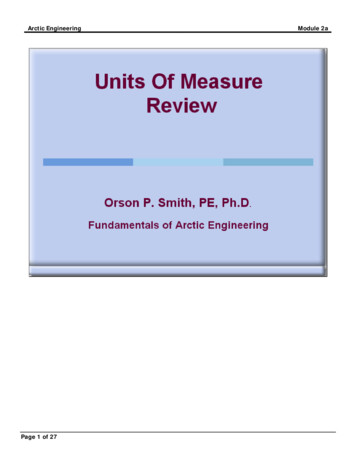
Transcription
Arctic EngineeringPage 1 of 27Module 2a
Arctic EngineeringPage 2 of 27Module 2a
Arctic EngineeringModule 2aBefore we look at fundamentals of heat transfer, this is a good time to review definitions of some essential physicalparameters and units of measure. We have in the class students of diverse educational background. We're all technicalpeople, but our everyday work may have led us away from some aspects of the measurement systems in use today bycold regions specialists. We'll take some time at this point to review the meanings of some useful terms and variables.Page 3 of 27
Arctic EngineeringModule 2aThe fundamental units of measure are mass, length, and time, abbreviated by the letters capital M, L, and T. All systemsof measure use seconds as the basic measure of time. Length is basically feet in British units and meters in theInternational SI or metric system. Centimeter, one one-hundredth of a meter, is also common.Mass is the measure of the inertia of an object, that is, its tendency to stay at rest or stay in motion. The British systemmeasures mass in slugs. The hybrid English engineering system measures mass in units of pound-mass. The SI systemuses mass units of kilograms and grams, a gram being one one-thousandth of a kilogram.Density is the measure of mass per unit volume. An object with a particular volume has a density of its total mass dividedby its volume, typically reported either in slugs per cubic foot, kilograms per cubic meter, or grams per cubic centimeter.Density is typically designated by the lower-case Greek letter rho.Page 4 of 27
Arctic EngineeringModule 2aSpecific gravity is a dimensionless relative measure that compares the density of a substance to the maximum density offresh water. The density of pure fresh water at standard atmospheric pressure and 4 degrees Celsius is 1.94 slugs percubic foot or 1,000 kilograms per cubic meter.Page 5 of 27
Arctic EngineeringModule 2aNewton's second law states that an object in motion tends to stay in motion and an object at rest tends to stay at rest,unless acted upon by an external force." We learned in physics class that this means "force equals mass timesacceleration." Acceleration has units of Length per Time squared, that is, feet per second squared or meters per secondsquared.Force, therefore, has units of Mass-Length per Time squared, such as slug-feet per second squared or kilogram-metersper second squared. Slug-feet per second squared are known as pounds force. Kilogram-meters per seconds squaredare known as Newtons. A dyne is a force of 1 gram-centimeter per second squared.The now-rarely-used English Engineering system, which measures mass as pound mass, requires a constant ofproportionality for definition of pounds force, related to the acceleration of standard Earth gravity. Weight, after all, is theforce of gravity on an object of a particular mass.Page 6 of 27
Arctic EngineeringModule 2aSpecific weight is similar to density in that it measures the weight of a substance per unit volume. Specific weight of asubstance, often designated by the Greek letter gamma, is computed by multiplying its mass density by the accelerationof gravity. Standard Earth gravity, designated as the lower-case letter g, is 32.2 feet per second squared or 9.81 metersper second-squared. Units of specific weight are pounds per cubic foot or Newtons per cubic meter. It is easy to confusespecific weight, which has units, with dimensionless specific gravity.Page 7 of 27
Arctic EngineeringModule 2aPressure is a phenomenon in fluids that distributes force over an exposed area, with basic units of pounds per square footor square inch or Newtons per square meter. One Newton per square meter is a Pascal. The pressure of Earth'satmosphere is included in most pressure measurements on the planet surface. Related units of measure includeatmospheres, multiples of standard atmospheric pressure, and bars, equal to one hundred thousand Pascals,approximately one standard atmosphere. Since atmospheric pressure involves so many Pascals, the unit kiloPascals,equal to one thousand Pascals, is in common use.Page 8 of 27
Arctic EngineeringModule 2aA more precise definition of the accepted standard atmospheric pressure is 101.3 kilo-Pascals or 14.7 pounds per squareinch. Many devices for measuring pressure include sensors that respond to differences in pressure between a specificarea and ambient atmospheric pressure. This difference in practice is known as gauge pressure. Most engineeringapplications implicitly apply gauge pressure, but occasionally it is important to note that total, or absolute pressure, equalsthe sum of gauge and atmospheric pressures. Gauge pressure is the pressure measured above (or below) atmosphericpressure. Absolute pressure includes atmospheric pressure. Absolute pressure gauge pressure atmospheric pressurePage 9 of 27
Arctic EngineeringModule 2aTransfer of mechanical energy is described with the concept of work, or force applied over a specific distance. The unitsof work, and of transfer of energy, in general, are those of force times length. Work is measured in either foot-pounds orNewton-meters. One Newton-meter is equivalent to one Joule. Power is defined as the rate of transfer of energy. In thecase of mechanical energy, power is work per unit time, with units of foot-pounds per second or Joules per second. Thetraditional horsepower units have an equivalent value in foot-pounds per second. One joule per second is equivalent toone watt. A kilowatt is one thousand watts.Page 10 of 27
Arctic EngineeringModule 2aTemperature is a measure of the energy-correlated vibration or motion of an object's component particles. The higher thetemperature, the more energetic are its component particles. When heat energy is added to a body, its internal energyincreases. The internal energy of a body decreases when heat is removed.Most materials expand with added internal energy and contract with decreased internal energy. Thermometers use theexpansion and contraction of a liquid, often mercury or alcohol, to measure temperature of a substance with which theyare in contact.The Fahrenheit scale of temperature measurement is based on the conventions that water freezes at 32 and boils at212 under atmospheric pressure.The international Celsius scale of temperature measurement is based on the conventions that water freezes at 0 andboils at 100 under atmospheric pressure.The Fahrenheit and Celsius scales can easily be converted one to the other.Page 11 of 27
Arctic EngineeringModule 2aAll objects with any internal energy have a measurable temperature. At very low temperatures, the Fahrenheit and Celsiusscales are less practical. A scale of absolute temperature must be applied in some physical relationships. Both Fahrenheitand Celsius have counterpart absolute temperatures scales. The Rankine scale has the same unit degree width as theFahrenheit scale. The Kelvin scale, in units of "Kelvins," is similarly associated with the Celsius scale.Page 12 of 27
Arctic EngineeringModule 2aInternal energy can be transferred from one object to another as heat energy. Basically, heat transfer is accomplished byradiation and absorption or by contact. The same units can quantify heat energy as mechanical energy, that is, footpounds or joules. Because heat energy has the effect of changing an object's temperature, some special units have comeinto practice. A British Thermal Unit, or BTU, is defined as the amount of heat energy to raise the temperature of onepound of water by one degree Fahrenheit. A calorie is defined as the amount of heat energy to raise the temperature ofone gram of water by one degree Celsius. A kilocalorie is defined as the amount of heat energy to raise the temperatureof one kilogram of water by one degree Celsius.Page 13 of 27
Arctic EngineeringModule 2aVarious substances respond differently to addition or removal of heat. The specific heat capacity of a substance is theamount of heat energy needed to change the temperature of a unit quantity by one degree Celsius or Fahrenheit.Pressure affects the heat capacity of fluids, especially gases; therefore heat capacity is defined two ways. Cp is the heatcapacity at constant pressure, and Cv is the heat capacity at constant volume.Page 14 of 27
Arctic EngineeringModule 2aWater has the highest heat capacity of all natural substances. Metals have low heat capacity, in comparison. Forexample, the heat capacity of iron, CFe, is 0.11 kcal/kg- C, which is low compared to 1 kcal/kg- C for water. It is importantfor cold regions engineers to note that ice and water vapor have lower heat capacities than liquid water.Page 15 of 27
Arctic EngineeringModule 2aHeat energy shed or absorbed by an object that results in a change of temperature is "sensible" by temperature sensors.The formula on this slide defines the relationship of sensible heat energy, Q, temperature change, Δ T, for an object ofmass, M, and heat capacity, C. When only liquids or solids are involved, the subscript of heat capacity is often dropped,since Cv and Cp are practically equivalent for liquids and solids.Page 16 of 27
Arctic EngineeringModule 2aThe amount of heat exchange required to produce a change of state in a substance is termed "latent heat" energy. Thisheat loss or gain does not result in a corresponding temperature change. It is instead observable in the change of state ofthe substance.Water at freezing temperature requires additional heat energy loss to freeze, or change from liquid to solid state. Oncefrozen, ice temperature can then decrease by sensible heat loss.Page 17 of 27
Arctic EngineeringModule 2aThe most common values applied in engineering computations for latent heat of fresh water are presented here.Page 18 of 27
Arctic EngineeringModule 2aThe illustration here shows how a gram of fresh water must be warmed or cooled between changes of state. Gain or lossof 100 calories as liquid water between the temperatures 100 and zero degrees Celsius results in sensible heat loss.Arctic engineers are primarily concerned with this and the 80 calories per gram latent heat of fusion required for the liquidsolid phase change.Page 19 of 27
Arctic EngineeringModule 2aA sample computation is presented here, to help understand the units of heat capacity and latent heat of fusion. The firstpart of the problem is to cool the water to freezing temperature by sensible heat loss. The second part is to removeenough latent heat to accomplish the change of state.A common error with such computations is failure to apply the units of measure consistently. If the units are treated asalgebraic variables, they should all "cancel" one another, except the desired resultant unit. This particular exampleillustrates the common practice of substituting weight in pounds for mass in kilograms, when working with BTU's andBritish units.Page 20 of 27
Arctic EngineeringModule 2aThis second example further illustrates application of heat capacity and latent heat. You should try this computation withall units specified, as in the previous example, to satisfy yourself that the answer is in kilograms.The ice must warm to melting temperature and then change to liquid state. The water is assumed to provide (andtherefore lose) this amount of heat energy, thus experiencing a sensible heat loss and drop in temperature. We specifythe change in water temperature in the equation and algebraically solve for the required mass of ice.Page 21 of 27
Arctic EngineeringModule 2aThe ideal gas law defines the combined effects of temperature and pressure on the density of a perfect gas. The equationapplies absolute temperature (K or R) and absolute pressure instead of gauge pressure. Fahrenheit and Celsiustemperatures and gauge pressure yield errors in ideal gas law applications.Page 22 of 27
Arctic EngineeringModule 2aThe ideal gas law is expressed various ways in texts, sometimes relying on alternative definitions of, R, the ideal gasconstant.Page 23 of 27
Arctic EngineeringModule 2aCold temperatures dramatically affect the ability of fluids to flow. Resistance to flow, or viscosity, increases astemperatures decrease. The ideal relationship in this slide states that the shear stress in a fluid is proportional to itsviscosity.Page 24 of 27
Arctic EngineeringModule 2aThe differential ratio du/dy represents the change in fluid flow velocity parallel to a stationary boundary with respect todistance away from the boundary. Fluid particles at the boundary are ideally assumed to have no velocity. In the sketch, aplate moves parallel to the fixed boundary. Fluid particles at the moving plate are assumed to have the velocity of theplate. A linear variation between is the characteristic behavior of a Newtonian fluid. Liquid water and oil usually behave asNewtonian fluids.Page 25 of 27
Arctic EngineeringModule 2aThe last parameter we'll discuss in this module relates a change in pressure on an object to its associated change involume and density. The differential quantity dp represents a change in pressure. The denominators here represent first arelative change in volume and a relative change in density. The bulk modulus of elasticity has a negative value withrespect to volume change, because a positive change in pressure is expected to produce a decrease in volume, that is, anegative relative change in volume. Density, on the other hand, is expected to increase with an increase in pressure. Thebulk modulus is a material property tabulated in texts and reference manuals.Page 26 of 27
Arctic EngineeringModule 2aThis concludes our brief review of units of measure. If you feel the need for further review, most any introductory physicstext will include the topics of this module. We'll move on in the next module to review of fundamental principles of heattransfer.Page 27 of 27
Arctic Engineering Module 2a Page 4 of 27 The fundamental units of measure are mass, length, and time, abbreviated by the letters capital M, L, and T. All systems of measure use seconds as the basic measure of time. Length is basically feet in British u