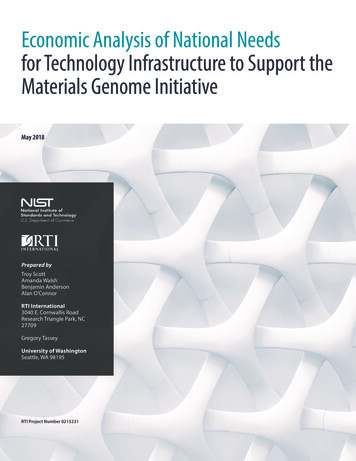
Transcription
Economic Analysis of National Needsfor Technology Infrastructure to Support theMaterials Genome InitiativeMay 2018Prepared byTroy ScottAmanda WalshBenjamin AndersonAlan O’ConnorRTI International3040 E. Cornwallis RoadResearch Triangle Park, NC27709Gregory TasseyUniversity of WashingtonSeattle, WA 98195RTI Project Number 0215231Economic Analysis of National Needsfor Technology Infrastructure to Support the Materials Genome Initiative Page 1
Analysis BriefThe MGI is a strategic effort spanning multiple federalagencies to promote a globally competitive U.S. manufacturing sector by addressing important gaps in the MaterialsInnovation Infrastructure1. Its aim is to enable U.S. companiesto more rapidly and efficiently develop and deploy advancedmaterials, with applications ranging from consumer goods, torenewable energy generation and energy storage, to supercomputing and national defense.This briefing provides key findings from economic analysis and interviews with more than 120 industry experts ontheir needs for new technological infrastructure supportingadvanced materials innovation and the potential economicimpacts of meeting those needs. We conclude that animproved Materials Innovation Infrastructure woulddeliver between 123 billion and 270 billion in valueannually.The National Institute of Standards and Technology (NIST) issupporting the MGI through efforts to establishABOUT THE MATERIALS GENOME INITIATIVE m ate rials data-exchange and model-exchange protocols; t he means to ensure the quality of materials data andmodels; and n ew methods, metrologies, and capabilities needed foraccelerated materials development.Additionally, through its integration of these activities, NISTis working to test and disseminate elements of an improvedMaterials Innovation Infrastructure to stakeholders in othernational laboratories, universities, and U.S. industry.The aim of the Materials Genome Initiativeis to enable U.S. industry to develop anddeploy advanced materials more quicklyand efficiently.Advanced materials are an increasingly essential—andincreasingly complex—component of the manufacturingenvironment, forming the first tier of advanced manufacturing supply chains.2 This complexity demands larger, morediversified, and integrated R&D resources, which are noteasily defined and implemented.3Recognizing this challenge, more industrialized nations aremaking larger and more sophisticated investments in newmaterials and their product applications, especially in materials R&D infrastructure. 123Billion per year. 270NIST is a key player in supporting the MGI approach and the development of a national Materials InnovationInfrastructure. This analysis presents estimates of potential impacts attributable to improved infrastructure ofbetween 123 billion and 270 billion per year.1 To make this analysis tractable, the central concept of a Materials Innovation Infrastructure was reduced to the six industry needs summarized in Table 1.2 Moskowitz, S. L. (2014). The advanced materials revolution: Technology and economic growth in the age of globalization. John Wiley & Sons, Inc., New York.3 Tassey, G. (2016). The technology element model, path-dependent growth, and innovation policy. Economics of Innovation and New Technology, 25(6), 594-612.Economic Analysis of National Needsfor Technology Infrastructure to Support the Materials Genome Initiative Page 2
an advanced material from laboratory to market could significantly improve U.S. global competitiveness and ensure thatthe United States remains at the forefront of the advancedmaterials marketplace.The MGI “offers a unique opportunityfor the United States to discover,develop, manufacture, and deployadvanced materials at least twice asfast as possible today, at a fraction ofthe cost” (NSTC, 2011).Examples include the Industrial Technology ResearchInstitute of Taiwan, the Electronics and TelecommunicationsResearch Institute of South Korea, the Fraunhofer appliedresearch institutes in Germany, and, in the United States,the National Network for Manufacturing Innovation(Manufacturing USA), Advanced Manufacturing TechnologyConsortia, National Nanotechnology Initiative, the MGI, andother initiatives.4Launched in 2011 as a key enabling element of a strategyto spark domestic competitiveness in manufacturing inhigh-demand and emerging technology markets, the MGI is a“multi-stakeholder effort to develop infrastructure to accelerate advanced materials discovery and development in theUnited States . . . [and] leverage existing Federal investmentsthrough the use of computational capabilities, data management, and an integrated approach to materials science andengineering.” 5Motivating the initiative are two ideas: first, that developingadvanced materials is critical to addressing challenges insectors like energy, supercomputing, national security, andhealthcare; second, that accelerating the process of moving4567Released in 2014, the MGI strategic plan sets out four defininggoals for the initiative: 6 C ulture change: MGI-aligned efforts will aim to improveknowledge flows and break down traditional silos inmaterials science and engineering, integrating theefforts of theorists and experimentalists, and promotingcollaboration among academia, national and federallaboratories, and industry. I ntegration of experiments, computation, and theory:A defining feature of MGI-aligned efforts is an integrated,collaborative workflow that draws simultaneously fromexperiments, computation, and theory. The MaterialsInnovation Infrastructure envisioned by the MGI includesadvanced simulation tools validated through experimental data, networks to share useful modeling and analysiscode, and access to quantitative synthesis and characterization tools. A ccess to digital data: MGI-aligned efforts will expandaccess to validated data and tools generated by thematerials community across the materials developmentcontinuum. W orkforce development: To prepare the next generation of materials scientists and engineers to leverage anew Materials Innovation Infrastructure and apply theintegrated, systems approach to materials innovation itenables, MGI-aligned efforts will support undergraduateand graduate-level curriculum development togetherwith workforce development and training for professionals in the workplace.Leading the MGI are the Department of Defense, Departmentof Energy, National Science Foundation, and NIST. Otheragency partners in the MGI include the National Aeronauticsand Space Administration; National Institutes of Health; andU.S. Geological Survey, Department of the Interior.7See www.manufacturing.gov/programs.National Science and Technology Council (NSTC). (June 2011). Materials Genome Initiative for Global Competitiveness.National Science and Technology Council (NSTC). (December 2014). Materials Genome Initiative Strategic Plan.See https://www.mgi.gov/partners.Economic Analysis of National Needsfor Technology Infrastructure to Support the Materials Genome Initiative Page 3
Table 1. Technology Infrastructure Needs for Advanced Materials InnovationINDUSTRY NEEDAccess to High-Quality DataNonproprietary experimental data,computational data, metadata, andsoftware codeEXAMPLES OF INFRASTRUCTURETECHNOLOGY TO ADDRESS NEED F undamental materials dataPOTENTIAL IMPACTS D ata standardization and curation M ore easily leverage prior research withless duplication of effort M odels underpinning accurate andrepeatable material measurement E nable greater reliance on more efficientcomputational approaches M ultiply the value of every other elementof a Materials Innovation InfrastructureCollaborative NetworksEfficient means of sharing materialsinformation (e.g., along a supplychain, among research collaborators)Material Design MethodsEnabling application of a systemsapproach to materials development,from discovery and design all the waythrough to deployment Methods for capturing, characterizing,and sharing materials data in structuredformats C ommunication standards and translators(“MT Connect for material measurementequipment”) Models, simulations, and metrologies foradvanced materials design and means ofintegrating tools with one another. Machine learning tools A lign academic and public-sector researchto industry-relevant challenges I ntegrate experimental measurement andcomputational modeling to improve modelfidelity and overall utility R ealize network externalities E nable more targeted searches of designspace for promising candidate materials E nable purposeful design of materials tomeet specific performance requirementsfor targeted applications T arget significant performanceimprovements with more-novel materials,as opposed to seeking smaller incrementalimprovements by refining known materials E nable co-design of new materials andnew product applicationsProduction & Scale-UpModel-based alternatives to expensivephysical testing, trial and error–basedapproachesFaster, cost-effective means ofproducing advanced materials at pilotand full scalesEconomic Analysis of National Needsfor Technology Infrastructure to Support the Materials Genome Initiative Multiscale modeling frameworks(integrating macroscopic process modelswith microscopic materials simulation) R educe trial and error when scaling up(from lab scale to pilot scale, from pilotscale to production scale) Process technology platforms (e.g., coldsintering, additive manufacturing, roll-toroll printing, directed self-assembly) A llow consideration of production-scaleprocesses to be integrated into the initialdesign process O vercome the “Valley of Death” betweenlab scale and production scale: pilot-scalemanufacturing services and facilities areunderprovided by the market Page 4
Table 1. Technology Infrastructure Needs for Advanced Materials Innovation (continued)EXAMPLES OF INFRASTRUCTURETECHNOLOGY TO ADDRESS NEEDPOTENTIAL IMPACTSQuality Assurance, Quality Control& Component CertificationAbility to model, predict, and controlformation of defectsAbility to forecast manufacturingvariation Performance metrics (benchmarks,reference data, testbeds to characterizeperformance of systems andcomponents) R educe the cost of controlling andverifying the performance attributesof materials—and components andproducts embodying those materials Process control tools (test protocols,objective scientific and engineering data,reference databases) R educe the risk of large costs incurredif defects are not detected and lead toproduct failures in use (e.g., lithium-ionbattery fires)Model Validation & UncertaintyQuantificationBasis for trust and acceptance ofcomputational modelsBasis for objective decision-makingregarding reliance on computationalanalysis and simulation at a businesslevel Generally accepted and easily appliedmethods for uncertainty quantificationfor both experimental and computationaldata E nhance the utility of computationalapproaches from an engineeringperspectiveINDUSTRY NEED V alidation of analytical methods andprocedures, emphasizing industriallyrelevant systems, comparing predictedand measured properties from multiplesources E nable rational decision-makingregarding computational approachesfrom a business perspective A dvance industry’s reliance oncomputational approaches in situationswhere they can save cost and add valueINDUSTRY NEEDS ASSESSMENTSix identified areas of industry need provided the commonbasis for the more than 120 interviews that support thisanalysis: access to high-quality data; collaborative networks; material design methods; production and scale-up; q uality assurance, quality control, and componentcertification; and m odel validation and uncertainty quantification (Figures 1and 2).the strong complementarity among the six areas of need andthe consequent overlap among the types of infrastructureand potential impacts: efforts to address one need tend toincrease the return on efforts to address the others. But thecomplementarity was not completely symmetrical: access tohigh-quality data was perceived to play a pivotal role, being aprerequisite for model validation and uncertainty quantification and for the productive application of machine learning,modeling and simulation, and other elements of an envisioned Materials Innovation Infrastructure. The upshot is thataddressing industry’s need for high-quality, nonproprietarydigital data can be expected to lower the barriers—faced byboth the public and the private sectors—to addressing theother areas of need.Access to high-quality data emerged as a linchpin of aMaterials Innovation Infrastructure. Industry experts stressedEconomic Analysis of National Needsfor Technology Infrastructure to Support the Materials Genome Initiative Page 5
Figure 1. Interviewees’ Rating of Importance of Technology Infrastructure Needs5 - critically43%4 - very45%3 - moderately34%2 - somewhat1 - not 5%9%Model Validation &Uncertainty Quantification(mean: 3.9)Materials Design Methods(mean: 3.7)Access toHigh-Quality Data(mean: 4.1)7%3%Quality Assurance &Component Certification(mean: 4.1)30%27%43%16%12%9%2%9%Production & Scale-Up(mean: 3.8)Collaborative Networks(mean: 3.5)Note: Percentages shown reflect the distribution of ratings. Average ratings are given in parentheses below each area of industry need.Figure 2. Interviewees’ Rating of Difficulty of Meeting Needs through Private Investment5 - prohibitively19%4 - very18%3 - moderately21%2 - somewhat21%1 - not 5%5%11%5%42%4%42%25%34%19%11%Access toHigh-Quality Data(mean: 3.6)Quality Assurance &Component Certification(mean: 3.5)Model Validation &Uncertainty Quantification(mean: 3.7)Materials Design Methods(mean: 3.7)Production & Scale-Up(mean: 3.6)Collaborative Networks(mean: 3.1)Note: Percentages shown reflect the distribution of ratings. Average ratings are given in parentheses below each area of industry need.Economic Analysis of National Needsfor Technology Infrastructure to Support the Materials Genome Initiative Page 6
IMPACT ON RISKCompanies developing new materials face the risk that aresearch and development (R&D) project will fail to reachdeployment and generate investment returns. We estimatethat the total risk could be reduced by almost half withimproved infrastructure: for each new material deployed,only 5 R&D projects would need to enter the R&D pipeline atthe discovery/design stage, down from an estimated 9.8 inthe current environment (Figure 3).Figure 3. Potential Impact on Risk10.09.89.0R&D Projects in Stage per Deployment8.07.0Existing Infrastructure6.05.05.04.02.93.02.0Improved velopmentManufacturingDeploymentNote: With improved infrastructure, materials R&D projects are more likely to transition to successive stages. The number of projects that must enter the R&Dpipeline at the discovery/design stage for each one that successfully reaches the final deployment stage improves from 9.8 to 5.0 (a 20% chance of deploymentwith improved infrastructure versus a 10% chance currently). The number of projects that must enter the development stage for every one that reaches thedeployment stage improves from 2.9 to 2.1 (a 48% chance of deployment, conditional on reaching the development stage, with improved infrastructure versus a35% chance currently).IMPACT ON TIME TO MARKETWe estimate that development of a new material takes onaverage more than 10 years and that an average accelerationof 3.5 years could be possible with improved infrastructure(Figure 4).Figure 4. Potential Impact on Time to Market ation3.024Deployment2.062.581012Note: U.S. manufacturers would be able to bring new materials to market faster with the benefit of improved infrastructure. Averagetime to market is estimated to be 6.6 years with improved infrastructure compared with 10.2 years in the current environment.Economic Analysis of National Needsfor Technology Infrastructure to Support the Materials Genome Initiative Page 7
IMPACT ON RELATIVE COSTS PER PROJECTPER YEARWe estimate that improved infrastructure has the potentialto reduce relative costs by an average of 25% in the discovery/design stage, 45% in the development stage, 48% inthe manufacturing stage, and 28% in the deployment stage(Figure 5).Overall, estimated potential impacts of an improved MaterialsInnovation Infrastructure achieve a 71% improvement inR&D efficiency, worth an estimated 39 billion to 69 billionper year to U.S. companies that comprise the new materialssupply chain (Table 2).8Beyond increasing R&D efficiency,improved infrastructure couldalso create new opportunities forcompanies to improve productquality and performance, expandproduct offerings, and enter newmarkets.Figure 5. Potential Impact on Relative R&D Cost per Project per Year35DeploymentManufacturing30Relative Cost per Project per rovedInfrastructureNote: Cost per project, per year, in the discovery/design stage in the currentenvironment was normalized at 1.0. In the current environment, manufacturing and deployment stages are estimated to be, respectively, roughlyfour times and three times more cost-intensive than the development stage,which is, in turn, roughly four times more cost-intensive than the discovery/design stage. Improved infrastructure is estimated to reduce relative costs byan average of 25% in the discovery/design stage, 45% in the developmentstage, 48% in the manufacturing stage, and 28% in the deployment stage.IMPACTS ON PRODUCT QUALITY ANDPERFORMANCEThe potential benefits of an improved Materials InnovationInfrastructure do not stop at raising R&D efficiency. Theimproved infrastructure could also enable companies toundertake R&D projects they would not otherwise have done,leverage that R&D to commercialize improved products andnew product lines, and expand into new markets. Not onlywould the expected R&D cost be lower for a given R&D result,the anticipated R&D result would also be superior.One way in which this could happen is by enabling companies to incorporate new materials into the product designprocess earlier, so that new product applications take fulladvantage of new materials’ capabilities. Going even further,computational materials design methods, fed with high-quality, nonproprietary digital data, could enable co-design ofnew materials and new product applications.We estimate the value of these additional benefits to beroughly 2 to 3 times the value of potential R&D efficiencyimpacts, or between 4% and 9% of the annual value addedfor the industries considered (i.e., 4% to 9% of these industries’ contributions to U.S. gross domestic product).8 The estimated 71% improvement in R&D efficiency is the percentage reduction in average R&D investment cost per new material deployed. The range of 39 billion to 69 billion peryear in potential impacts represents between 15% and 25% of R&D investment in the industries considered.Economic Analysis of National Needsfor Technology Infrastructure to Support the Materials Genome Initiative Page 8
TOTAL ECONOMIC IMPACTSAltogether, the potential economic benefits of an improvedMaterials Innovation Infrastructure are estimated to be worthbetween 123 billion and 270 billion per year (Table 2).Public investment is needed to create a Materials InnovationInfrastructure. To explain the difficulty of addressing infrastructure needs solely through private investment and sojustify the need for public investment in Materials InnovationInfrastructure, industry experts emphasized the public-goodcontent of this infrastructure and the multidisciplinarityrequired to develop it.Nonproprietary data is an example of a public good. Arepository of measu
Jun 26, 2018 · the National Network for Manufacturing Innovation (Manufacturing USA), Advanced Manufacturing Technology Consortia, National Nanotechnology Initiative, the MGI, and other initiatives. 4 Launched in 2011 as a key enabling element of a strategy to spark dome