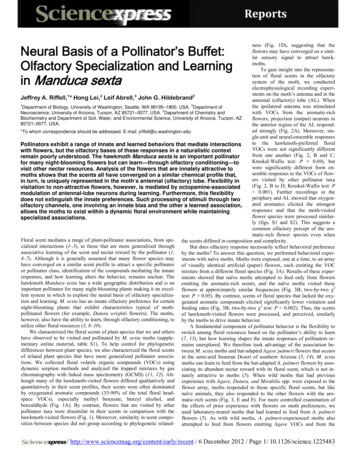
Transcription
ReportsNeural Basis of a Pollinator’s Buffet:Olfactory Specialization and Learningin Manduca sextaness (Fig. 1D), suggesting that theflowers may have converged on a similar sensory signal to attract hawkmoths.To gain insight into the representation of floral scents in the olfactorysystem of the moth, we conductedelectrophysiological recording experiments on the moth’s antenna and in the1232Jeffrey A. Riffell, * Hong Lei, Leif Abrell, John G. Hildebrandantennal (olfactory) lobe (AL). When12the ipsilateral antenna was stimulatedDepartment of Biology, University of Washington, Seattle, WA 98195–1800, USA. Department of3with VOCs from the aromatic-richNeuroscience, University of Arizona, Tucson, AZ 85721–0077, USA. Department of Chemistry andBiochemistry and Department of Soil, Water, and Environmental Science, University of Arizona, Tucson, AZflowers, projection (output) neurons in85721–0077, USA.the anterior region of the AL responded strongly (Fig. 2A). Moreover, sin*To whom correspondence should be addressed. E-mail: jriffell@u.washington.edugle-unit and neural-ensemble responsesto the hawkmoth-preferred floralPollinators exhibit a range of innate and learned behaviors that mediate interactionsVOCs were not significantly differentwith flowers, but the olfactory bases of these responses in a naturalistic contextfrom one another (Fig. 2, B and C;remain poorly understood. The hawkmoth Manduca sexta is an important pollinatorKruskal-Wallis test: P 0.69), butfor many night-blooming flowers but can learn—through olfactory conditioning—towere significantly different from envisit other nectar resources. Analysis of the flowers that are innately attractive tosemble responses to the VOCs of flowmoths shows that the scents all have converged on a similar chemical profile that,ers visited by other pollinator taxain turn, is uniquely represented in the moth‘s antennal (olfactory) lobe. Flexibility in(Fig. 2, B to D; Kruskal-Wallis test: Pvisitation to non-attractive flowers, however, is mediated by octopamine-associated 0.001). Further recordings in themodulation of antennal-lobe neurons during learning. Furthermore, this flexibilityperiphery and AL showed that oxygendoes not extinguish the innate preferences. Such processing of stimuli through twoated aromatics elicited the strongestolfactory channels, one involving an innate bias and the other a learned association,responses and that the moth-visitedallows the moths to exist within a dynamic floral environment while maintainingflower species were processed similarspecialized associations.ly (figs. S1 and S2). This suggests acommon olfactory percept of the aromatic-rich flower species even whenFloral scent mediates a range of plant-pollinator associations, from spe- the scents differed in composition and complexity.cialized interactions (1–3), to those that are more generalized throughBut does olfactory response necessarily reflect behavioral preferenceassociative learning of the scent and nectar reward by the pollinator (1, by the moths? To answer this question, we performed behavioral exper4–7). Although it is generally assumed that many flower species may iments with naïve moths. Moths were exposed, one at a time, to an arrayhave converged on a similar scent profile to attract a specific pollinator of visually identical artificial (paper) flowers, each emitting the VOCor pollinator class, identification of the compounds mediating the innate mixture from a different floral species (Fig. 3A). Results of these experresponses, and how learning alters the behavior, remains unclear. The iments showed that naïve moths attempted to feed only from flowershawkmoth Manduca sexta has a wide geographic distribution and is an emitting the aromatic-rich scents, and the naïve moths visited theseimportant pollinator for many night-blooming plants making it an excel- flowers at approximately similar frequencies (Fig. 3B; two-by-two χ2lent system in which to explore the neural basis of olfactory specializa- test: P 0.05). By contrast, scents of floral species that lacked the oxytion and learning. M. sexta has an innate olfactory preference for certain genated aromatic compounds elicited significantly lower visitation andnight-blooming plants that exhibit characteristics typical of moth- feeding rates (Fig. 3B; two-by-two χ2 test: P 0.002). Thus, the scentspollinated flowers (for example, Datura wrightii flowers). The moths, of hawkmoth-visited flowers were processed, and perceived, similarlyhowever, also have the ability to learn, through olfactory conditioning, to by the moths to drive innate behavior.utilize other floral resources (5, 8–10).A fundamental component of pollinator behavior is the flexibility toWe characterized the floral scents of plant species that we and others switch among floral resources based on the pollinator’s ability to learnhave observed to be visited and pollinated by M. sexta moths (supple- (7, 13), but how learning shapes the innate responses of pollinators rementary online material, table S1). To help control for phylogenetic mains unexplored. We therefore took advantage of the association bedifferences between plant species, we also characterized the floral scents tween M. sexta moths and bat-adapted Agave palmeri flowers that occursof related plant species that have more generalized pollinator associa- in the semi-arid Sonoran Desert of southern Arizona (5, 14). M. sextations. We collected floral volatile organic compounds (VOCs) using moths can learn to feed from the bat-adapted A. palmeri flowers by assodynamic sorption methods and analyzed the trapped mixtures by gas ciating its abundant nectar reward with its floral scent, which is not inchromatography with linked mass spectrometry (GCMS) (11, 12). Alt- nately attractive to moths (5). When wild moths that had previoushough many of the hawkmoth-visited flowers differed qualitatively and experience with Agave, Datura, and Mirabilis spp. were exposed to thequantitatively in their scent profiles, their scents were often dominated flower array, moths responded to those specific floral scents, but likeby oxygenated aromatic compounds (35-90% of the total floral head- naïve animals, they also responded to the other flowers with the arospace VOCs), especially methyl benzoate, benzyl alcohol, and matic-rich scents (Fig. 3, E and F). For more controlled examination ofbenzaldhyde (Fig. 1A). By contrast, flowers that are visited by other the effects of prior experience with flowers on moth preferences, wepollinator taxa were dissimilar in their scents in comparison with the used laboratory-reared moths that had learned to feed from A. palmerihawkmoth-visited flowers (Fig. 1). Moreover, similarity in scent compo- flowers (5). As with wild moths, A. palmeri-experienced moths alsosition between species did not group according to phylogenetic related- attempted to feed from flowers emitting Agave VOCs and from the/ http://www.sciencemag.org/content/early/recent / 6 December 2012 / Page 1/ 10.1126/science.1225483
hawkmoth flowers (table S5). Thus olfactory conditioning providedmoths with flexibility in their foraging behavior but did not extinguishtheir innate preferences for scents from the moth-pollinated flowers.To determine how the olfactory system processes odor informationas the moth learns, we performed experiments involving simultaneousmulti-channel AL recording and olfactory conditioning (Fig. 4A and fig.S3) (15). This approach allowed us to correlate the neural and behavioralresponses directly as the animal learned the association between the A.palmeri scent and sugar reward (fig. S3) (16, 17). After 3 training trials,the moths exhibited significantly increased proboscis-movement responses (PMR) from 0 to 33% (χ2 test: P 0.01). The ability to learn thescent association was directly correlated with the change in AL neuralresponses evoked by the VOCs (Fig. 4B). As the moths learned, thefiring rate of AL neurons significantly increased, response latency decreased, firing synchrony between neurons increased, and the pattern ofneural activity by the ensemble changed significantly (Fig 4 and fig. S4;Kruskal-Wallis test: P 0.02). By contrast, moths that were not rewarded or for which the reward was paired randomly with VOC stimulationhad significantly lower PMR than the trained moths and did not learn theassociation (0% PER for both treatments; χ2 test: P 0.001). Furthermore, AL neuronal activity in the control moths did not change significantly over the course of the experiment (fig. S4; Kruskal-Wallis testwith multiple comparisons: P 0.10). Last, differential conditioningexperiments were conducted to determine how learning influences thecoding of a scent paired with a reward (CS ; A. palmeri) versus an unpaired scent (CS-; Carnegiea gigantea). These experiments demonstrated that moths could readily learn to discriminate between the twocomplex scents, and that modulation of the AL ensemble served to increase the difference in representation of the two scents (fig. S5;Kruskal-Wallis test: P 0.05). Thus, learning the association between anecologically important floral scent and sugar-water reward significantlymodified AL neural responses and increases the contrast between scents.The change in the AL associated with learning leads to the questionof how this plasticity occurs. Biogenic amines, in particular octopamineand dopamine, have been shown in other insects to participate in theacquisition of olfactory memory and modulation of AL neural circuitry(18–21). Because putative receptors for octopamine and dopamine areexpressed in the M. sexta AL (10, 22), we injected receptor antagonistsor agonists into the AL before training the moths to associate the A.palmeri VOCs with a sugar reward. Results of these experimentsdemonstrated that microinjection of dopamine-receptor antagonists(Fluphenazine [10-8 M], Le300 [10-6 M] or SCH23390 [10-6 M]) had noeffect on the ability of moths to associate the reward with the A. palmeriodor and were not significantly different from the results obtained withcontrol moths (two-by-two χ2 test: P 0.55). By contrast, injection ofoctopamine-receptor antagonists (Epinastine, or Mianserine [10-6 M])prevented the moths from learning the odor-reward association and elicited significantly lower PMR compared to the vehicle control (Fig. 4F;two-by-two χ2 test: P 0.01). Moreover, when octopamine (10-5 M) wasinjected into the AL and moths were stimulated with the VOCs without apaired sugar reward, these moths exhibited significantly higher PMR inresponse to the VOC stimulus than did moths that were injected with thevehicle (Fig. 4F; two-by-two χ2 test: P 0.001). Finally, superfusion ofoctopamine (10-5 or 10-6 M) onto the AL during multi-channel andjuxtacellular neural recordings elicited effects similar to those observedwhen the moth learned. Neurons showed increased VOC-evoked firingrates, response latencies decreased, and firing synchrony between neurons increased (Fig. 4G and fig. S6). Thus octopamine apparently acts asa key neuromodulator in the moth’s olfactory pathway to mediate interactions with flowers in the field.When considering the effects of learning on innate preferences, it isimportant to examine the strength or evolutionary importance of theassociation. Our results show that learning does not extinguish responsesto the innately attractive floral scents and thus suggest that olfactoryconditioning may operate in an olfactory “channel” separate from, butparallel to, that involved in the innate responses. In the case of M. sexta,many but not all of the flowers that elicit innate responses are alsoSolanaceous plants that are hostplants for ovipositing females. In suchcases the flower may signal an appropriate host, or a site at which tolocate a mate, and thus the strength of the association may be a functionof the important life-history events that are unrelated to the immediateforaging responses of the moths. However, some of the flowers that emitthe aromatic-rich scents that elicit innate responses are not host plants,which raise the question of how these diverse species have evolved similar floral scents to attract hawkmoths. We here provide impetus for suchfuture work by showing that moths perceive these diverse floral speciessimilarly.References and Notes1. R. A. Raguso, Wake up and smell the roses: the ecology and evolution of oi:10.1146/annurev.ecolsys.38.091206.0956012. F. P. Schiestl et al., The chemistry of sexual deception in an orchid-wasppollination system. Science 302, 437 (2003). doi:10.1126/science.1087835Medline3. J. Stökl et al., A deceptive pollination system targeting drosophilids througholfactory mimicry of yeast. Curr. Biol. 20, 1846 (2010).doi:10.1016/j.cub.2010.09.033 Medline4. H. E. M. Dobson, in Biology of Floral Scent, N. Dudareva, E. Pichersky, Eds.(CRC Press, Boca Raton, 2006), pp. 147–198.5. J. A. Riffell et al., Behavioral consequences of innate preferences and olfactorylearning in hawkmoth-flower interactions. Proc. Natl. Acad. Sci. U.S.A. 105,3404 (2008). doi:10.1073/pnas.0709811105 Medline6. J. T. Knudsen, B. B. Klitgaard, Floral scent and pollination in Browneopsisdisepala (Leguminosae: Caesalpiniodeae) in western Equador. Brittonia 50,174 (1998). doi:10.2307/28078487. R. Menzel, in Experimental Behavioral Ecology, B. Holldobler, M. Lindauer,Eds. (Gustav Fischer Verlag, Stuttgart, 1985), vol. 1, pp. 55–74.8. R. A. Raguso, C. Henzel, S. L. Buchmann, G. P. Nabhan, Trumpet flowers ofthe Sonoran Desert: floral biology of Peniocereus cacti and Sacred Datura.Int. J. Plant Sci. 164, 877 (2003). doi:10.1086/3785399. J. A. Riffell, H. Lei, T. A. Christensen, J. G. Hildebrand, Characterization andcoding of behaviorally significant odor mixtures. Curr. Biol. 19, 335 (2009).doi:10.1016/j.cub.2009.01.041 Medline10. A. M. Dacks, J. A. Riffell, J. P. Martin, S. L. Gage, A. J. Nighorn, Olfactorymodulation by dopamine in the context of aversive learning. J. Neurophysiol.108, 539 (2012). doi:10.1152/jn.00159.2012 Medline11. R. A. Raguso, O. Pellmyr, Dynamic headspace analysis of floral volatiles: acomparison of methods. Oikos 81, 238 (1998). doi:10.2307/354704512. N. Dudareva et al., Developmental regulation of methyl benzoate biosynthesisand emission in snapdragon flowers. Plant Cell 12, 949 (2000). Medline13. G. A. Wright, F. P. Schiestl, The evolution of floral scent: the influence ofolfactory learning by insect pollinators on the honest signalling of floralrewards. Funct. Ecol. 23, 841 (2009). doi:10.1111/j.1365-2435.2009.01627.x14. R. Alarcon, G. Davidowitz, J. L. Bronstein, Nectar usage in a southernArizona hawkmoth community. Ecol. Entomol. 33, 503 (2008).doi:10.1111/j.1365-2311.2008.00996.x15. K. C. Daly, T. A. Christensen, H. Lei, B. H. Smith, J. G. Hildebrand, Learningmodulates the ensemble representations for odors in primary olfactorynetworks. Proc. Natl. Acad. Sci. U.S.A. 101, 10476 (2004).doi:10.1073/pnas.0401902101 Medline16. A. Arenas, M. Giurfa, W. M. Farina, J. C. Sandoz, Early olfactory experiencemodifies neural activity in the antennal lobe of a social insect at the adultstage. Eur. J. Neurosci. 30, 1498 (2009). doi:10.1111/j.14609568.2009.06940.x Medline17. D. Yu, A. Ponomarev, R. L. Davis, Altered representation of the spatial codefor odors after olfactory classical conditioning; memory trace formation bysynaptic recruitment. Neuron 42, 437 (2004). doi:10.1016/S08966273(04)00217-X Medline18. T. Farooqui, K. Robinson, H. Vaessin, B. H. Smith, Modulation of earlyolfactory processing by an octopaminergic reinforcement pathway in the/ http://www.sciencemag.org/content/early/recent / 6 December 2012 / Page 2/ 10.1126/science.1225483
honeybee. J. Neurosci. 23, 5370 (2003). Medline19. S. Cassenaer, G. Laurent, Conditional modulation of ng.Nature482,47(2012).doi:10.1038/nature10776 Medline20. M. Hammer, R. Menzel, Learning and memory in the honeybee. J. Neurosci.15, 1617 (1995). Medline21. S. E. McGuire, M. Deshazer, R. L. Davis, Thirty years of olfactory learningand memory research in Drosophila melanogaster. Prog. Neurobiol. 76, 328(2005). doi:10.1016/j.pneurobio.2005.09.003 Medline22. A. M. Dacks, J. B. Dacks, T. A. Christensen, A. J. Nighorn, The cloning ofone putative octopamine receptor and two putative serotonin receptors fromthe tobacco hawkmoth, Manduca sexta. Insect Biochem. Molec. Biol. 36, 741(2006). doi:10.1016/j.ibmb.2006.07.002 Medline23. R. A. Bell, F. G. Joachim, Techniques for rearing laboratory colonies oftobacco hornworms and pink ballworms. Ann. Entomol. Soc. 69, 365 (1976).24. C. E. Reisenman, J. A. Riffell, E. A. Bernays, J. G. Hildebrand, Antagonisticeffects of floral scent in an insect-plant interaction. Proc. Biol. Sci. 277, 2371(2010). doi:10.1098/rspb.2010.0163 Medline25. C. A. Kearns, D. W. Inouye, Techniques for Pollination Biologists (Univ.Press of Colorado, Boulder, CO, USA, 1993).26. H. Lei, J. A. Riffell, S. L. Gage, J. G. Hildebrand, Contrast enhancement ofstimulus intermittency in a primary olfactory network and its behavioralsignificance. J. Biol. 8, 21 (2009). doi:10.1186/jbiol120 Medline27. T. A. Christensen, V. M. Pawlowski, H. Lei, J. G. Hildebrand, Multi-unitrecordings reveal context-dependent modulation of synchrony in odor-specificneural ensembles. Nat. Neurosci. 3, 927 (2000). doi:10.1038/78840 Medline28. H. Lei, T. A. Christensen, J. G. Hildebrand, Spatial and temporal organizationof ensemble representations for different odor classes in the moth antennallobe. J. Neurosci. 24, 11108 (2004). doi:10.1523/JNEUROSCI.3677-04.2004Medline29. H. Lei, T. A. Christensen, J. G. Hildebrand, Local inhibition modulates odorevoked synchronization of glomerulus-specific output neurons. Nat. Neurosci.5, 557 (2002). doi:10.1038/nn0602-859 Medline30. C. E. Reisenman, T. A. Christensen, W. Francke, J. G. Hildebrand,Enantioselectivity of projection neurons innervating identified olfactoryglomeruli. J. Neurosci. 24, 2602 (2004). doi:10.1523/JNEUROSCI.519203.2004 Medline31. J. A. Riffell, H. Lei, J. G. Hildebrand, Neural correlates of behavior in themoth Manduca sexta in response to complex odors. Proc. Natl. Acad. Sci.U.S.A. 106, 19219 (2009). doi:10.1073/pnas.0910592106 Medline32. W. L. Roelofs, A. Comeau, A. Hill, G. Milicevic, Sex attractant of the codlingmoth: characterization with electroantennogram technique. Science 174, 297(1971). doi:10.1126/science.174.4006.297 Medline33. K. C. Daly, B. H. Smith, Associative olfactory learning in the moth Manducasexta. J. Exp. Biol. 203, 2025 (2000). Medline34. M. Giurfa, Cognitive neuroethology: dissecting non-elemental learning in i:10.1016/j.conb.2003.10.015 Medline35. R. Menzel, U. Muller, Learning and memory in honeybees: from behavior doi:10.1146/annurev.ne.19.030196.002115 Medline36. M. Hammer, R. Menzel, Multiple sites of associative odor learning asrevealed by local brain microinjections of octopamine in honeybees. Learn.Mem. 5, 146 (1998). MedlineAcknowledgments: The authors thank C. Reisenman, R. Alarcón, A. Dacks, G.Davidowitz and J. Bronstein for advice and assistance; C. Hedgcock and F.van Breugal for images of flowers and moths; and M. Dickinson and H.Bradshaw for manuscript comments. This work was supported by NationalScience Foundation grants IOS 0822709 to JAR and CHE 0216226 to LA,and National Institutes of Health grant R01-DC-02751 to JGH.Published online 6 December 2012;10.1126/science.1225483Supplementary ce.1225483/DC1Materials and MethodsFigs. S1 to S7Tables S1 to S5References (23–36)01 June 2012; accepted 09 November 2012/ http://www.sciencemag.org/content/early/recent / 6 December 2012 / Page 3/ 10.1126/science.1225483
Fig. 1. Floral scent composition and similarity. (A) Total ionmass chromatograms of the scent from flowers visited by M.sexta (left) and species that have more generalizedassociations (right). Bioactive constituents are benzaldehyde(a), benzyl alcohol (b), methyl benzoate (c), and linalool (d).(B) The flowers analyzed in (A). (C) Principal componentsanalysis (PCA) plot based on the composition of the floralscent identified by GCMS from 19 flower species. Flowerspecies are abbreviated according to species name (tableS1). Dotted red oval indicates those flowers with aromaticdominant scents that are not significantly different from oneanother (ANOVA: P 0.01)./ http://www.sciencemag.org/content/early/recent / 6 December 2012 / Page 4/ 10.1126/science.1225483
Fig. 2. Antennal-lobe neuronal response to floral scents. (A)Intracellular morphology and recordings of PN responses toscents from hawkmoth-visited flowers. AL, antennal lobe; AN,antennal nerve. (B) PN responses to scents from flowers notvisited by M. sexta (top) and hawkmoth-visited flowers(bottom). (C) Confocal-microscopic image of AL showing thelocations of the recording sites of the multi-channel arrayused to record the neural ensemble responses. (D)Ensemble response to floral scents. (E) Principalcomponents analysis (PCA) of the firing-rate responses ofthe ensemble of neurons to the different floral scents. Thered oval indicates not significantly different responses tohawkmoth-visited floral scents (Kruskal-Wallis test: P 0.10).(F) Correlation coefficients between ensemble responses tothe different flower scents./ http://www.sciencemag.org/content/early/recent / 6 December 2012 / Page 5/ 10.1126/science.1225483
Fig. 3. (A) Image of a M. sexta moth attempting to feed fromone in an array of 20 artificial flowers used to test theattractiveness of different floral scents. (B) Feedingresponses by lab-reared M. sexta to the different floralscents. (C) PCA plot of the behavioral responses (table S3)to the different scents. Dashed red oval indicates thosehawkmoth-visited floral scents that were not significantlydifferent (P 0.05). (D) Pollen species found on theproboscises of wild M. sexta. (E,F) Wild M. sexta feedingresponses (E) and PCA plot of behavioral responses (F) tothe different floral scents./ http://www.sciencemag.org/content/early/recent / 6 December 2012 / Page 6/ 10.1126/science.1225483
Fig. 4. Olfactory learning modifies the neural representationof the scent in the AL. (A) Olfactory learning during classicalforward-paired conditioning experiments with A. palmeriscent and sucrose reward (orange triangles). Moths did notlearn the association in the absence of the unconditionedstimulus (US) (white diamonds), or the random paired CS-UStreatment (grey circles). (B) Response of an AL neuron tostimulation with A. palmeri scent at trial 1 (yellow) and trial 4(orange). (C) PCA plot of the ensemble responses to the A.palmeri scent through training. (D) Change in Euclideandistance of the ensemble representation of the A. palmeriscent during training. (E) Change in firing rate of AL neuronsduring trials 1, 4 (diagonal bars), and 8 (hashed bars) formoths in the different conditioning treatments: forward-paired(orange bars), no-US reward (white bars), and randompaired (grey bars). (F) In individual moths, focalmicroinjection of receptor antagonists (DA, OA), and agonist(OA) were conducted prior to conditioning. (G) Superfusion ofoctopamine significantly increased the firing rate of PNresponses. Letters denote a significant difference betweentreatments (P 0.05)./ http://www.sciencemag.org/content/early/recent / 6 December 2012 / Page 7/ 10.1126/science.1225483
Kruskal-Wallis test: P 0.69), but were significantly different from en-semble responses to the VOCs of flow-ers visited by other pollinator taxa (Fig. 2, B to D; Kruskal-Wallis test: P 0.001). Further recordings in the periphery and AL showed that oxygen-ated aromatics elicited the strongest responses and that the moth-visited