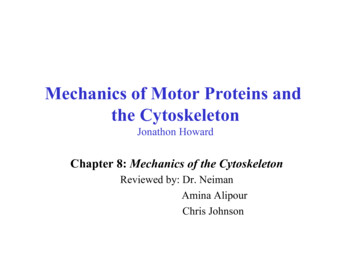
Transcription
Mechanics of Motor Proteins andthe CytoskeletonJonathon HowardChapter 8: Mechanics of the CytoskeletonReviewed by: Dr. NeimanAmina AlipourChris Johnson
Rigidity of Actin Filaments in VitroNagashima 1980: The flexural rigidity was first measured from observations ofthermal fluctuations in curvature of actin filamentsThe first real timesingle-molecule mechanical measurements.Lp 12 µmE 1.7 GPaLp EIkTKojima 1994: Longitudinal stiffness bymicromanipulation of individual filamentsL 1 µmk 44 pN/nm; E 2.3 GPaFigure 8.4: Thermal fluctuations of an actin filamentYasuda 1996: Torsional rigidity from thermally driven torsional fluctuation(EI)torsional 28 10-27 Nm2G 0.5 GPa; E 1.5 GPaThe persistence length is a basic mechanical property quantifying thestiffness of a polymer.
PhalloidinThe property of phalloidin is auseful tool for investigating thedistribution of F-actin in cells bylabelingphalloidinwithfluorescent analogs and usingthem to stain actin filaments forlight microscopy. Fluorescentderivatives of phalloidin haveturned out to be enormouslyuseful in localizing actinfilaments in living or fixed cellsas well as for visualizingindividual actin filaments invitro.Fluorescent phalloidin (red) markingactin filaments in endothelial cells
Rigidity of Actin Filaments in VitroActinThin filament:Tropomyosin- Tropomyosin increases the crosssectional area and mass of the filamentby 22%: It increases the longitudinalstiffness by 22% and the flexuralrigidity by 50% (Kojima et al. 1994;Isambert et al. 1995).Results: This leads to a close agreement between the Young’s modulus of thethin-filament protein calculated from the measurements on muscle (2.3 GPa)and that calculated from the measurements on reconstituted actin-tropomysinfilaments (2.8 GPa by Kojima et al. 1994; 2.0 GPa by Isambert et al. 1995).
Rigidity of Microtubules in VitroThe most accurate measurementsResults:(EI) 26 2 10-24 Nm2E 1.9 GPa.Sufficiently high to explain the rigidity ofmicrotubules in sperm. There is nophysiological requirement for an agent oradditional proteins to make microtubules morerigid in vivo.Figure 8.5: Measurement of theflexural rigidity of a microtubule
Rigidity of Microtubules in VitroResults:Microtubule –associated proteins (MAPs) add only 10% additional mass to themicrotubuleThey would be expected to increase the longitudinal stiffnessby only 10% and the flexural rigidity by 20%.Mickey 1995; Kurz 1995: Neither tau nor bovine brain MAPs have anappreciable effect on microtubule rigidity.
Rigidity of Microtubules in VitroFelgner 1996: MAPs increased the flexural rigidity of naked microtubulesapproximately 4-fold.One possibility: The lower value measured by Felgner et al. in the absenceof MAPs is due to structural defects in the walls of their unstabilizedmicrotubules, which were observed in a nonphysiological buffer. Bystabilizing these microtubules against such defects, the MAPs might haveincreased the flexural rigidity to a value in agreement with the other values.Results: The nonhydrolyzable nucleotide analogue GMP-CPP increases therigidity of microtubules by about a factor of two.Isambert 1995: The phosphate analogue BeF3- increases the stiffness ofactin filaments by 50%.
Rigidity of Coiled Coils in VitroPhillips 1996: coiled-coil protein tropomyosin; Lp 50 to 200 nmHvidt 1982: coiled-coil tail of myosin and paramyosin; Lp 130 nmHoward 1996: coiled coil tail of myosin tails adsorbed to EM grids; Lp 100 nmThis is a lower limit because adhesive forces might bend the molecules as theybind to the EM grid.Suezaki 1976: Theoretical calculations for polyglycine and polyalanine α-helicesLp 40 and Lp 65 nm, respectively.For dimeric coiled coil (twice the cross-sectional area); Lp 160-260 nm.Conclusion: For a hydrated coiled coil; Lp 100-200 nmE 2 GPaThis is sufficiently high to account for the rigidity of the hydrated keratincontaining materials.
Rigidity of Intermediate Filaments in VitroHeins 1993: Electron micrographs for neurofilaments Lp 1 µmInagaki 1989: Electron micrographs for vimentin Lp 3 µmHohenadl 1999: light-scattering experiments on desmin Lp 1 µmConclusion:If the 16 coiled coils in each cross-section of an intermediate filament weretightly crosslinked: Lp 25-50 µm (162 times greater than that of an individualcoiled coil). If they were not crosslinked: Lp 1.6-3 µm (only 16 times greater).In hydrated intermediate filaments there is little crosslinking and slippagebetween neighboring coiled coils: The result is a mechanical structure which ismuch less resistant to bending and twistingstring or rope
Rigidity of Bacterial Flagellum in VitroCalladine 1983: Due to an unusual deviation fromhelical symmetry, the flagellum adopts a coiledshape, like a tiny spring.Fujime 1972: light scattering for measuring theflexural rigidity:(EI) 2.2-4 10-24 Nm2E 0.5-0.9 GPaHoshikawa 1985: Hydrodynamic methodG 0.5 GPaE 1.5 GPaConclusion:The rigidity of the flagellar protein is similar tothat of the cytoskeletal proteins, and the similarityof values for the Young’s modulus measured usingthe different approaches is consistent with theflagellum being mechanically isotropic.Examples of bacterial flagellaarrangement schemes:A-Monotrichous; B-Lophotrichous;C-Amphitrichous; D-Peritrichous
Rigidity of DNA and Titin in VitroSmith 1992; Bustamante 1994: Single-molecule techniques for DNAKellermayer 1997; Rief 1997; Tskhovrebova 1997; Carrion-Vazquez 1999:Single-molecule techniques for muscle protein titinBustamante 1994: The extensioncurve for DNA resembles theLangevin function, which describesa freely joined chain.Figure 8.6: The force-extension curve for DNA
Summary1- The rigidity of purified actin filaments and microtubules measured in vitroaccounts well for the rigidity of the corresponding cytoskeletal filaments incells. Therefore actin-binding proteins and microtubule-associated proteinshave a comparatively small effect on rigidity, as expected, given that theycontribute only a small fraction of the total mass of the filaments.2- To a first approximation, the cytoskeletal proteins behave as isotropic,homogenous materials. This is supported by experiments on actin filamentsshowing that the stretching stiffness, the bending stiffness, and the torsionalstiffness all yield similar values for the Young’s modulus. Likewise the flexuraland torsional rigidity of bacterial flagella give similar Young’s modulus.Furthermore, the rigidity of dry hair is similar in the longitudinal and transversedirections, and the flexural and torsional rigidities of wool are also consistentwith isotropy. The main exception to this general statement is that hydratedkeratinaceous materials as well as individual intermediate filaments are notisotropic; instead, there appears to be considerable shear between theconstituent coiled coils.
Summary3- Hydrated actin, tubulin, flagellin, and coiled-coil proteins all have Young’smoduli in the range 1 to 3 GPa, similar to that of hard plastics, collagen (themajor constituent of tendons), and silk. The finding that the cytoskeletalproteins are among the most rigid proteins known supports the hypothesis thatthese proteins have evolved to serve structural roles in cells. Because theproteins are unrelated in sequence and structure, high protein rigidity hasprobably evolved independently a number of times.4- The absolute value of the Young’s modulus of these rigid proteins is within afactor of 2 of the Young’s modulus of a van der Waals solid. Thus we infer thatthe compliance of these proteins is primarily determined by the compliance ofthe van der Waals bonds that hold the polypeptide chains in their foldedconformation. This is expected given that van der Waals forces are the weaklinks in protein structures and will therefore tend to dominate the compliance.
Mechanics of Motor Proteins and . Chapter 8: Mechanics of the Cytoskeleton Reviewed by: Dr. Neiman Amina Alipour Chris Johnson . Nagashima 1980: The flexural rigidity was first measured from observations of thermal fluctuations in curvature of actin filaments The first real time single-molecule mechanical measurements. .