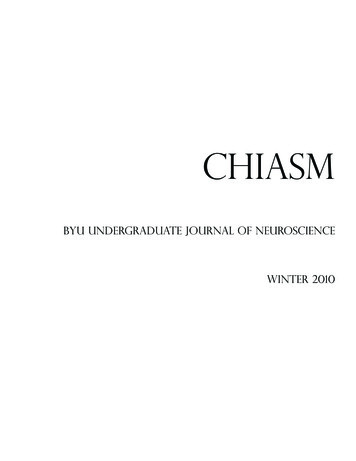
Transcription
ChiasmBYU Undergraduate Journal of Neurosciencewinter 2010
Neuroscience Student Association MembershipChiasm staffEditor in ChiefJD WilcoxCopy EditorsCaitlin NicholsDebbie HendersenKayla QuinneyManaging EditorAndrew HartshornAssociate EditorsJeff ClarkSierra DebenhamSpencer DyerBrian HoytJacob JonesCierra KeithTyler OwensStephanie SandovalTyler SlaterBrad StringfellowFaculty AdvisorsScott SteffensenDawson HedgesThe Neuroscience Student Association invites all neuroscience studentsand other interested persons to join our academically sponsored club.A NSA membership includes: A neuroscience t-shirt Discounted admission to Food for Thought (monthly dinner and research discussion with a neuroscience faculty member) Service opportunities like Friday’s Kids and Brain Awareness Week Connections with neuroscience students and faculty Internship and scholarship information A subscription to the sYnapse neuroscience newsletter Access to neuroscience social activitiesOne of the NSA’s notable accomplishments this year was its involvementin Brain Awareness Week (March 15–19), when over 51 NSA volunteersvisited and taught 29 high school, junior high school, and elementary schoolclasses at 11 different schools.Students may join the NSA in the Neuroscience Department Office (1055SWKT). Dues are 10 for the year. The NSA can be reached at byuneuroclub@gmail.com.Guidelines for SubmissionChiasm is always looking for quality publications from undergraduate students. If you’d like your work to be considered for our next issue, pleaseobserve the following guidelines for your submission: Work should be related to the field of neuroscience Articles should observe APA writing style Work may be collaborations or individual projects If chosen, your work will be reviewed by our editors, who will helpyou polish it for this and other publicationsAcknowledgmentsWe’d like to thank our faculty reviewers Dawson Hedges, Scott Steffensen,Michael Larson, and Michael Brown. We also want to thank Micah Hansen,the Neuroscience Center, and all others involved in any way.2Chiasm Winter 2010
ContentsContentsLetter from the editor 5The cognitive neuroscience of memory: An interview with Dr. Brock Kirwan 6The role of connexin-36 gap junctions in alcohol intoxication and reward 8Biological and adoptive mother-infant relationships in laboratory-reared rhesus monkeys (Macaca mulatta) across the first six months of life: The effects of adoptive mothers on infants’cortisol and ACTH levels 12Homing abilities in Dendrobates pumilio poison dart frogs 19Research ethics involving schizophrenic participants and informed consent 23The misdiagnosis of attention-deficit/hyperactivity disorder and corrective recommendations 28Office of Research and Creative Activities (ORCA) research awards to neuroscience students 37Volume 23
Letter from the editorLetter from the editorFriends,We have successfully finished the second volume of Chiasm! Thank you to all those who were involved in making thishappen again. We received even more submissions than last year and are excited to present the best of them to you.Chiasm is a student journal that is run and edited entirely by undergraduates. We created Chiasm so neuroscience students who are involved in research could receive recognition for their efforts and take their research to the next level. Ihope that each of you will take advantage of the opportunity to gain experience in the research, writing, and publishingprocess.You may remember an article published in Chiasm last year about detecting different botulinum toxin genes. I recentlyreceived the following email from the author Ben Satterfield and share it with his permission:Dear Editor,Last spring I had an article published in Chiasm entitled “A Quadraplexed real-time PCR assayfor rapid detection and differentiation of the Clostridium botulinum toxin genes A, B, E, and Fin pure culture and environmental samples.” An updated version of that article was publishedthis month in the Journal of Medical Microbiology with me as first author. I am very appreciative of the chance that Chiasm gave me to publish my article in a “trial run” and for the inputand edits from your team. I hope that other students take the opportunity to use Chiasm as aplatform to publish their works.This is an excellent example of how Chiasm can help students take their work to the next level, and I hope you will takeBen’s advice.We are privileged to be at an undergraduate institution with many opportunities to get involved in research. The mentored research program at BYU offers undergraduate students opportunities that would be restricted to graduate students at other universities. If you are not currently involved in research, make it a goal to get involved. This involvementwill help propel you to the next level of education and will enhance your experience here at BYU. If you are currentlyinvolved in research, make it a goal to submit an article to Chiasm before you graduate. This is truly a unique opportunity that is only found at a few select universities across the nation.I sincerely hope that you enjoy this volume of Chiasm.Best,JD WilcoxEditor in ChiefVolume 25
The cognitive neuroscience of memory: An interview withDr. Brock KirwanInterview conducted by Andrew Hartshorn & Stephanie SandovalDr. Brock Kirwan is the newest member of the BYU Neuroscience Faculty. He earned his PhD in Psychological and BrainSciences from Johns Hopkins University in 2006 and completed a postdoctoral fellowship at UCSD last year.What is the main focus of your research?My research focuses on long-term declarative memory. I’m interested in multiple aspects of memory and I use a number of different methodologies to get at my research questions, includingfMRI, neuropsychological testing with amnesic patients, EEG,and behavioral testing with healthy younger adults (undergrads)and older adults.What are the goals or objectives of your research?We’ve known for a long time (since the initial description of Patient H.M. in the 50’s) that the medial temporal lobe is responsiblefor long-term declarative memory. However, there are still big theoretical debates about the exact functions of the medial temporallobe, and specifically of the hippocampus. The goal of my researchis to shed some light on the question of what the hippocampusdoes and hopefully help to resolve some of the big debates.I’m particularly interested in what determines what we will remember and what we’ll forget. Our memories are pretty good forevents that stand out (a clown walking into your Neuroanatomycourse would be remembered) but are really bad for things thatare ordinary (we are always forgetting where we parked at themall). Why is that? What is the brain (i.e. the hippocampus) doing differently in one situation vs. the other?What type of people or students do you have working in you lab?Excellent ones (and I’m not just saying that because I knowsome of my students are on the Chiasm editorial staff). Rightnow, I have one graduate student from the Psychology Department and about half a dozen undergraduates who all happen tobe neuroscience majors. Since the primary duty of undergraduateRAs in my lab is to run subjects, it’s a requirement that you havegood people skills. Beyond that, it’s important that students havean interest in cognitive neuroscience and be excited about whatthey’re doing and learning.6What are some of the major discoveries that you have made?One exciting result that we’ve had was when we were able toshow that within the hippocampus, different sub-regions responddifferently when you’re learning new or overlapping information.We used high-resolution fMRI to scan subjects while they vieweda series of everyday objects. We didn’t ask the subjects to learnthe objects, but we’ve shown that subjects learn them incidentally anyway. Unbeknownst to the subjects some of the objectswere repeated, and some were followed by very similar (but notidentical) lure objects. We showed that the response to the lureobjects was different in different regions of the hippocampus, indicating that some regions treated them just like the repeats (anddidn’t “see” the change) while some regions treated them just likebrand-new objects. This is a useful thing if you need to remembersimilar or overlapping information, like where you parked yourcar today instead of where you parked your car yesterday.What are some of the difficulties you face working with yourtest subjects?I don’t usually work with particularly troublesome populations, so I can’t really complain. The biggest challenge in doingmy research so far has been that BYU doesn’t (yet) have an MRIscanner. I’ve been working on setting up scanning at the U of U,and that should be up and running soon. I’m really looking forward to when we do have a scanner on campus and we can involve students more directly in the research.What initially got you interested in this type of research?I got into research as an undergraduate working in a rat lab thatstudied very similar problems of learning and memory. I came tolove the process of generating knowledge through experimentation. I was lucky in that I happened to land in a lab that was researching some very interesting questions that have continued tohold my interest for years since.Chiasm Winter 2010
The cognitive neuroscience of memory: An interview with Dr. Brock KirwanDo you have a favorite vacation spot?I have fond memories of my grandma’s cabin near PalisadesReservoir in Idaho. We lived in San Diego for a few years and I’dhappily visit there any time. Disneyland would probably top thelist, though.Who has been the biggest influence in your life?Beyond family, my biggest influences in my professional lifeare my undergraduate advisor, Ray Kesner, and my graduateand postdoc advisors, Craig Stark and Larry Squire. All three arescary-smart and passionate about what they do.Dr. Brock Kirwan (top row, third from right) with members ofhis research lab.What advice would you give to a new neuroscience student?You’ve picked an excellent major. Don’t shy away from the larger theoretical questions. Too often we lose sight of the bigger picture questions (like “How does the mind work?”) in favor of ourmore proximal questions (like “how does this protein work?”).Both are important questions, and may be getting at the samething from different levels of analysis. It’s important to be able towork at different levels of analysis and make those connections.How do you see the future of neuroscience?Cognitive neuroscience, I think, is going to become more sophisticated in how we use the tools that we have available to us.So much information is lost (or thrown away) in a typical fMRIexperiment. Most fMRI studies do group analyses and you lose alot of individual variability when you work with group-level data.I think that we’ll see more emphasis on these individual differences.Tell us a bit about your family.We have two kids (ages 9 and 6) and one on the way in July.My wife and I were introduced in high school (she’s from Arizona; I’m from Idaho) and we started dating in college. We’ve beenmarried for 11 years. After living in sunny San Diego for 3 yearswhile doing my postdoc, we had some reservations about movingto the cold of Provo, but we’ve loved it so far.What is your favorite book?I’m a big sci-fi/fantasy geek. I’ve probably read Ender’s Gameby Orson Scott Card the most times (I’m currently reading it tomy son—with a liberal amount of editing out the bad words onmy part). The Lord of the Rings also ranks high on my list of favorite books.Volume 2What is the best way for interested undergrads to get involvedin your research?Talk to me. If you’re interested we can usually find some wayfor you to help out in the lab.Any additional things you would like to say about your research?I’m very excited to be part of the neuroscience program atBYU. I think that the program is really going in a great directionand I think that my research fits in nicely with the other strengthsof the program.Tell us about you experience as a volunteer assistant to theHungarian national Olympic committee at the 2002 WinterOlympics.This is one of those unusual things that I get to put on my CV.I was assigned to be a translator and assistant to the coaches andadministrators for the Hungarian delegation. I got to pick up folksfrom the airport, drive them to different venues and functions,and generally hang out in the team’s office in the Olympic village.I got to interact with the athletes and got a number of perks (tickets to some events, including the Closing Ceremonies). It wasalso a great way to talk about the Church when I went to gradschool interviews, which happened a few weeks after the Olympics. Since I was coming from Salt Lake City, everyone asked ifI went to the Olympics, so I got to talk about being an assistantto the Hungarian NOC and how I happen to know Hungarian,which in turn lead to a discussion about the Church.So far, how do you like teaching here as opposed to at SDSUor Johns Hopkins? What are the biggest differences for you or ingeneral?My experience so far at BYU has been great. I’ve been consistently impressed with students here. I’ve found that studentseverywhere are overextended in their time commitments, andthe difference between students at different universities is in howthey are overextended. Since I taught evening classes at SDSU,many of my students were trying to balance full-time jobs withfinishing school. Here, the focus is different, but students seemjust as busy as anywhere else.7
The role of connexin-36 gap junctions in alcohol intoxicationand rewardRyan S. McClellan, Katie D. Bradley, David W. Allison, D. Micah Hansen, Jeffery D. Wilcox, MandyFoote, Brian Hoyt, Jordan T. Yorgason, and Scott C. SteffensenThe midbrain Ventral Tegmental Area (VTA) is connected to reward and pleasure mechanisms associated with intoxication and addiction. The VTA uses dopamine (DA), making DA a molecule of interest in investigating addiction. GABA is theprimary inhibitory neurotransmitter in the brain and inhibits the dopaminergic neurons of the VTA. The VTA GABA neuronshave been shown to synchronize with one another through gap junctions, including the connexin-36 (Cx36) gap junction(Lassen et al., 2007). Further, alcohol affects the Cx36 junction, an action possibly associated with some of the intoxicating effects of alcohol (Stobbs et al., 2004; Allison et al., 2006). We hypothesized that Cx36 gap junctions regulate the VTAGABA neurons by synchronizing neuron excitability and firing, thereby regulating DA levels. Through the results of theOpen Field Assay for Motor Activity, Fast Scan Cyclic Voltammetry, and subsequent analysis of the data collected, we observed the effects of alcohol on Cx36 knockout (KO) mice, wildtype (WT) mice, and WT mice injected with mefloquine, aCx36-selective antagonist. The KO mice drank significantly less alcohol than did WT mice and had increased ataxic effectsdue to the alcohol. However, the KO mice performed better than the WT mice in coordination tests at lower doses of ethanol. Fast Scan Cyclic Voltammetry tests performed on all mice showed that DA levels in KO mice took longer to return tobaseline levels, suggesting that DA active transport (DAT) function may be altered in KO mice. Our findings show that Cx36alters VTA GABA inhibition of DA and is involved in the mechanism for alcohol intoxication and reward.IntroductionThe Ventral Tegmental Area (VTA) of the midbrain is involved in addiction and reward. Part of the mesocorticolimbic diffuse modulatory system, the VTA is associated with theperception of pleasure through dopamine (DA) and the brain’sprimary inhibitory neurotransmitter, GABA. Further, moderately intoxicating doses of ethanol reduce VTA GABA neuron firingrates and synaptic responses (Gallegos et al., 1999; Allison et al.,2006). GABA released from VTA GABA neurons inhibits VTAdopaminergic neurons. When VTA GABA neurons are inhibited,DA levels increase, leading to intoxication and impaired function(Di Chiara & Imperato, 1985; Steffensen et al., 1998). Generallyspeaking, higher levels of neurotransmitters in the synapses ofthe brain cause a stronger stimulation on the presynaptic neuron.Stronger stimulations lead to greater intracellular and neuronalresponses, which in turn strengthen the connections between theneurons and make future stimulations easier. Therefore, higherlevels of DA in the VTA strengthen synapses and ease neuronalstimulation, causing neurons to need increasing amounts of dopamine to function; this dopamine resistance leads to addiction.8Gap junctions are made up of two connexons, which are eachformed by six connexin proteins. When these proteins join toform gap junctions between adjacent neurons, the ions andchemicals necessary for nerve function can pass between thecells. These pathways couple the cells electrically and chemicallyand can serve to synchronize the excitability and firing of the neurons (Roerig and Feller, 2000; Rozental, 2000; Bennett, 2002).Gap junction blockers, including the connexin-36-selective antagonist mefloquine, suppress the excitability of the VTA GABAneurons. This evidence suggests that coupling of these neuronsthrough gap junctions controls the excitability of dopamine neurons and the level to which the mouse exhibits intoxicating andsedative effects of ethanol (EtOH). Therefore, this study aims todetermine if the Cx36 gap junction causes synchrony in the VTAGABA neurons and to determine the role of this gap junction inreward and intoxication due to alcohol. Furthermore, we hypothesized that the KO mice would be less inhibited by the sedativeeffects of EtOH due to a lack of the coupling effect provided bythe Cx36 gap junction.Chiasm Winter 2010
The role of connexin-36 gap junctions in alcohol intoxication and rewardMethodsAnimalsMale C57BL/6J mice were bred and cared for in accordancewith the National Institutes of Health Guide for the Care andUse of Laboratory Animals. Once weaned at PND 25, they werehoused in maximum groups of four and given ad libitum access towater and solid food and placed on a reverse light/dark cycle withthe lights on from 8pm to 8am. Mice were then housed separatelyafter all EtOH injections and other drug testing to accommodateproper measurement of EtOH self-administration. All mice, except those that were evaluated for EtOH self-administration inthe drink-in-the-dark procedure, were given ad libitum access tosolid food and water.Self-AdministrationWe implemented a drink-in-the-dark method in order to determine if male C57BL/6J mice, the WT mice, would self-administer EtOH (Rhodes et al., 2005). Three hours after the beginning of the dark cycle, the normal water bottles of both the Cx36KO and WT mice were replaced with a sipper tube filled with20% EtOH. The mice were then allowed to drink for nine days,two hours every day. Alcohol consumption was measured witha graduated pipette sipper tube, which recorded the EtOH levelbefore and after the two-hour period (Gupta et al., 2008). Thesemethods were repeated for five days on WT mice injected withmefloquine and vehicle as a control for the KO and WT mice. Tocontrol for any physical characteristic that might have affected amouse’s ability to drink and to evaluate any significant differencesbetween KO and WT mice (i.e., performance deficits), we gavethe mice water bottles filled with 10% sucrose for five days, twohours every day (Gupta et al., 2008). Any mice that were significantly low performers for sucrose drinking (i.e., that were morethan two standard deviations away from the mean) were not included in the EtOH self-administration test.Behavioral AssessmentOpen Field Assay for Motor Activity:For both WT and KO mice we measured the gross motor activity and ataxia (the loss of overall motor coordination—a common effect of intoxicating doses of alcohol) in one of four openfield chambers, each equipped with a piezoelectric transducer cemented to the underside of the suspended floor. The signal fromthe piezoelectric transducer was amplified 10x and filtered from0.1–100 Hz (-3dB). We evaluated locomotor activity in Cx36 KOmice and WT littermates for 25 minutes after an injection of varying doses of acute EtOH (0.75, 1.5, 2.5, and 4.0 g/kg), pentobarbital (25 mg/kg), MK-801 (0.5 mg/kg), and Ritalin (20 mg/kg).Volume 2The root mean squared voltage (Vrms) indicates the amount oflocomotor activity (Fisher & Itkin, 1995). These methods wererepeated on WT mice injected with mefloquine and vehicle as acontrol for the KO and WT mice.Fast Scan Cyclic Voltammetry:Fast Scan Cyclic Voltammetry (FSCV) measures the oxidization and reduction of dopamine in response to an applied voltageramp run through a carbon fiber electrode. Specific voltage rangesoxidize and reduce dopamine (Garris et al., 2004). The change incurrent when DA is oxidized and reduced is proportional to theconcentration of DA. Voltammetry shows the relative changes inDA release and uptake in control versuss treated mice.Drugs and InjectionsFor non-contingent EtOH experiments, mice were injected intraperitoneally with 16% EtOH under brief isoflurane anesthesia.Doses of EtOH (0.75, 1.5, 2.5, and 4.0 g/kg) were administeredevery other day on a randomized schedule. For contingent EtOHexperiments, during self-administration drinking tests, mice weregiven 20% EtOH ad libitum from a sipper tube. After all EtOH injections and drinking experiments, mice were injected intraperitoneally with 25mg/kg pentobarbital, a GABA agonist, and thensubjected to behavioral tests. At least two days after pentobarbitalinjections, mice were injected intraperitoneally with 0.5 mg/kgMK-801, an NMDA antagonist. At least two days after MK-801injections, mice were injected intraperitoneally with 20 mg/kgRitalin, a dopamine active transport (DAT) inhibitor. Testingwas delayed 15 minutes to compensate for the delay in onset ofthe effects of Ritalin.Statistical AnalysisBehavioral measures (motor activity in the open field assayand EtOH drinking) were analyzed in Cx36 KO and WT controls. For repeated measures in the same subjects (e.g., EtOHdose response in motor tests), a mixed models ANOVA withTukey post hoc test was performed using the Statistical Analysis System (SAS). For all other behavioral comparisons, a SingleFactor ANOVA was performed using Microsoft Excel. DA releasevia FSCV was analyzed using Single Factor ANOVA in Excel. Expression of DAT in WT versus KO mice was compared using anunpaired, two-tailed t-test with Graph Pad software. IGOR ProSoftware was used in the creation of figures.ResultsCx36 KO mice drank significantly less EtOH than the WTcontrol mice (p 0.0001, KO 1.5–2.5 g/kg/day, WT 3–5 g/kg/day), suggesting that KO mice are more sensitive to the seda9
The role of connexin-36 gap junctions in alcohol intoxication and rewardFigure 1: Differences in self-administration of EtOH andsucrose in KO vs. WT mice and mefloquine- vs. vehicle-treatedmice. (A) KO mice consumed significantly less EtOH than WTmice. (B) There was no significant difference in sucrose consumption between KO and WT mice. (C) Mefloquine-treatedmice also consumed significantly less EtOH than vehicletreated mice. (D) Mefloquine-treated mice, however, consumesignificantly less sucrose. (Significance between WT and KOmice, *: P 0.05, **: P 0.01).tive effects of EtOH (see Figure 1). The KO mice were still capable of drinking normal amounts of liquid as shown by the amountof sucrose that was self-administered, but they drank significantlyless alcohol. Further, mefloquine-treated mice drank significantlyless EtOH than the vehicle-treated mice (P 0.05, mefloquinetreated 2–3.5 g/kg/day, vehicle-treated 3.5–5 g/kg/day), suggesting that mefloquine has a similar effect on mice as did the genetic process of knocking out the connexin-36 gap junction. Bothmice treated with mefloquine and Cx36 KO mice demonstrate aheightened sensitivity to the sedative effects of EtOH.In tests done to measure gross motor control and ataxia, allKO mice performed worse than the WT mice did, further showing the higher sensitivity of KO mice to the effects of EtOH (seeFigure 2: Effects of acute intoxicating doses of EtOH on motoractivity in (A) KO vs. WT mice and (B) in mefloquine-treatedvs. vehicle-treated mice. EtOH-induced ataxia was significantlygreater in KO mice than in WT mice but not in mefloquinetreated vs. vehicle-treated mice.10Figure 2). Typically, ataxia (evidenced by stumbling and highlyuncoordinated movements) increases with increasing doses ofEtOH. However, when the same low dose of alcohol was given toboth KO and WT mice, the KO mice showed much more ataxia,or decreased motor control, demonstrating that they have a greater sensitivity to the effects of EtOH.When we used Fast Scan Cyclic Voltammetry (FSCV) to further test the differences between KO mice, WT mice, and WTmice injected with mefloquine, we found that the DA signal in theKO mice was significantly lower than that in both the WT control mice and the WT mice injected with mefloquin (see Figure3). The amount of DA in the mefloquine-injected WT mice washigher than that in the normal WT mice. The upward slope of thepeak was also much lower, indicating a possible change in the roleof dopamine active transport (DAT) in KO mice. The recoveryperiod for both KO mice and mefloquine-treated WT mice wasmuch longer than for WT mice; this finding suggests that DATFigure 3: Disruptions in dopamine transmission in KOmice and WT mice treated with mefloquine. Inset left showsDA oxidation (to dopamine-o quinone) and reduction produced by a voltage ramp applied to the carbon fiber electrode.Dopamine is oxidized and reduced at specific voltage ranges.The change in current when DA is released is proportional tothe concentration of DA. Inset at top-right shows the currentresponse produced by the rising and falling phases of the voltage ramp (voltammogram). The inset graph at middle-rightshows the DA current produced in the nucleus accumbens coreby stimulation of the medial forebrain bundle (MFB), and theinset image plot at bottom-right shows the time course of thecyclic voltammograms around MFB stimulation. The graphcompares the averaged MFB-NAcc DA signal (calibrated to astandard DA current) from the NAcc in WT vs KO mice andin WT mice after intraperitoneal administration of 30 mg/kgmefloquine.Chiasm Winter 2010
The role of connexin-36 gap junctions in alcohol intoxication and rewardmay affect the manner in which neurons recycle DA and repackage it for future use.DiscussionThe results of our study suggest two main findings. First, KOmice were more sensitive to EtOH. Second, DAT seemed to havean altered function in the KO mice, providing another potentialmechanism for intoxication. Our original hypothesis was that theKO mice would be less inhibited by the sedative effects of EtOHbecause these mice lack the coupling effect that connexin-36 hason VTA GABA neurons. Our results suggested otherwise. TheKO mice were actually more sensitive to the sedative effects of theEtOH, as shown by the results of the motor tests and the EtOHself-administration test. Without the coupling and synchronizingeffects of Cx36 gap junctions, the VTA GABA neurons may havehad reduced capacity to inhibit VTA DA neurons; this deficiencymay have prevented them from effectively managing DA levels,thereby contributing to the heightened sensitivity of Cx36 KOmice to EtOH. Studies have shown that when a rat’s VTA or nucleus accumbens (Nacc) is stimulated when the rat pokes its nose ina hole, the animal will continue this behavior—disregarding everything else—in order to receive the continued stimulus to its pleasure system (Gatto et al., 1994). However, the inability of GABAneurons to control DA neurons in KO mice increased the sensitivity of these mice to the ataxic effects of alcohol by lowering levels ofDA; this finding suggests that KO mice drank less EtOH becausethey were so intoxicated that they could not drink anymore. Thedata also support the idea that intoxication limited the amount ofEtOH consumed by KO mice because the KO mice generally performed worse on the motor tests. The FSCV tests demonstrated aslower uptake in DA for the KO mice, indicating that DAT has altered function. We are unsure exactly why DAT does not functionnormally in the KO mice, but its altered function is another possible explanation for the increased sensitivity to EtOH in KO mice.DAT removes DA from the synapse, shortening its synaptic effect.When DAT function is slightly lower than normal, as seen in theFSCV recordings on KO mice, the DA stays in the synapse longer.This may be causing the increased intoxication seen in KO mice.Further research of these neurons and their effects on the mesocorticolimbic system could help determine how the mechanismsof pleasure and reward work in addiction. One focus of furtherresearch could be to examine the effects of DAT on mice in conjunction with their sensitivity to EtOH and other drugs that causeintoxication in animals. Animal models may be used in this fieldfor some time until suitable studies approved for human use canbe found. Once such studies are undertaken, researchers will gaina better understanding of how drugs change the natural chemicaland physiological signals inside the body, giving us the opportunityto help rid the world of the growing problem of addiction throughthe same means that addiction is created.Volume 2ReferencesAllison DW, Ohran AJ, Stobbs SH, Mameli M, Valenzuela CF,Sudweeks SN, Ray AP, Henriksen SH and Steffensen SC(2006) Connexin-36 gap junctions mediate electrical coupling between ventral tegmental area GABA neurons. Synapse 60:20-31.Bennet MV (2002) Neoreticularism and neuronal polarization.Prog. Brain Res 136:189-201.Di Chiara G and Imperato A (1985) Ethanol preferentially stimulates dopamine release in the nucleus accumbems of freelymoving rats. Eur. J. Pharma
Dr. Brock Kirwan is the newest member of the BYU Neuroscience Faculty. He earned his PhD in Psychological and Brain Sciences from Johns Hopkins University in 2006 and completed a postdoctoral fellowship at UCSD last year. 7 The cognitive neuroscience of memory: An interview with Dr. Brock Kirwan .