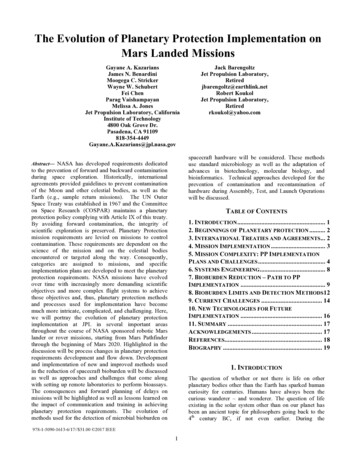
Transcription
The Evolution of Planetary Protection Implementation onMars Landed MissionsGayane A. KazariansJames N. BenardiniMoogega C. StrickerWayne W. SchubertFei ChenParag VaishampayanMelissa A. JonesJet Propulsion Laboratory, CaliforniaInstitute of Technology4800 Oak Grove Dr.Pasadena, CA ck BarengoltzJet Propulsion Laboratory,Retiredjbarengoltz@earthlink.netRobert KoukolJet Propulsion Laboratory,Retiredrkoukol@yahoo.comspacecraft hardware will be considered. These methodsuse standard microbiology as well as the adaptation ofadvances in biotechnology, molecular biology, andbioinformatics. Technical approaches developed for theprevention of contamination and recontamination ofhardware during Assembly, Test, and Launch Operationswill be discussed.Abstract— NASA has developed requirements dedicatedto the prevention of forward and backward contaminationduring space exploration. Historically, internationalagreements provided guidelines to prevent contaminationof the Moon and other celestial bodies, as well as theEarth (e.g., sample return missions). The UN OuterSpace Treaty was established in 1967 and the Committeeon Space Research (COSPAR) maintains a planetaryprotection policy complying with Article IX of this treaty.By avoiding forward contamination, the integrity ofscientific exploration is preserved. Planetary Protectionmission requirements are levied on missions to controlcontamination. These requirements are dependent on thescience of the mission and on the celestial bodiesencountered or targeted along the way. Consequently,categories are assigned to missions, and specificimplementation plans are developed to meet the planetaryprotection requirements. NASA missions have evolvedover time with increasingly more demanding scientificobjectives and more complex flight systems to achievethose objectives and, thus, planetary protection methodsand processes used for implementation have becomemuch more intricate, complicated, and challenging. Here,we will portray the evolution of planetary protectionimplementation at JPL in several important areasthroughout the course of NASA sponsored robotic Marslander or rover missions, starting from Mars Pathfinderthrough the beginning of Mars 2020. Highlighted in thediscussion will be process changes in planetary protectionrequirements development and flow down. Developmentand implementation of new and improved methods usedin the reduction of spacecraft bioburden will be discussedas well as approaches and challenges that come alongwith setting up remote laboratories to perform bioassays.The consequences and forward planning of delays onmissions will be highlighted as well as lessons learned onthe impact of communication and training in achievingplanetary protection requirements. The evolution ofmethods used for the detection of microbial bioburden onTABLE OF CONTENTS1. INTRODUCTION . 12. BEGINNINGS OF PLANETARY PROTECTION . 23. INTERNATIONAL TREATIES AND AGREEMENTS. 24. MISSION IMPLEMENTATION . 35. MISSION COMPLEXITY: PP IMPLEMENTATIONPLANS AND CHALLENGES . 46. SYSTEMS ENGINEERING . 87. BIOBURDEN REDUCTION – PATH TO PPIMPLEMENTATION . 98. BIOBURDEN LIMITS AND DETECTION METHODS 129. CURRENT CHALLENGES . 1410. NEW TECHNOLOGIES FOR FUTUREIMPLEMENTATION . 1611. SUMMARY . 17ACKNOWLEDGEMENTS . 17REFERENCES. 18BIOGRAPHY . 191. INTRODUCTIONThe question of whether or not there is life on otherplanetary bodies other than the Earth has sparked humancuriosity for centuries. Humans have always been thecurious wanderer – and wonderer. The question of lifeexisting in the solar system other than on our planet hasbeen an ancient topic for philosophers going back to the4th century BC, if not even earlier. During the978-1-5090-1613-6/17// 31.00 2017 IEEE1
Renaissance, when Galileo made significant discoveriesand confirmed planets orbit the Sun, the question ofextraterrestrial life became directly relevant to astronomy.1957 by the Soviet Space Program. These dual successfullaunches heralded the beginnings of a new era nces for the balance in the Cold War. Inresponse, the United States Congress – in addition to themilitary establishment and the American public itself –saw the urgent need for scientific and technologicaladvancements to match the Soviets. The conquest ofspace became a new Cold War imperative for the U.S.,bringing with it concerns about planetary protection.As human exploration spread to the “New World” startingin the 15th century, a race to map and dominate the worldwas begun, contributing to the interaction and transfer oforganisms from one land mass to another. Although thiswas not intentional, the consequences of forwardcontamination usually had catastrophic consequences onthe local ecosystem, which had existed untouched up tothis point. As an example, entire populations ofendogenous natives were almost lost in the Americas asan outcome of not having an immune system resistant tosmallpox, which was brought along with explorers fromEurope. Not only were there dire consequences such asthese, similar interactions occurred with backcontamination.Instrumental in voicing these alarming concerns were ahandful of scientists – pioneers – who were keyvisionaries of the importance of practicing what we nowidentify as planetary protection. Stanford geneticist andNobel Laureate Joshua Lederberg, University ofCalifornia at Berkeley Chemist and Nobel LaureateMelvin Calvin, and British biologist J. B. S. Haldane wereall essential in identifying the ease by which spaceexploration, could in theory, contaminate theenvironments which were being explored. At the time,there was the fear that living organisms from Earth couldgrow and spread in a new environment, thereby affectingthe characteristics and ecologies of any existing life formswhich might be natural habitants of the satellite underquestion. Lederberg’s concerns and warnings to thescientific community at large were eventually recognizedby the U.S. National Academy of Sciences (NAS), whichtook the first action of adopting resolutions paving theway to international and national treaties and agreements[2].Planetary protection is NASA’s policy of addressing theconsequences, which might occur when material isactually transferred between different planets – if life doesexist on other worlds. Not only does planetary protectionwant to protect solar system bodies (planets, moons,asteroids, and comets) from Earth life, it also intends toprotect the Earth from extraterrestrial life, which, if itexists, could be brought back from other solar systembodies.Here, we wish to describe the historicaldevelopment of the practice and philosophy of planetaryprotection as it relates to Mars missions.2. BEGINNINGS OF PLANETARY PROTECTION3. INTERNATIONAL TREATIES ANDAGREEMENTSIn the 1950’s, a new frontier was added to those of landand sea exploration: space. The burning “need to know”was (and is) real, and was instrumental in the launch ofthe Space Age to determine if we are alone in theuniverse, i.e., the existence of life on other planets.In 1958, the NAS called for the International Council ofScientific Unions (ICSU) to develop a process wherespacecraft would not jeopardize or compromise theintegrity of any scientific investigation [3]. As a result,ICSU established an ad hoc Committee on Contaminationby Extraterrestrial Exploration (CETEX) primarily toprovide findings on vulnerabilities to contamination.CETEX recommended precautions be taken against spacevehicles landing on Mars or Venus (accidentally ordeliberately) without first sterilizing them. CETEXreasoned that if precautions were not taken, then futureexplorations would have devastating effects on any typeof life form – if they existed. Consequently, it wasapparent that a code needed to be drafted to protect futureinterplanetary exploration as well as the protection ofextraterrestrial environments. The ICSU gatheredinternational experts to address these issues [4], leading tothe establishment of the Committee on Space Research,COSPAR [5].Although life exists in different extreme environments onEarth, there is no data whatsoever on alternativechemistries that may support life – not as we know life tobe, but of an entirely exotic, unrelated form – elsewherein the Universe.To determine these alternativechemistries, extraterrestrial environments – and any livingorganisms that exist within them – must be protected untilthe space community has the opportunity and technologyto identify them. Today’s planetary protection policy isdominated by the principle of safeguarding scientificinvestigations and instrumentation “It’s strictly toprotect science. Planets for the sake of science [1].”As early as 1956, there were rumblings of concernregarding possible lunar and planetary contamination asthe race for space exploration was just beginning. Thisled to an attempt to coordinate international efforts so thatsuch things did not happen – particularly since there weretwo launches that surprised most Americans andinstigated the U.S. Space Program: the successfullaunches of Sputnik I and of Sputnik II towards the end ofDuring this same time, Lederberg’s efforts contributedthe establishment of the Space Science Board (SSB)June of 1958 [6]. Not only was the NAS instrumentalestablishing the SSB, it also directed the SSB to work2toininin
collaboration with civilian and Government agencies, inparticular, the newly established National Aeronauticsand Space Administration (NASA), the National ScienceFoundation (NSF), the Advanced Research ProjectsAgency (ARPA), and foreign partners in this field. Theestablishment of NASA was a direct outcome of therecent political unease, which had arisen from the launchof Sputnik in 1957. Within just a few months, COSPARwas in the middle of encouraging joint internationalcollaboration and information exchange in interplanetaryendeavors. COSPAR had to deal with fundamental spaceresearch, and explore and understand biological aspects rilization and planetary quarantine. COSPAR was toserve as an arena for open dialog between space scientistsfrom the Eastern bloc and those from the rest of the world– mostly from the United States. During the Cold War,COSPAR selected vice-presidents from the USSR andfrom the US, assuring both countries of equalrepresentation. COSPAR actively enforced that both ofthese countries examine approaches, which would avoidtransfer of terrestrial organisms to other planets. This wasthe first step in implementing a program to protect planetsof biological interest so that life detection experimentswould not be disqualified by contamination, leading toirreversible and permanent changes. In essence allspacefaring nations had to share details for spacecraftengineering and fabrication. Planetary protection couldonly be achieved with full cooperation of all countriesparticipating in space exploration [7].Abe Silverstein’s efforts, the first sterilization policies andapproaches were officially implemented in November1959 [9].In the early 1960’s, a special Life Science Program wasestablished within NASA, which included spacecraftsterilization and exobiology programs within its mandate.The Jet Propulsion Laboratory (JPL) led the effort forconstructing a facility to identify fundamental issues indecontaminating and sterilizing space probes [10]. JPL’srole in the implementation of planetary protectionpractices began at that time, and was focused on twomajor issues: to determine which spacecraft componentswere compatible to sterilization treatments, and todevelop new spacecraft components or materials whichwere more compatible to sterilization treatments, becausetesting had proven that not all components werecompatible. Adding to this level of complexity was thematter of mission categorization, which was defined inessence, by the science the mission would carry out.4. MISSION IMPLEMENTATIONMission planetary protection requirements are defined bythe NASA Procedural Requirements (NPR) 8010.12, PPCategorization Letter from the NASA PlanetaryProtection Officer (PPO), and the NASA Level 1requirements.The requirements from NPR 8020.12 detail the generalmission requirements for target body and mission type,monitoring and verification requirements, gate umentation and review requirements, microbialreduction requirements, launch and post launchoperations, and roles and responsibilities. Processrequirements are also detailed in NPR 8020.12 in theAppendices to include communications with the PPO foreach mission phase and process specification sheets forbioburden assumptions and microbial reduction processes.Similar to COSPAR, the United Nations GeneralAssembly established another ad hoc committee inreaction to the launch of Sputnik and for the prevention ofcontamination of celestial environments. In 1959, theCommittee on the Peaceful Uses of Outer Space(UNCOUPUOS) was asked to identify and report on legalissues arising from human exploration of space, includingforward and back contamination dangers. This led to therecommendation of the development of internationalagreements and guidelines – similar to COSPAR. Due tothese efforts, President Lyndon Johnson proposed thebasis of such a treaty in July 1966. The Outer SpaceTreaty was issued in January 1967. This internationalagreement provided articles supporting space exploration,but specifically claimed that all exploration should beconducted to preserve the environments being explored,particularly in Article IX [8].As part of the NASA PP process, the mission is requiredto submit a request for a PP categorization. This request isto include a matured mission plan, description of theprimary mission, science objectives, and payloadinvestigations to include particular detection sensitivitiesof biochemical molecules. From a PP stance, the desiredlanding site capabilities should also be discussed toinclude (or exclude) a Mars Special Region (SR) asdefined by the Mars Exploration Program Analysis Group(MEPAG-SR) Report. The categorization should includethe projects’ best knowledge of the project’s likely PPcategorization and build upon that assertion. Detailedanalysis may be required for the mission specificengineering or science needs (e.g. Radioisotope PowerSystem (RPS) assessment for creation of a spacecraftinduced special region in an off-nominal landing orcontamination / recontamination analysis). The PP Officer(PPO) will then evaluate the mission categorizationrequest letter and issue a mission categorization letterJoshua Lederberg and Carl Sagan’s lobbying efforts werevery effective in identifying methods to prevent forwardand back contamination. Consequently, the first anticontamination panel was formed in 1963, underCOSPAR’s jurisdiction. Lederberg’s stature for being aNobel Laureate helped make a strong case forimplementing rigorous planetary protection measures.NASA’s first spacecraft sterilization policy statements areall attributed to Lederberg’s concerns and lobbying.Along with NASA’s Director of Space Flight Programs,3
detailing the mission categorization formally assigned,reiterate the relevant NPR 8020.12 requirements, andnotify the project of any mission specific requirements.regions”) were detected by orbiters, and the evidenceindicated that none could exist.An alternative approach was developed in the 1980s [13],[14]. The previous method employed the Coleman-Saganformula in a form apparently never formally published,which allowed an estimation of the probability ofcontamination Pc of Mars versus a requirement (10-4 forViking):NASA HQ can directly impose Level 1 requirementsspecifically related to the mission in the Program LevelRequirements Appendix. These requirements are brokeredbetween the Project and NASA Program Managementand are a direct path into the Project’s SystemEngineering requirements.Pc N0RPsPIPRPgThus, PP requirements come to a mission in somecombination of the NPR 8020.12, PP categorization fromthe PPO, and the NASA Level 1 requirements. In general,the mission type defines the PP implementation approachfor Mars-based missions. These requirements then flowinto the Project System Engineering requirements systemand the PP approach is defined in the Project PP Plan. Ifrelevant, the detailed implementation approaches to theprobability of impact analyses, bioburden assessment,unique project analysis, and the closed loop verificationprocess is described in the PP Implementation Plan andsubsidiary Plans (if relevant).(1)Where N0 the number of microorganisms on thespacecraft initially, R reduction due to conditions on thespacecraft before and after launch (include Dry HeatMicrobial Reduction, DHMR), PS Probability themicroorganisms on the spacecraft reach the surface of theplanet, PI Probability that the spacecraft will impact theplanet (one for a lander), PR Probability ofmicroorganisms being released in the environment afterlanding (usually set to one for all bioburden for crashlanding, specification values for surface, mated, andencapsulated otherwise), and Pg Probability of growth(for targets with liquid water typically set to one).5. MISSION COMPLEXITY: PPIMPLEMENTATION PLANS AND CHALLENGESThis calculation was performed for each of the exposed,mated and encapsulated components of the total burden,which had unique values for the probability of release.An Introduction to PP Implementation – NASA RoboticMars Missions at JPLThe new requirements for Mars were placed on theburden N0 itself (no calculations required). The logic wasto invert the formula into a formula for the allowed N0.More importantly, the requirements for landers without insitu life detection experiments were set to the burdenvalues for the Viking landers prior to terminal heatsterilization. For landers with life detection experiments,the requirements were set to the best estimate for theViking landers post the terminal sterilization (not zero).This general approach was accepted and recommended bythe SSB in 1992 [15]. Further work [16] provided thespecification values for the allowed (maximum) burden,3x105 spores on exposed and mated surfaces, and 5x105spores total (all surfaces, mated and encapsulated). Thetwo values reflect the thought behind the probability ofrelease. These values were adopted for use by MPFthrough its PP documentation, which refers to a final draftform of the new NASA Planetary ProtectionRequirements document [17], [18]. Also, the averagebioburden on the exposed surfaces of the landed systemwas not to exceed 300 spores/m2.Planetary protection implementation requirements havecontinued to evolve from one mission to the next asknowledge of the red planet and other celestial bodies ofbiological interest continues to expand based on what islearned in preceding projects. In general, PP requirementsbecome more restrictive as more is learned about life inextreme environments and new ways to detect it.Mars Pathfinder (MPF)—In the 22 years between thelaunch of Viking in 1975 and the launch of MarsPathfinder late in 1996, there were no NASA landermissions. Hence, when the development of MPF began,the formal NASA planetary protection requirements forMars were as they were for Viking [11]. There was,however significant activity to modify these requirementsin the intervening period. The findings of Viking biologyexperiments and the measurements by other instrumentssuggested that spacecraft sterility was an excessivelyrigorous requirement. In 1978, the Space Science Board(SSB) of the National Research Council1 issued a sharplyreduced (less stringent) set of values for the keyparameter in the analytical approach to the estimation ofthe probability of contaminating Mars, the probability ofgrowth Pg [12]. The SSB was particularly reluctant to set avalue for Pg, especially for the surface at subpolarlatitudes, because terrestrial organisms could not growthere except in oases. No oases (early version of “special1MPF satisfied the burden requirements by selected dryheat microbial reduction (DHMR) with the use of theexisting specifications. There was no system levelsterilization.The central electronics module wasexempted for the burden requirement by isolation behinda high-efficiency particulate arresting (HEPA) filter, aunique approach first done for this project. The sporecount was estimated per the NASA standard assay [19] asNow the Space Studies Board (SSB)4
used for Viking, except only heat-shocked samples wereassayed, to represent spores. The requirements weresilent on statistics; MPF used estimated standarddeviations and 3-sigma upper limit in counts appliedagainst burden requirement. This conservative measurewas intended to account for the sampling. A majordeparture from the burden requirements applying to thevalues at launch was approved through the MPF PP plandocument. The external surfaces of the aeroshell and theentirety of the cruise stage were shown sterilized byatmospheric heating during entry at Mars.Thisprecedent was employed in later missions as well. Moredetails on the microbiological cleanliness of MPF areavailable in the public domain [20].each component and to feed the rolled up estimates at thesubsystem level. The PPEL contained surface areas,volumes, materials, and cleaning/microbial reductionprocesses, organized based on the project’s massequipment list to facilitate updates as the flight systemmatured. This methodical approach was necessitated bythe vastly increased complexity and size of the MERspacecraft, compared to MPF. The MER PP teamdeveloped another new tool to support bioburdenestimation: the Barcode system. The Barcode system wasan Access database containing all data relative to bioassaysampling from descriptions and pictures of each sampletaken through the 24, 48, and 72-hr plate counts for eachsample.Following the developments in the 1980s and early 1990s,changes were made to other requirements, such as forprobability of impact PI of Mars (that also apply toorbiters and flybys) [17], [18]. A new structure to therequirements for all solar system bodies was alsoestablished (but beyond the subject of this paper). Thespecification values for the launch vehicle and thespacecraft PI were made less stringent during the MPF PPactivities. The value for the launch vehicle (or any part ofit) was 10-5 at time the PP plan was written, but became10-4 for PP prelaunch report. The PI (for a hard impact)by the lander was 10-3.Note that the total maximum number of spore formingorganisms, and the population density per square meterrequirements were unchanged from MPF. In addition,there were two complete spacecraft to account for. Thespreadsheet was based on the analogous Mass EquipmentList (MEL), used to track and estimate the mass of allcomponents and the system mass. In addition, MERgreatly extended the use of HEPA filter isolation firstemployed with MPF, for many electronic modules.The MPF Project did not consider PP issues/requirementsuntil the critical design phase of spacecraft development.The MER Project chose to actively include PP in designconsiderations beginning in the Pre-Project phase andcontinuing through launch. The PP requirements for MERwere the same as for MPF with one exception: theprobability of impact by the flight system requirementwas 10 2 rather than 10 3. The probability of impactrequirements were met using the same methodology as forMPF.The method for the estimation of PI for a spacecraftduring the interplanetary cruise phase was improved fromthe previous practice by the introduction of systemreliability and meteoroid kill parameters. The formula forPI𝑃! !!!! 𝑝!𝑞!!!(2)The MER Project had the added complexity of twoessentially identical flight systems, each consisting ofapproximately 4.5 x 103 m2 of accountable surface area.The biological cleanliness requirements were met using acombination of dry heat microbial reduction (DHMR),alcohol-wipe cleaning, bioassay sampling, and HEPAfilter isolation. DHMR was used on components withlarge surface area (e.g., airbags, parachute, multi-layerinsulation), and those with surfaces that were difficult toclean or not cleanable (e.g., open-cell foam, motor interiorsurfaces, aluminum honeycomb). HEPA filter isolationwas used on the main rover chassis and smallerelectronics modules on the rover and lander. Alcoholwipe cleaning was used on easy-to-clean surfaces prior tointegration and to maintain cleanliness during integrationand after system-level testing. Bioassay sampling wasperformed periodically to assess cleanliness protocols,and at last available access during integration. Thebioassay sampling goal was to sample, at last availableaccess, 10% of those surfaces accountable by bioassay.The MER PP team chose to include the results of theNASA Planetary Protection Officer’s (PPO) verificationsamples in the final flight system bioburden estimate.where pi is the probability that the ith maneuver leaves thespacecraft in an impact trajectory and qi 1 is theprobability that the next maneuver (after the ith maneuver)would fail. Prior to MPF, the value of all qi 1 were takento be 0.01, except for i n, the last maneuver, for whichthe value was one, of course. Of course, the probabilityof a system failure or a meteoroid impact depends on theduration between maneuvers. Values for these twoparameters were obtained by a reliability analysis of MarsGlobal Surveyor (a Mars orbiter) and a study of onespacecraft and some historical data [11]. This analysis setthe precedent for later projects. The values were neveradopted as NASA specifications, but their use wasexplicitly permitted by way of project PP plans. Ofcourse, project specific analyses are preferred, but costly.Mars Exploration Rover (MER)—The principaladvancement in the PP implementation of the MarsExploration Rover mission (MER) was the developmentof a spreadsheet of all of the parts of each MERspacecraft system, the PP Equipment List (PPEL). ThisEXCEL workbook provided a method for tracking the PPstatus and estimated or measured value of the spores on5
rigorous constraints (i.e., like MER), a deployablebiobarrier was designed to isolate the robotic arm. Thebiobarrier was deployed after landing. This was the firstinstance of a split system requirement and a possibleprecursor to future Mars sampling projects.The MER PP team developed two new tools to supportbioburden estimation: the Planetary Protection EquipmentList (PPEL) and the Barcode system. The PPEL was anExcel workbook containing surface areas, volumes,materials, and cleaning/microbial reduction processes;organized based on the project’s mass equipment list tofacilitate updates as the flight system design matured. TheBarcode system was an Access database containing alldata relative to bioassay sampling from descriptions andpictures of each sample taken through the 24, 48, and 72hr plate counts for each sample.Mars Science Laboratory (MSL)—The Mars ScienceLaboratory mission (MSL) had the same spore constraintsas MER did, while the entire flight system and the rovergrew even larger in size and complexity than MER.Considering evolving requirements from the joint work ofNASA and ESA, getting a fully functioning flight systemto meet PP requirements became more difficult than ever.The MER Project took credit for entry heating of thecruise stage and heat shield as was done by MER andMars Polar Lander (MPL); however, entry heating of thebackshell was not expected to raise the backshell (BS)external surfaces to sterilization temperatures. The MERPP team then had to consider all backshellrecontamination risks and the bioburden encapsulated inthe backshell thermal protection system (TPS). Backshellrecontamination risks consisted of cruise stage (CS)surfaces, the heat shield (HS) outboard surface, launchvehicle (LV) fairing, payload attachment fitting (PAF),upper stage, and fairing air conditioning. To limitrecontamination of the backshell, the PP team imposed acleanliness requirement of a maximum surface sporedensity of 1000 spores/m2 on all of these surfaces,necessitating cleaning and bioassay sampling of thesesurfaces. The fairing air conditioning duct was alsosampled, and HEPA filters were required in the airconditioning flow. Bioburden in the backshell TPS wasreduced by DHMR of the backshell and the TPS.PP requirements for a mission formally begin with arequest for categorization from the Planetary ProtectionOfficer, the PPO. For MSL there were additionalrequirements: landing sites had to be addressed as well asthe ability to access special regions on Mars. MSL wasdesignated a Category IVb mission. After the landing sitewas selected and changes to some of the hardware, it wasultimately launched as a Category IVa mission.Biological cleaning or microbial reduction of thehardware was accomplished through the use of severaltechniques including mechanical cleaning using precisioncleaning, alcohol wiping, as well as dry heat microbialreduction. All flight hardware was cleaned and bioassayedprior to entering the Assembly, Test and LaunchOperations (ATLO) flow. For MSL, almost 90% of allsurfaces saw standard or non-standard Dry HeatMicrobial Reduction (DHMR). During ATLO ashardware inaccessibility was achieved, bioburden levelswere assessed by sampling surfaces, and if values wereabove limits, hardware was subjected to re-cleaning andre-sampling until accept
throughout the course of NASA sponsored robotic Mars lander or rover missions, starting from Mars Pathfinder through the beginning of Mars 2020. Highlighted in the discussion will be process changes in planetary protection requirements development and flow down. Develo