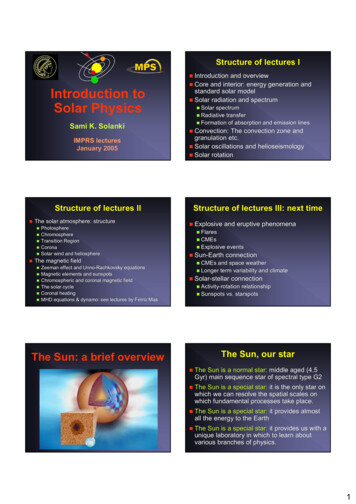
Transcription
Structure of lectures I IntroductionIntroduction toSolar PhysicsSami K. SolankiIMPRS lecturesJanuary 2005Structure of lectures II The solar atmosphere: structure PhotosphereChromosphereTransition RegionCoronaSolar wind and heliosphereThe magnetic field Zeeman effect and UnnoUnno-Rachkovsky equationsMagnetic elements and sunspotsChromospheric and coronal magnetic fieldThe solar cycleCoronal heatingMHD equations & dynamo: see lectures by Ferriz Masand overview Core and interior: energy generation andstandard solar model Solar radiation and spectrum Solarspectrumtransfer Formation of absorption and emission lines Radiative Convection:The convection zone andgranulation etc. Solar oscillations and helioseismology Solar rotationStructure of lectures III: next time Explosiveand eruptive phenomena Flares CMEs Explosive SunSun-Eartheventsconnection CMEsand space weather Longer term variability and climate SolarSolar-stellarconnection ActivityActivity-rotation Sunspotsrelationshipvs. starspotsThe Sun, our starThe Sun: a brief overview TheSun is a normal star: middle aged (4.5Gyr)Gyr) main sequence star of spectral type G2 TheSun is a special star: it is the only star onwhich we can resolve the spatial scales onwhich fundamental processes take place. TheSun is a special star: it provides almostall the energy to the Earth TheSun is a special star: it provides us with aunique laboratory in which to learn aboutvarious branches of physics.1
The Sun: a few numbersThe Sun: Overview The Sun’s StructureSolar interior: Everything belowthe Sun’s (optical)surfaceDivided intohydrogenhydrogen-burningcore, radiative andconvective zonesThe solar surface Since solar material does not exhibit a phase transition (e.g.from solid or liquid to gaseous as for the Earth), a standardway to define the solar surface is through its radiation. The photons travelling from the core outwards make arandom walk, since they are repeatedly absorbed andreemitted. The mean free path increases rapidly with radialdistance from the solar core (as the density and opacitydecrease). A point is reached where the average mean free pathbecomes so large that the photons escape from the Sun.This point is defined as the solar surface. It corresponds tooptical depth τ 1. Its height depends on λ. Often τ 1 at λ 5000 Å is used as a standard for the solarsurface.Solar atmosphere: Directly observablepart of the Sun.Divided eliosphereMass 1.99 1030 kg ( 1 M )Average density 1.4 g/cm3Luminosity 3.84 1026 W ( 1 L )Effective temperature 5777 K (G2 V)Core temperature 15 106 KSurface gravitational acceleration g 274 m/s2Age 4.55 10 9 years (from meteorite isotopes)Radius 6.96 105 kmDistance 1 AU 1.496 ( /( /-0.025) 108 km1 arc sec 722 722 12 km on solar surface (ellipticalEarth orbit)Rotation period 27 days at equator (sidereal, i.e.as seen from Earth; Carrington rotation)Wide range of physical parameters The Sun presents a wide variety of physical phenomena andprocesses, between solar core and corona.E.g. Gas density varies by 30 orders of magnitude,temperature by 4 orders, relevant time scales from 10-10 secto 10 GyrSolar physics inrelation to otherbranches of physicsÎDifferent observational and theoretical techniques needed tostudy different parts of Sun, e.g. helioseismology & nuclearphysics for interior, polarimetry & MHD for magnetism, etc.2
Solar Physics in Relation to Other FieldsThe Sun as a plasma physics lab.Sun-Earth relations:climate, space weatherCosmic rays, localinterstellar mediumTurbulence,dynamosSolar planets,extrasolar planetsPlasma physicsSunCool stars: activity,structure & evolutionAtomic/molecularphysicsFundamental physics:Neutrinos, GravitationSolar Tests of Gravitational Physics Curved light path in solar gravitational field Î Testof General Relativity Red shift of solar spectral lines Î Test of EEP Oblate shape of Sun Î Quadrupol moment of solargravitational field: Test of BransBrans-Dicke theory (R.Mecheri)Mecheri) Comparison of solar evolution models withobservations Î Limits on evolution of fundamentalconstants Polarization of solar spectral lines: Testsgravitational birefringence Î Tests of equivalenceprinciple & alternative theories of gravity (O. Preuss)Preuss)The Sun and particle physics Phenomena happening all over the universe can bebest studied at close distances, where the relevantphysical processes can be spatially resolved (samefor solar planets).E.g. magnetic activity, is present on innumerablestars, in accretion disks, in jets, in the interstellarmedium, etc., but the relevant spatial scales cangenerally not be resolved. The Sun provides a key.Radio astronomy, radiative transfer,spectropolarimetry,spectropolarimetry, asteroseismolgy,asteroseismolgy, etc. aretechniques first developed to study the SunStandard solar model is wrongNeutrino physics is incompleteRecent findings from SNO and Superkamiokande:Superkamiokande:Problem lies with the neutrino physicsÎStandard model of particle physics needs to berevised Nobel prize 2002 for R. Davies for discovery of thesolar neutrino problem. Which stars have magnetic fieldsor show magnetic activity?Solar physics and astrophysics The fact that the rate of neutrinos measured by theHomestake 37Cl detector is only 1/3 of that predictedby standard solar models was for 30 years one ofthe major unsolved problems of physics.Possible resolutions: Best studied star: SunF, G, K & M stars (outerconvection zones) showmagnetic activity & have B fields of GG-kG.kG.Early type stars: Ap,Ap, Bp,(kGkG-100kG), Be (100G)White dwarfs have B kGkG-109 G, no activityNot on diagram: pulsars3
Sun, Earth and planetsThe Sun compared with active stars Solar output affects the magnetospheres andatmospheres of planetsSolar energy isresponsible forproviding ahabitableenvironmenton EarthSolar evolutionand liquid wateron Mars.The Sun’s core The solar interior In the Sun’s core mass isturned into energy.Nuclear reactions burn7x1011 kg/s of hydrogen intohelium.Inside the core the particledensity and temperature areso high, that individualprotons ram into each otherat sufficient speed toovercome the Coulombbarrier, formingheavier He atomsand releasingenergyNuclear reactions of pp-chainNuclear reactions in cores of stars Sun gains practically all its energy from the reaction4p α 2e 2ν2ν 4He 2e 2ν2ν Two basic routes p-p chain: yields about 99% of energy in Sun CNO cycle : 1% of energy released in present day Sun(but dominant form of energy release in hotter stars) Both chains yield a total energy Q of 26.7 MeV,MeV,mainly in the form of γ-radiation Qγ (which isabsorbed and heats the gas) and neutrinos Qν(which escapes from the Sun). p protond deuteriumα Heliumγ radiationν neutrino2nd reactionreplacesstep 3 of1st reaction3rd reaction replaces steps 2 3 of 2nd reactionBranching ratios: 1st vs. 2nd 3rd 87 : 132nd vs. 3rd 13 : 0.0154
Temperature dependence of ppchain and CNO cycleNuclear reactions of CNO-cycle C,N and O act only as catalysts: Basicallythe same things happens as with protonchain. Solar neutrinos Neutrinos, ν, are produced at various stages of the pppp-chain. Neutrinos are also produced by the reaction: p(p e- , ν)d , sosocalled pep reaction. Being a 33-body reaction it is too rare tocontribute to the energy, but does contribute to the numberof ν.Solar neutrino spectrum Solar neutrinos II Since 1968 the Homestake 37Cl experiment hasgiven a value of 2.1 0.3 snu (1snu 1 ν / 1036target atoms) Standard solar models predict: 7 7 2 snup-p chain incool mainmainsequencestarsCNO cycle inhot mainmainsequencestarsTriple alphaprocess in redgiants: 3He CContinua:number/(cm2 sMeV)MeV)Lines:number/(cm2s)Bars at top &shading:sensitivity ofdifferentmaterials to νResults of various neutrinoexperimentsÎSolar Neutrino Problem! In 1980s & 90s water based Kamiokande and largerSuperkamiokande detectors found thatapproximately half the rare, high energy 8B ν weremissing. 71Gaexperiments (GALLEX at Gran Sasso andSAGE in Russia) showed that the neutrino flux wastoo low, even including the p(p,e ν)d neutrinos.5
Solarneutrinos IIISensitivity of H2O,71Ga and 37Clto ν increases exponentially withincreasing energy.ÎHomestake 37Cl detector and (Super(Super-) Kamiokandesee mainly highhigh-energy ν from rare β -decay of 8B. Branching ratios between the various chains: centralfor predicting exact ν-flux detectable by 37Cl & H2O Branching ratios depend very sensitively on T(r 0),while total ν-flux depends only linearly on luminosity. Even 71Ga experiments sensitve largely to highenergy ν. Resolution of neutrino problemSNO (Sudbury Neutrino Observatory) in Sudbury,Canada uses D2O and can detect not just theelectron neutrino, but also µ and τ neutrinosÎ The neutrinos aren’aren’t missing, e- neutrinos producedin the Sun just convert into µ and τ neutrinosÎ The problem lies with the neutrino physics. The neutrino has a small rest mass (10-8 me), whichallows it to oscillate between the three flavours: e neutrino, µ neutrino and τ neutrino (proposed 1969by russian theorists: Bruno Pontecorvo and VladimirGribov,Gribov, but nobody believed them) Confirmation by measuring antianti-neutrinos frompower plant (with Superkamiokande).Superkamiokande). Standard solar model Ingredients: Conservation laws and material dependentequations Mass conservationHydrostatic equilibrium ( momentum conservation in a steady state)state)Energy conservationEnergy transportEquation of stateExpression for entropyNuclear reaction networks and reaction rates energy productionOpacityAssumptions: standard abundances, no mixing in core or inradiative zone, hydrostatic equilibrium, i.e. model passesthrough a stage of equilibria (the only time dependence isintroduced by the reduction of H and the build up of He in thecore).Solar Neutrinos IV Possiblesolutions to solar neutrino problem: Standardsolar model is incorrect (5(5-10% lowertemperature in core gives neutrino flux consistentwith Homestake detector). Neutrino physics is incomplete (i.e. the standardmodel of particle physics is wrong!) Nuclear physics describing the pppp-chain isincorrect Nuclear physics describing interaction betweenneutrino and 37Cl is incorrect (Kamiokande(Kamiokande &71Ga showed that this wasn’t the problem)Resolution of neutrino problem II Lessonlearnt: neutrinos have a multiplepersonality problem (J. Bahcall)Bahcall) Other lesson learnt: the “dirty” and difficultsolar model turned out to be correct, theclean and beautiful standard theory of particlephysics turned out to be wrong, or at leastincomplete (J. Bahcall)Bahcall) 2002: Raymond Davis got Nobel prize foruncovering the neutrino problemEquations describing solar interiorMass conservation : r1 m 4πρr 2ρ gas densityHydrostatic equilibrium :Gm P m4πr 2P pressureG Gravitational constantEquation of state :ρ ρ ( P,T )or (for an ideal gas) :ρ ℜTPG µPG gas pressureℜ gas constantµ mean molecular weightEnergy tra nsport :Energy balance :F FR FC Fcond L S ε T m Tε energy generation per unit massT temperatureF total energy fluxS entropyL luminosityL4πr 2FR radiative fluxFC convective fluxFcond conductive flux6
Internal structure of the Sun Internalmodelsshown forZAMS Sun(subscriptz) and forpresent daySun (radiusreachingout to 1.0,subscript )Solar evolution Path of the Sun in theHR diagram, startingin PMS stage andending at Red giant stageWhite dwarf stageNote the complexpatch during the redgiant phase due tovarious phases ofHelium burning andcore contraction andexpansion, etc.η mass loss rateFaint young Sun paradoxEvolution of Sun’s luminosity Accordingto the standard solar model theSun was approximately 30% less bright atbirth than it is todayZAMS Today Toofaint to keep the Earth free of ice! Problem:Albedo of ice is so high that evenwith its current luminosity the Sun would notbe able to melt all the ice away. Obviously the So:Possible resolution of the faintyoung Sun paradox The Earth’s atmosphere was different 4 Gyr ago. Moremethane and other greenhouse gases. Higher insulationmeant that even with lower solar input the Earth remainediceice-free. As the Sun grew brighter life grew more abundant andchanged the atmosphere of the Earth, reducing thegreenhouse effect. Problem: what about Mars? Could it have had liquid water4Gyr ago if Sun were so faint? Alternative: Sun was slightly more massive (1.04(1.04-1.07M atbirth and lost this mass (enhanced solar wind) in the courseof time (Sackmann(Sackmann & Boothroyd 2003, ApJ).ApJ). A more massivestar on the ZAMS emits more light. Also agrees w. Mars dataEarth is not covered with ice.Where is the mistake?Evolution of solar luminosityRunaway greenhouse effectthrough evaporation of oceansTodaySackmann et al. 1997The future of the Earth?7
The Sun in white light: Limb darkeningSolar radiation andspectrumLimb darkening vs. λ Upperfig.:λ: largelimb darkening; long λ: smalllimb darkening In the visible, theSun’s limb is darkerthan the centre ofthe solar disk (Limbdarkening) Since intensity Planck function,Bν(T), T is lowernear limb. Due to grazingincidence we seehigher near limb: Tdecreases outwardThe Sun in the EUV: Limb brightening short departurefromstraight line:limb darkeningis morecomplex thanI(θ) cos(cos(θ)The Sun in the EUV: Limb brightening Limbbrighteningin optically thinlines does notimply that theSun’stemperatureincreasesoutwards(although bychance it does inthese layers.) In the EUV, the Sun’slimb is brighter thanthe centre of the solardisk (Limbbrightening)Since the solaratmosphere isoptically thin at thesewavelengths, intensity thickness of layercontributing to it. Dueto geometrical effectsthis layer appearsthicker near limb(radiation comes fromroughly the sameheight everywhere).C IVSolar irradiance spectrumIrradiance solar flux at 1AUSpectrum issimilar to, butnot equal toPlanck functionÎ Radiationcomes fromlayers with diff.temperatures.Often usedtemperaturemeasure for stars: Effective temp: σT4eff Area underflux curve8
Absorption in the Earth’satmosphereThe solar spectrum: continua withabsorption and emission lines The solar spectrum changes in character at differentwavelengths.X-rays: Emission lines of highly ionized speciesEUV: Emission lines of neutral to multiply ionizedspecies plus recombination continuaUV: stronger recombination continua and absorptionlinesVisible: H- b-f continuum with absorption linesFIR: H- f-f continuum, increasingly cleaner (i.e. lesslines, except molecular bands)Radio: thermal and, increasingly, nonnon-thermalcontinuaSolar UV spectrumVisibleNote the transition from absorption lines (for λ 2000Å)to emission lines (for λ 2000Å)EUV spectrum Detail of EUV spectrum by SUMERThe solar spectrum from 500 Å to 1600 Å measuredby SUMER (logarithmic scale)Activitysensitiveline:S VILymancontinuumLy αλ (Å) 80010001200Lyman continuum edge140016009
Solar EUV irradianceFormation of the solar spectrum Continuum Spectralenergy distribution Centre to limb variation Spectrallines Absorption Emission RadiativeRadiative transfer: optical depth Axis zpoints in the direction of lightpropagation Optical depth: τν -κν zwhere κν is the absorption coefficient and ν isthe frequency of the radiation. Light onlyknows about the τν scale and is unaware of zÎIntegration:τν - κν(z)dIntegration:(z)dz(note that the scales are floating, no constantof integration is fixed)Height of τ 1, brightnesstemperature and opacity vs. λlineslinestransferOptical depth and solar surface Radiationescaping from the Sun is emittedmainly at values of τν 1. At wavelengths at which κν is larger, theradiation comes from higher layers in theatmosphere. In solar atmosphere κν is small in visible andnear IR, but large in UV and FIR Î We seedeepest in visible and NIR, but sample higherlayers at shorter and longer wavelengths.Radiative Transfer EquationEquation of radiative transfer:µ dIν/dτ/dτν Iν – Sν1.6µmwhere Iν is the intensity (i.e. the measured quantity)and Sν is the source function. µ cosθcosθ is onlyimportant for nonnon-vertical rays.Sν emissivity εν divided by absorption coefficient κνThe physics is hidden in εν and κν , i.e. in τν and Sν .These quantities depend on temperature, pressure,elemental abundances, and frequency orwavelength10
When is an emission line formed,when an absorption line?Formal solution of RT equationFor Sν 0 the solution of the RTE in a slab with(optical) boundaries τ ν2 and τν1 is :Iν(τν2) Iν(τν1) exp(exp(– τν2 τν1 ) For general case formal solution reads (formal soln.soln.assumes that we already know Sν )Iν(τν2) Iν(τν1) exp(exp(– τν2 τν1 ) Sν exp(exp(– τν) dτν 1st term describes radiation that enters throughlower boundary (only absorption, no emission inslab), 2nd term describes radiation emitted in slab. In a stellar atmosphere τν1 , so that only the 2ndterm survives (lower boundary is unimportant). Continuaare formed deeper in a stellaratmosphere than spectral lines at the samewavelengths. A line is in absorption if Sν decreases withheight, i.e. if the absorption at greater heightsdominates over emission A line is in emission if Sν increases withheight, i.e. if the emission at greater heightsdominates over absorptionStatistical equilibriumThe assumption of LTE Ingeneral both Sν and κν require acomputation of the full statistical equilibriumfor the species being considered. This implies computing how much eachatomic/ionic/molecular level is populated, i.e.solving rate equations describing transitionsto and from each considered level. Requires detailed knowledge of atomic, ionic,molecular structure and transitions. Elementalabundances Photospheric valuesLogarithmic (to base 10)abundances of the 32 lightestelements on a scale on whichH has an abundance of 12Heavier elements all have lowabundancesNote that in general the solarphotospheric abundances arevery similar to those ofmeteorites, with exception ofLi, with is depleted by a factorof 100.In the solar interior and photosphere (i.e. wheredensity is large and collisions are common) we canassume Local Thermodynamic Equilibrium (LTE)Thermodynamic equilibrium (TE): a single(TE):temperature everywhere (blackbody) Î Radiationemerging from object follows the Planck function Bν .LTE:LTE: Each layer of the solar atmosphere has its owntemperature Î Replace Sν by Bν(T) in the RTE andits solution.Problem of knowing Sν is reduced to knowing T(τT(τ) inthe atmosphere.In addition, the statistical equilibrium can be solvedsimply by considering the SahaSaha-Boltzmannequilibrium Î basically T and ne need to be known.Elemental abundances: the FIPeffect In TR and coronaabundances differ fromphotospheric valuesdepending on the firstionization potential (FIP)of element.Elements with low FIPhave enhancedabundanceApparently, accelerationthrough the chromosphereis more efficient for theseelements (which areionized in chromosphere,chromosphere,while high FIP elementsare not).11
Spectrum synthesis by 1-Dradiative equilibrium modelDiagnostic power of spectral lines Dopplershift of line: (net) flows in the LOSdirection. Line width: temperature and turbulent velocity Equivalent width: elemental abundance,temperature (via ionisation and excitationbalance) Line depth: temperature and temperaturegradient Line asymmetry: inhomogenieties in the solaratmosphere.Heights of formation: on whichlayers do lines give information ?UV, neutral EUV andstrong visible linesSolar convection“normal”linesin visibleMultiply ionizedEUV linesThe convection zone Through the outermost 30% of solar interior, energyis transported by convection instead of by radiation In this layer the gas is convectively unstable. Theunstable region ends just below the solar surface.I.e. the visible signs of convection are actually dueto overshooting. Due to this, the time scale changes from the timescale for a random walk of the photons through theradiative zone (due to high density, the mean freepath in the core is well below a millimeter)millimeter) to theconvective transport time:t radiative 106years tconvective monthsScales of solar convection Observations: 4 mainscales Colour:Colour: granulationmesogranulationsupergranulationgiant cellswell observedless strong evidenceTheory: larger scalesat greater depths. Indetail a lot is unclear.12
Surface convection:SupergranulationSurface convection: granulation Typical size: 2 Mm Lifetime: 6-8 min Velocities: 1 km/s (butpeak velocities 10km/s, i.e. supersonic) Brightness contrast:20% in visiblecontinuum (underideal conditions)All quantities show acontinuousdistribution of values 106At any one timegranules on sun. Observing convection Continuum images and movies at high spatial resolution:gives sizes, lifetimes and evolution (splitting anddissolving granules), contrastsSpectral lines (also at low spatial resolution). Linebisectors, line widths and convective blue shifts:contrasts, area factors, stratificationObserving supergranulation Images and movies in cores of chromospheric spectrallines, or magnetograms:magnetograms: observe the magnetic field at theedges of the supergranules instead of the supergranulesdirectlyHelioseismic techniquesSupergranules seen by SUMER Line bisectorsObserving granulation 1 hour average ofMDI Dopplergrams(averages outoscillations).DarkDark-bright: flowstowards/away fromobserver.No supergranulesvisible at disk centre:centre:velocity is mainlyhorizontalSize:Size: 2020-30 Mm,lifetime:lifetime: days,horiz.horiz. speed:speed: 400 m/s,no contrast in visibleSi I 1256 Å fulldisk scan bySUMER in 1996Bright networkindicates locationof magneticnetworkDarker cells:supergranulesSupergranules& magneticfield Why are supersupergranules seen inchromospheric andtransition regionlines?Supergranules arerelated to themagnetic network.Network magneticfields areconcentrated atedges ofsupergranules.supergranules.13
Illustration of convectively stableand unstable situationsConvectively stableConvectively unstableOnset of convectionSchwarzschild’s instability criterionConsider a rising bubble of gas:ρ*ρz- z zρρ0 ρbubblesurroundingsdepthCondition for convective instability: ρ* ρ0For small z, bubble will not have time to exhange heat withsurroundings: adiabatic behaviour. Convectively unstable if:[dρ/dz – (dρ(dρ/dz)dz)adiab] z 0dρ/dz : true stellar density gradient,(dρ(dρ/dz)dz)adiab : adiabatic gradientOnset of convection II ad (d log T/d log P) adrad (d log T/d log P) rad gradient in anatmosphere with radiative energy transportSchwarzschild’Schwarzschild’ s convective instability criterion: adradWhy an outer convection zone? Why does radiative grad exceed adiabatic gradient?Mainly: radiative gradient becomes very large dueto ionization of H and He below the solar surface.Expression for radiative gradient (for Eddingtonapproximation): Rewriting in terms of temperature and pressure:rad Ionisation of H and He Ionisation balance is described by Saha’s equation:degree of ionisation depends on T and ne H ionisation happens just below solar surface He He e- happens 7000 km below surface He He e- happens 30’30’000 km below surface Since H is most abundant, it provides mostelectrons (largest opacity) and drives convectionmost strongly At still greater depth, other elements also provide aminor contribution. (3Fr /16σ/16σg) (κ(κgr Pg / T4 )Fr radiative flux ( ( constant)σ StefanStefan-Boltzmann constantg gravitational acceleration ( ( constant)κgr absorption coefficient per gram. As H and Hebecome ionized with depth, κgr increases rapidly, leadingto large radiative gradient.Radiative, adiabatic & actualgradients14
The mixing length As a gas packet rises, diffusion ofparticles and thermal exchangewith surroundings causes it to loseits identity and to stop from movingon.Length travelled up to that point:mixing length l.Often used parameterizationparameterization of l:l α Hp Why is mixing length interesting Givesan idea of the size scale of aconvection cell (height or depth of cell givenby mixing length) horizontal extent of cell cannot be muchbigger than mixing length due to massconservation!Hp pressure scale heightα mixing length parameter, typically1-2 (determined empirically)Convective overshootHistory of mixing length Mixinglength was introduced by L. Prandl,Prandl,who was director at Max Planck Institut fürStröStrömungsforschung in Göttingen.ttingen. It allowed him to describe convection in asimple, but powerful way. Even today, most stellar interior models (andmany atmospheric models) use the mixinglength to describe the effects of convection.Due to their inertia, the packets of gas reaching theboundary of the CZ pass into the convectively stablelayers, where they are braked & finally stopped.Îovershooting convection Typical width of overshoot layer: order of Hp This happens at both the bottom and topboundaries of the CZ and is important: Convection simulations IIConvection simulations 3-D hydrodynamic simulations reproduce a number ofobservations and provide new insights into solar convection. These codes solve for mass conservation, momentumconservation (force balance, NavierNavier-Stokes equation), andenergy conservation including as many terms as possible. Problem: Simulations can only cover 22-3 orders of magnitudein length scale (due to limitations in computing power), whilethe physical processes on the Sun act over at least 6 ordersof magnitude. Also, simulations can only cover a part of the size scale ofsolar convection, either granulation, supergranulation,supergranulation, orlarger scales, but not all.top boundary: Granulation is overshooting material. Hp 100 km in photospherebottom boundary: the overshoot layer allows BB-field to bestored seat of the dynamo? Simulations do not achieve the solar Reynolds number (R(Re vl/ν) of 1010 , where v typical velocity, l typical lengthscale, ν kinematic viscosity (R(Re ratio of viscous toadvection time scales). For comparison with observations it is important that thesimulations describe the surface layers well, i.e. code needsto consider: radiative transport of energy. Only few simulation codes do thisproperly. partial ionization of many elements as low a viscosity as numerically possibleThe role of radiation is primarily to transport energy. At thesolar surface the energy transported by radiation becomescomparable to that by convection.15
6 MmSimulations of solar granulationSolution of NavierNavier-Stokes equation etc. describing fluiddynamics in a box (6000 km2 x 1400 km) containing thesolar surface. Realistic looking granulation is formed.GranulestructureUpflows are broad &slow, downflows arenarrow and fast.Why?Testing thesimulationsComparisonbetween observedand computedbisectors forselected spectrallines (2 computedbisectors shown:one each with andwithout oscillationsin the atmosphere)Granule evolution Granules die in two ways: Granules are born in two ways: Granule evolution IIWhy do large granules split and small granules getsqueezed out of sight? Granules are overshooting convection structures,with an upflow in their centre, a radial horizontal flowover the whole granule and a downflow in thesurrounding lanes. The upflow builds up density and excess pressureabove the granule Î pressure gradient relative toflanks of granule. Pressure gradient force.ÎGas is accelerated sideways. Above intergranular lane: horizontally flowing gasmeets gas from opposite granule Î build uppressure excess which decelerates flow. dissolve: grow fainter and smaller until they disappear(small granules)split: break into two smaller granules (large granules)as fragments of a large splitting granuleappearing as small structures and growingInitially most granules grow in size, some keepgrowing until they become unstable (see next slide)and split, others stop growing and start shrinkinguntil they disappear (all within 55-10 min).Granule evolution III Considermass conservation: A larger granulewill build up a larger pressure above its centrebecause more mass needs to be acceleratedhorizontally. At some point the pressure becomes so largethat the upflow is quenched. The centre of thegranule cools and a new downflow lane formsthere. The granule splits16
Relation between granules andsupergranules Downflows of granules keep going down to bottomof simulations. However, the intergranular lanesbreak up into individual narrow downflows.downflows.I.e. topology of flow reverses with depth: Increasing size of convective cellswith depthAt surface: isolated upflows,upflows, connected downflowsAt depth: connected upflows,upflows, isolated downflowsIdea put forward by Spruit et al. 1990: Atincreasingly greater depth the narrow downflowsfrom different granules merge, forming a larger andless finefine-meshed network that outlines thesupergranules.supergranules.Convection on other starsF, G, K & M starsposses outer convectionzones and showobservable effects ofconvection (also WDs)WDs) Observations aredifficult since surfacescannot be resolved.ÎUse line bisectors:independent of spatialresolution A,F stars show inversebisectors: granulationhas different geometry. Oscillations andhelioseismology5-minute oscillationsHear the Sun sing! The entire Sun vibrates froma complex pattern of acousticwaves, with a period ofaround 5 minutesThe oscillations are best seenas Doppler shifts of spectrallines, but also as intensityvariations.Identified as acoustic waves,called pp-modesSpatioSpatio-temporal properties ofoscillations best revealed by3-D Fourier transforms.Solar Eigenmodes The pp-modes show adistinctive dispersionrelation (k(k-ω diagram:k ω2) Important: there is poweronly in certain ridges, i.e.for a given k2 ( k x2 ky2),only certain frequenciescontain power.This disc
1 Introduction to Solar Physics Sami K. Solanki IMPRS lectures January 2005 Structure of lectures I Introduction and overview Core and interior: energy generation and standard solar model Solar radiation and spectrum Solar spectrum Radiative transfer Formation of absorption and emission lines Convection: The convection zone and granulation etc.