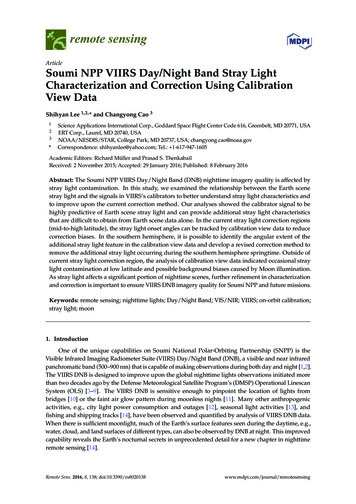
Transcription
remote sensingArticleSoumi NPP VIIRS Day/Night Band Stray LightCharacterization and Correction Using CalibrationView DataShihyan Lee 1,2, * and Changyong Cao 3123*Science Applications International Corp., Goddard Space Flight Center Code 616, Greenbelt, MD 20771, USAERT Corp., Laurel, MD 20740, USANOAA/NESDIS/STAR, College Park, MD 20737, USA; changyong.cao@noaa.govCorrespondence: shihyanlee@yahoo.com; Tel.: 1-617-947-1605Academic Editors: Richard Müller and Prasad S. ThenkabailReceived: 2 November 2015; Accepted: 29 January 2016; Published: 8 February 2016Abstract: The Soumi NPP VIIRS Day/Night Band (DNB) nighttime imagery quality is affected bystray light contamination. In this study, we examined the relationship between the Earth scenestray light and the signals in VIIRS’s calibrators to better understand stray light characteristics andto improve upon the current correction method. Our analyses showed the calibrator signal to behighly predictive of Earth scene stray light and can provide additional stray light characteristicsthat are difficult to obtain from Earth scene data alone. In the current stray light correction regions(mid-to-high latitude), the stray light onset angles can be tracked by calibration view data to reducecorrection biases. In the southern hemisphere, it is possible to identify the angular extent of theadditional stray light feature in the calibration view data and develop a revised correction method toremove the additional stray light occurring during the southern hemisphere springtime. Outside ofcurrent stray light correction region, the analysis of calibration view data indicated occasional straylight contamination at low latitude and possible background biases caused by Moon illumination.As stray light affects a significant portion of nighttime scenes, further refinement in characterizationand correction is important to ensure VIIRS DNB imagery quality for Soumi NPP and future missions.Keywords: remote sensing; nighttime lights; Day/Night Band; VIS/NIR; VIIRS; on-orbit calibration;stray light; moon1. IntroductionOne of the unique capabilities on Soumi National Polar-Orbiting Partnership (SNPP) is theVisible Infrared Imaging Radiometer Suite (VIIRS) Day/Night Band (DNB), a visible and near infraredpanchromatic band (500–900 nm) that is capable of making observations during both day and night [1,2].The VIIRS DNB is designed to improve upon the global nighttime lights observations initiated morethan two decades ago by the Defense Meteorological Satellite Program’s (DMSP) Operational LinescanSystem (OLS) [3–9]. The VIIRS DNB is sensitive enough to pinpoint the location of lights frombridges [10] or the faint air glow pattern during moonless nights [11]. Many other anthropogenicactivities, e.g., city light power consumption and outages [12], seasonal light activities [13], andfishing and shipping tracks [14], have been observed and quantified by analysis of VIIRS DNB data.When there is sufficient moonlight, much of the Earth’s surface features seen during the daytime, e.g.,water, cloud, and land surfaces of different types, can also be observed by DNB at night. This improvedcapability reveals the Earth’s nocturnal secrets in unprecedented detail for a new chapter in nighttimeremote sensing [14].Remote Sens. 2016, 8, 138; nsing
Remote Sens. 2016, 8, 1382 of 15The superior DNB nighttime data quality in SNPP is partly due to the fact that itson-orbit performance exceeds its original design specification [15]. On-orbit DNB calibration andcharacterization is a challenging undertaking because most useful observations have radiance valuesthat are below the sensor’s designed minimum observable radiance. Stray light contamination causesthe most significant degradation in DNB nighttime image quality. The most persistent stray lightcontamination is in the mid-to-high latitude regions where the spacecraft is crossing the northern andsouthern day/night terminators [16]. The exact latitudes affected by stray light can be determined bythe Earth-Sun-spacecraft geometry and orbital inclinations, which change over time. In these regions,the spacecraft is under direct solar illumination due to its elevated orbital track at 830 km relative tothe nighttime Earth surface. Due to the large difference between the Sun and nighttime Earth viewradiances, the tiny fraction of Sunlight entering the optical system causes significant contamination ofthe images [17]. Without correction, large swaths of the nighttime scenes will have little use becausethe stray light dominates most nighttime imagery signals.The current VIIRS operational calibration algorithm includes stray light correction to removethe mid-to-high latitude stray light contamination that occurs during the spacecraft’s northern andsouthern day/night terminator crossings. The stray light is estimated based on Earth view datameasured over dark surfaces during moonless nights, with an assumption that stray light and airgloware the only contributors to the observed signals [16,17]. Stray light intensity is estimated as a functionof the satellite’s zenith angle to account for the effects of Earth-Sun-spacecraft geometry. The estimatedstray light is also detector- and scan angle-dependent, which indicates that there is a scattered lightpath that is sensitive to the positioning of the rotating telescope assembly (RTA) as well as to the minordifferences in detector locations on the focal plane. Operationally, stray light is estimated once permonth during the new moon to best approximate the stray light optical path. The monthly estimatedstray light magnitudes are stored in a look-up table (LUT). The online calibration algorithm appliesthe correction derived from the LUT with the closest Earth-Sun-spacecraft geometries relative to thescene to minimize biases.The accuracy of the stray light estimation depends on how well the dark surfaces are selected,and airglow approximated. Since a completely dark surface over the entire stray light-affected regionis unobtainable, the signals over the dark surfaces at each detector and scan angle are estimated usingmany orbits of data, excluding data with potential light sources [16,17]. Since the dark surface selectionprocess is imperfect, small uncertainties could occur due to residual data contamination. With theselected dark surfaces, the dark signals can be computed, taking into account the combined effect ofstray light and airglow. To estimate the stray light, airglow is estimated from dark surfaces outside ofthe stray light affected region as an approximation. This airglow approximation is apt to be biasedbecause airglow is not spatially and temporally uniform. Since airglow intensity is usually muchsmaller than the stray light, the error in stray light estimates is expected to be small. However, theuncertainty in airglow approximation indicates that current stray light-corrected images likely do nothave accurate airglow patterns and magnitudes.Additional uncertainty in stray light correction is due to the slight shift in the Earth-Sun-spacecraftgeometry between the time stray light is estimated and the imaging time. The stray light correctionbiases due to the mismatch in Earth-Sun-spacecraft geometry are small in areas where stray lightchange, with respect to the satellite’s zenith angle, is gradual. However, in regions with sharp straylight changes, i.e., the penumbra and the solar diffuser onset angle, large biases could occur. The largebiases result in a stray light-corrected image with an observable horizontal strip due to significantunder/over correction.In addition, the current operational method cannot resolve an additional stray light feature thatoccurs in the southern hemisphere during its springtime (October–December) [18]. The additionalstray light could affect up to one-fourth of the total stray light region. The additional stray light usuallyexists near the onset of the twilight region, an area where stray light is most difficult to estimate [16,17].The additional stray light cannot be predicted by current methods because it has features considerably
Remote Sens. 2016, 8, 1383 of 15different than the overall stray light pattern. Modification of the current methods is needed in order toremove the additional stray light features in the southern hemisphere.Previous studies have suggested using calibration view data to improve stray lightcharacterization and correction [16,17,19,20]. In this study, we performed detail analyses on DNBsignal characteristics in the VIIRS on-board calibrators, and their corresponding Earth view stray lightRemote Sens. 2016, 8, 1383 of 14characteristics.We show the potential of using the DNB calibration view signals to improvestraylight tcorrection.Inthesouthernhemisphere,show the potential of using the DNB calibration view signals to improve stray light onset anglethe regionsstray lightcan beInidentifiedin hemisphere,the calibratorsignals,withand thetrackingwithand toadditionalrefine the currentstray featureslight correction.the southernthe regionscurrentcorrectioncan canbe modifiedto removethese features.wecurrentwill showthe use ofadditionalstrayalgorithmlight featuresbe identifiedin the calibratorsignals, Last,and thecorrectionalgorithmcandatabe modifiedto removeLast, we will showthe ofusetheof currentcalibrationviewcalibrationviewto identifypossiblethesestrayfeatures.light o-highlatitudestraylatitude stray light regions, as well as other potential background contaminations.light regions, as well as other potential background contaminations.2. DNB Calibration Framework2. DNB Calibration FrameworkThe SNPP VIIRS is a Sun-synchronized, polar-orbiting, scanning radiometer [21]. On orbit, VIIRSThe SNPP VIIRS is a Sun-synchronized, polar-orbiting, scanning radiometer [21]. On orbit, VIIRScollects data in four separate view windows, Earth view (EV), blackbody (BB), solar diffuser (SV), andcollects data in four separate view windows, Earth view (EV), blackbody (BB), solar diffuser (SV), andspace view (SV), successively at each scan (Figure 1). The BB, SD, and SV are on-board calibratorsspace view (SV), successively at each scan (Figure 1). The BB, SD, and SV are on-board calibrators(OBCs)designedto thermalemissivebands,(OBCs)designedto calibrateVIIRS’sreflectivereflective solarsolar bandsμm)andthermalemissivebands,and y[22–24].TheVIIRSBBhasand are also used as an alternative DNB calibration methodology [22–24]. The VIIRS BB has high highemissivityandandis temperature-controlled vityis temperature-controlled atat 292.5thethethermalemissivebands.bands.The SDTheis SD is a nearLambertianpanel rbandsduringorbitala nearLambertianSpectralonSpectralon panelreflectivesolarbandsduringorbitalsolar here day/nightterminatorcrossings.The SVis SVlocatedat natorcrossings.Theis art ofof EVthreedegreesoff offthe theedgeedgeof thea preselectedscanangleEV ��sthe Earth’swhichis usedto providea adeepdeepspacespace viewreferences.limb,limb,whichis usedto provideviewforfordarkdarkreferences.Figure1. Schematicof es.Figure1. Schematicof VIIRSdatacollectioncollectionwindowswindows andangles.The Thescan scanangleangleat theatEarth’slimblimbis 62.5degrees.the Earth’sis 62.5degrees.The DNB consists of four sectors of detector arrays: one is in the low-gain stage (LGS); one is inThe DNBconsists of four sectors of detector arrays: one is in the low-gain stage (LGS); one is inthe intermediate-gain stage (MGS); and two redundant arrays, high-gain A (HGA) and high-gain Bthe intermediate-gain stage (MGS); and two redundant arrays, high-gain A (HGA) and high-gain B(HGB), are in the high-gain stage (HGS). In each scan, the DNB collects samples using the four detector(HGB),are in the high-gain stage (HGS). In each scan, the DNB collects samples using the four detectorarrays and aggregates them into 4064 EV samples and 16 calibration view samples. The output gainarraysandthem4064samplesand 16calibrationviewsamples.outputstage isaggregatesselected at eachEVintosampleto EVensureits radiancelevelis within thedynamicrangeTheof thatgain gainstagestageis selectedat eachEV sampleto ensureits radiancelevelwithin averagedthe dynamicrangeof thatto reducedown-linkbandwidth.The HGAand HGBare isnormallyon-boardandgain stageto reducedown-linkbandwidth.TheHGAandarraysHGB arearereportednormallyaverageddown-linkedas HGS.In calibrationviews, allfourdetectorin eachpixel. on-board andFigureshowsDNB signalindetectoreach of thecalibrationviews forentireorbit.down-linkedas 2HGS.Intypicalcalibrationviews,profilesall fourarraysare reportedin gure 2 shows typical DNB signal profiles in each of the calibration views for the entire orbit.relationshipbetweensatellite,Sun,and Earth.Duringdaytime,the SDanglessignalstoareseveral theordersofIn Figure2, the tegeometricmagnitude higher than the SV and BB signals. The stronger SD signals are due to high reflectivityrelationshipbetween satellite, Sun, and Earth. During daytime, the SD signals are several orders ofsurfaces that are illuminated by the daytime Earthshine coming through VIIRS EV port. The BB’s lowmagnitude higher than the SV and BB signals. The stronger SD signals are due to high reflectivityreflectivity absorbs most of the incoming lights, and the recorded BB signals are likely dominated bysurfacesthat areilluminateddaytimecomingthroughEV port.scatteredlightsentering intobythetheopticalpaths.EarthshineDuring daytime,the SVsignals VIIRSare slightlyhigherThethanBB’sBB lowreflectivityabsorbsmostof theincominglights,the recordedBBthesignalsareterminators.likely dominatedin generalbut sharesimilarpatterns,exceptwhenandthe spacecraftis nearday/nightSince bythe SV is about three degrees off the Earth’s limb, the Earth scene stray light coming through thetelescope could contribute additional signals added onto the stray light. The position of the telescopecould also change the scattered light patterns; an effect that can be observed in EV, where the stray light
Remote Sens. 2016, 8, 1384 of 15scattered lights entering into the optical paths. During daytime, the SV signals are slightly higher thanBB in general but share similar patterns, except when the spacecraft is near the day/night terminators.Since the SV is about three degrees off the Earth’s limb, the Earth scene stray light coming through thetelescope could contribute additional signals added onto the stray light. The position of the telescopeRemote Sens. 2016, 8, 1384 of 14could also change the scattered light patterns; an effect that can be observed in EV, where the straylight ). Examiningthe calibrationviewsignal characteristicsangles(see(seeSection3). Examiningthe calibrationview ionalinformationon potentialstraylightpathseach hascalibratorits ownadditionalinformationon potentialstray lightpathssinceeachsincecalibratorits own hasuniqueproperties.uniqueproperties.Figure 2. DNB calibration view signals for S-NPP orbit 15,758 on 12 November 2014. DNB detector 1Figure2. DNB calibration view signals for S-NPP orbit 15,758 on 12 November 2014. DNB detectorsignals for SV, BB, and SD are shown in (a–c), respectively. The y-axis are DNB signals in W·cm 2·sr 1. The1 signals for SV, BB, and SD are shown in (a–c), respectively. The y-axis are DNB signals inx-axis is the VIIRS Sun declination angle, in degrees. NH: northern hemisphere, SH: southern hemisphere.W cm 2 sr 1 . The x-axis is the VIIRS Sun declination angle, in degrees. NH: northern hemisphere,SH: southernThe DNBhemisphere.images have the most significant stray light problem (highlighted in Figure 2) near theday/night terminators. Although the stray light is stronger during the day than the day/nightTheDNB imageshavethe mostsignificantstrayproblem(highlightedFigure2) nearterminators,the effectis negligiblebecausethe straylightlightis severalordersof magnitudeinsmallerthanthe typical terminators.daytime radiance.In the thestraylightlightproblemregions,duringthe calibrationarethe day/nightAlthoughstrayis strongerthe day viewthan signalsthe day/nightelevated thewitheffectpatternsthat are differentthe lightnorthernand southernIn theterminators,is negligiblebecausebetweenthe strayis severalorders hemisphere.of an the typical daytime radiance. In the stray light problem regions, the calibration view signalsillumination. There is an additional scattered light through BB at the end of the DNB EV stray lightare elevated with patterns that are different between the northern and southern hemisphere. In theproblem region that caused sharp increase in BB signal. The timing of the sharp increase in BB signalsnorthern hemisphere, the bulk of the signals likely came through the EV port from the direct solarcorresponds to the onset of twilight on the Earth’s surface. However, there is no correspondingillumination.is an additionalscatteredEarthlightscenethroughBB atintheof theDNBTheEVlackstrayincrease inThereSV signalsdue to the increasedradiancethe endtwilightregion.of l.ThetimingofthesharpincreaseinBBsignalsresponse in SV signals indicates that an RTA pointing angle might have provided a better shield fromcorrespondsto theonsetof twilighton the Earth’sthe scatteredlightpathsfrom the Earth’stwilight surface.scene. However, there is no corresponding increaseIn thesouthernthe onsetof t inin SV signalsdueto thehemisphere,increased Earthsceneradiance inthesignaltwilightregion.The lacktoofsolar illuminationenteringVIIRS’s angleEV port.Thehavesharpincrease ainbetterthe SDsignalmarkedtheSV signalsindicates thatan RTAthepointingmightprovidedshieldfromthe beingilluminatedbytheSun.BothBBandSVlight paths from the Earth’s twilight s,duetoadditionallightsenteringfromSDscreen.In the southern hemisphere, the onset of calibration view signal increase corresponds to theThe BB signals showed a rise and fall that largely corresponded to the SD signals, although severaldirect solar illumination entering the VIIRS’s EV port. The sharp increase in the SD signal markedorders of magnitude smaller than SD. The SV signals showed a sharp increase at the onset of SDthe beginning of solar calibration events when SD is being illuminated by the Sun. Both BB andcalibration events but remained more or less constant throughout the solar calibration event. TheSV showedbut levelsin differentpatterns,due toadditionallightsfrom SDrecordedincreasedcalibrationsignals,view signalduring solarcalibrationcouldindicate thelevelenteringof stray n. Figure 3 shows that BB and SV have signals that are at least four orders of n.SD. The SVsignals showeda sharpincreaseat thesmallerthanSD signalssmallerduring solarUnfortunately,we cannotdirectlymeasurehowonsetmuch of the SD signals are stray light, which will cause bias in calibration. The signal ratios of SV/SDand BB/SD imply the potential stray light contamination during solar calibration of less than 0.01%.
Remote Sens. 2016, 8, 1385 of 15of SD calibration events but remained more or less constant throughout the solar calibration event.The recorded calibration view signal levels during solar calibration could indicate the level of straylight contamination. Figure 3 shows that BB and SV have signals that are at least four orders ofmagnitude smaller than the SD signals during solar calibration. Unfortunately, we cannot directlymeasure how much of the SD signals are stray light, which will cause bias in calibration. The signalSens. 2016,1385 of 14ratios ofRemoteSV/SDand8,BB/SDimply the potential stray light contamination during solar calibrationofless lightdependency,theresultsindicatethatthethere is a large wavelength stray light dependency, the results indicate that the reflective solarreflectivesolarbands calibrationerrorlightduecontaminationto stray lightcontaminationbandscalibrationerror due to strayshouldbe negligible.should be negligible.Figure3. ratiosMean ratiosof ation events25,25, 2014.Figure 3.Meanof BB/SDand andSV/SDsignalsaroundeventsononAugustAugust2014. The dashed lines indicate the approximated solar calibration angle range. SZA solar zenithThe dashed lines indicate the approximated solar calibration angle range. SZA solar zenith angle.angle.3. Stray3. LightCharacterizationandStray minentmost prominentstray contaminationlight contaminationon DNBimageryoccursmid-to-highlatitudeThe moststray lighton DNBimageryoccursin inthethemid-to-highlatitude of the northern and southern hemispheres when spacecraft is crossing the day/nightof the northern and southern hemispheres when spacecraft is crossing the day/night terminators [16].terminators [16]. The stray light magnitude and its affected latitudes depend on theThe straylight magnitude and its affected latitudes depend on the Earth-Sun-spacecraft geometricEarth-Sun-spacecraft geometric relationships, and the patterns usually reoccur at a yearly cycle whenrelationships,andEarth-Sun-spacecraftthe patterns usuallyreoccurat a yearlycycletheEarth-Sun-spacecraftthe esimilarEarth scenestray light,geometricrelationshipsrepeat.Toonremovethe Earthscenestraylight,twothedifferentstray lightis areestimatedthe straylight is estimatedbasedthe observedEV n,weexaminethebased on the observed EV dark signals. Currently two different methods are implemented instray light characteristicsbased on therelationshipof observedstray lightcalibrationthe publicly-availableDNB imageryproducts[16,17].In thisEVsection,weandexaminetheviewstray lightsignals to explore ways to improve current stray light corrections. We will demonstrate that thecharacteristics based on the relationship of observed EV stray light and calibration view signals toinformation in calibration view data can be used to modify current stray light correction method toexploreremoveways tolightWe willhemispheredemonstratethat the informationtheimproveadditional currentstray lightstrayfeaturesthatcorrections.occur during southernspringtime.in calibration view data can be used to modify current stray light correction method to remove the3.1. strayNorthernHemisphereadditionallightfeatures that occur during southern hemisphere springtime.Figure 4 shows the typical EV dark signals (d–f) in the northern hemisphere DNB EV stray3.1. NorthernHemispherelight-affectedregions and the corresponding calibration views signals (a–c). In the EV plots (d–f) theestimated stray light and mean signals after correction are also plotted in red and blue, respectively.Figure4 shows the typical EV dark signals (d–f) in the northern hemisphere DNB EV strayFigure 4 shows that both EV and calibration view signals increased sharply in the penumbra region,light-affectedregions and the corresponding calibration views signals (a–c). In the EV plots (d–f) thewhen the spacecraft is transitioning from the dark to the bright part of the orbit. The recorded SVestimatedstrayandtomeanafter bothcorrectionare alsoplotted inbeforered andrespectively.signalsarelightsimilarthe EVsignalsdark signalsin patternsand magnitudethe blue,Earth sceneFigure 4entersshowsbothregion.EV andcalibrationsignals orbitincreasedsharplyin theanglepenumbrathethattwilightSinceSNPP is onviewa descendingand hasa declinationof 100 region,degrees,the Earthscene at end ofscanthe(EOS)willthe twilightearlierthanthewhen thespacecraftis transitioningfromdarktoobservethe brightpart of regionthe ltisshowninFigure4asthesharpEVsignalincreasedueto enterssignals are similar to the EV dark signals both in patterns and magnitude before the Earth scenetwilight happening at a lower solar zenith angle (SZA) at BOS (Figure 4d) than at EOS (Figure 4f).the twilight region. Since SNPP is on a descending orbit and has a declination angle of 100 degrees,The BB signals also showed similar patterns to SV and EV from penumbra to SZA 108 degreesthe Earthscene at endof thenscan gionsharpearlierthan thebeginning( cos(SZA)0.32),signalsslightlyincreasesat SZA 100 of thescan ncreaseduetotwilighthappeningdegrees ( cos(SZA) 0.18). The signal pause at SZA 108 degrees coincided with EOS twilight onsetat a (Figure4f).TheBBsignalsalsoangle, indicating the increased Earth shine might have contributed slightly to BB signals. Similar aseinthepenumbraregion,thenashowed similar patterns to SV and EV from penumbra to SZA « 108 degrees ( cos(SZA) « 0.32), thengradualdecreasebeforeincreasingagainat aroundBOS twilightonsetangleswhen theEarth view « 0.18).the signalswereslightlyelevatedbeforesharpincreasesat SZA« 100degrees( cos(SZA)
Remote Sens. 2016, 8, 1386 of 15The signal pause at SZA « 108 degrees coincided with EOS twilight onset angle, indicating theincreased Earth shine might have contributed slightly to BB signals. Similar to SV, BB, and EV, theSD signalsshow the initial sharp increase in the penumbra region, then a gradual decreasebeforeRemote Sens. 2016, 8, 1386 of 14increasing again at around BOS twilight onset angles when the Earth view entered the twilight region.enteredthe aretwilightregion.The SDofsignalsare at leastan ordermagnitudestrongerthan SV,The SDsignalsat leastan ordermagnitudestrongerthanofSV,BB, or EV,indicatingtheBB,bulk ectedstraylightofftheSDsurface.the signal likely is the reflected stray light off the SD surface.Figure4. DNBdetector8 igure4. DNBdetector8 EVandcalibrationcalibration viewview darknorthernhemispherestraystraylight lightproblemregionsOctober2013.(a–c)(a–c) showshow calibrationEVEVdarksignalsfor beginningofproblemregionson onOctober5, 5,2013.calibrationviews.views.darksignalsfor beginningof(BOS),nadir,of .BlackBlack curves:curves: darkscan scan(BOS),nadir,andandendendof scan(EOS)areareshownin rves: estimatedstray bluelight;curves:blue curves:signalsafterstraylightcorrection.SZASZA solarsignals;curves:stray angle.zenith angle.The current operational stray light correction methods approximate the stray lights as the EVThe currentoperationalstray lightmethodsapproximatethebystrayas theEV darkdarksignals minusthe airglow(red correctioncurves in Figure4d–f)approximatedthe lightsEV rved outside of the stray light affected regions [16,17]. In thre northern hemisphere, this methodperformedwell inremovingbulk of[16,17].the strayexcept forhemisphere,the penumbrathisregions.Sincetheoutsideof the straylightaffectedtheregionsInlight,thre northernmethodperformedsharpstray lightwithrespectto SZAin theregion,even a Sincesmall mismatchthe lightwell inremovingtheincreasesbulk of thestraylight,exceptfor penumbrathe penumbraregions.the sharpinstraypenumbraonset anglesbetweenimaging region,and LUTgenerationcould inincreasenoticeableonsetincreaseswith respectto SZAin thethepenumbraevena small timemismatchthe penumbraimage artifacts after correction [17]. The effects can be demonstrated in Figure 5, where the same imageangles between the imaging and LUT generation time could increase noticeable image artifacts afteris corrected using correction LUTs derived from three different months. Figure 5 shows the correctedcorrection [17]. The effects can be demonstrated in Figure 5, where the same image is corrected usingimage using the current month’s LUT has the best quality (Figure 5a), and the worst image (Figure 5b)correctionLUTs deriveddifferent5 showsthe correctedimagewas correctedusing thefrompriorthreemonth’sLUT. Tomonths.show theFigurepenumbraangle mismatchwas thecause,usingwe andtheworstimage(Figure5b)wascorrectedestimated the median penumbra angles in SV signal profiles (Figure 6). Based on the estimatedusingpenumbrathe priorangles,month’sTo showanglewas en thestraymismatchlight correctionLUTsand wethe estimatedscene in themedianpenumbrain SVforsignalprofiles(Figure6). TheBasedon penumbrathe estimatedangles,Figure5 is about angles0.07 degrees(b) and0.02 degreesfor (c).largeranglepenumbrabias results inworse imagequalitycorrection.Figure6 showsthat theestimatedpenumbraregionsthe p
additional stray light feature in the calibration view data and develop a revised correction method to remove the additional stray light occurring during the southern hemisphere springtime. Outside of current stray light