showed no evidence of liquid dryout.Major reasons for this are that the initial temperature and pressure werelower than for experiment 4 - 2 , as indicated in Table 2 . 4 , and the endpressure and temperature were higher.Sufficient energy was not avail-able to evaporate all water in experiment 4 - 1 .A comparison of the specific energy extraction for both experimentsgiven in Table 2.5 show a significantly higher value for experiment 4 - 2 .The energy extraction fraction (proportional to the ratio of areaa-b-c-d-e-a to area a-b-c-d-f-g-a in Fig. 2 . 6 ) w a s lower for experiment4- 2 because of the incomplete energy extraction caused by the dryoutcondition.The energy extraction fractions for these experiments, how-ever, were generally of the same order (or higher) compared to thoseachieved in earlier experiments with higher porosity/permeability systems.The recovery factor defined as the ratio of steam actually producedto that which vould have been produced by flashing the steam alone (withoutenergy from rock or vessel steel) is seen to be 4 . 5 and 3.8 for experiments4- 1 and 4 - 2 , respectively.This is significantly higher than that achievedin earlier experiments with higher porosity/permeability systems wherethe maximum recovery factor was 2 . 5 8 (Hunsbedt, Kruger, and London, 1975,1977).
1720cIII2315C0IPRODUCTION TIME (HOURS)FIGURE 2 . 8CUMMULATIVE MASS PRODUCTION KISTORY4
18The overall conclusion of the preliminary data evaluation is thatenergy recovery is improved for the present system relative to the otherhigh porosity/permeability systems tested earlier.The natural convec-tion and cooling of the rock is not impeded by the presence of sand inthe voids of the laboratory model system.Further evaluations areneeded to determine to what point the permeability can be lowered withoutsignificant deterioration of the energy extraction effectiveness.2.2Thermal Fracturing ExperimentsThe utilization of energy stored in a hot dry geothermal rockgreatly depends on the ability to extract it from the rock.The tech-nique of hydraulic fracturing creates long vertical cracks in the rockthrough which water can be circulated to extract the thermal energy.There has been a speculation that cooling of rocks would produce thermalstresses causing the rocks to crack further and thus provide new surfacearea for heat transfer.Pracht and Harlow (1972) have shown theoreticallythat if thermal cracking does happen, the life of the geothermal reservoiris greatly enhanced.This work is the first part of the project whichinvolves the verification of thermal stress cracking experimentally.Physical ModelA long vertical crack formed by hydraulic fracturing can be repre-sented as shown in Fig. 2.9.A s the temperature in the interior of therock does not change with time, the temperature distribution near thesurface can be determined by treating the rock as a semi-infinite solid.The above situation can also be realized for a slab of finite thicknesswhich is insulated around its circumference (Fig. 2.10).The finite slabcan be considered as a semi-infinite body as long as the temperaturedisturbance is not felt at the x b face.Hence, to determine the effectof thermal stressing in actual systems, we would be doing an experimenton a finite slab (Fig. 2.10) which is preheated to a specified temperatureand then quenched on the x O face by water while keeping other facesinsulated.
19TooTiFLUIDFIGURE 2.9SCHEMATIC O F LONG VERTICAL CRACK FORMED BY HYDRAULICFRACTURINGI NSUL AT EDTooFLUIDIb--IFIGURE 2.10EWERIMENTAL EQUIVALENT-FINITE LAB
20Heat Transfer AnalysisThe temperature distribution in the slab for a finite value of thesurface conductance at the face is given by:Ti-T(x, t) T 1.-Too1 - erfX[3-2J Zexp2at2khx[ i;T h----esSwhere :h heat transfer coefficientkS thermal conductivity of rockkS - thermal diffusivity of the rocka fCt time (hrs)T temperatureSince the rock temperature would be of the order of 400 F, nucleatedboiling can be expected to occur on the surface of the body and thus result in a high heat transfer.The heat transfer coefficient in such a2 0case would be of the order of 1,000 Btu/hr-ft - F. It can be shown thatcorresponding t o such high heat transfer coefficients, the last term inE q . 2.1 becomes imignificant and can be neglected for all practicalpurposes.Thermal Stress AnalysisThe free thermal contraction of elements in the y and z directionswould result in thermal stresses in these directions.These thermalstresses are given by:-[l - erf(b/a)]--(ah)(a/b) e-(b/a)2 Ji; 2 ("I22berf(b/a)
21Crack Initiation and PropagationThe crack would be initiated when the thermal stress reaches thetensile strength of the rock at some point in the material.From Eq.2.2, the maximum tensile stress occurs at x O, and is given by:For tensile strength (a) of 1100 psi (granite), the temperature differtentia1 required for crack initiation would be:ei 1100(1-v)EatTypical values of v, E, and a for granite are 0.31, 8x106 psi, andt4 . 0 1 0- 6f oF.Using these values, we get 8 - 2 4 0 F for crack initiation.iOnce the crack is initiated, it would extend normal to the plane ofmaximum principal stress, i.e., x-direction provided there is enoughdriving force available.The stresses reduce because of stress
The Stanford Geothermal Program is a mobile and expansive group that fulfills other secondary roles in the geothermal community. Other than filling the University's task of providing training for new geo- thermal personnel, the program has provided several organized services to the nearby geothermal concerns. The Annual Stanford Geothermal