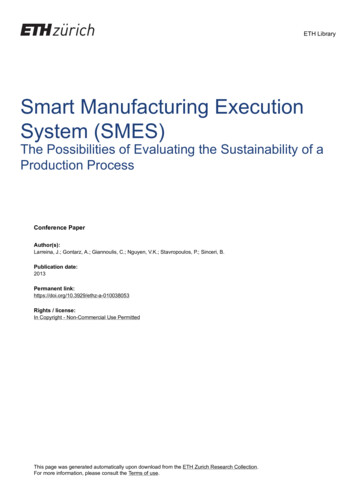
Transcription
ETH LibrarySmart Manufacturing ExecutionSystem (SMES)The Possibilities of Evaluating the Sustainability of aProduction ProcessConference PaperAuthor(s):Larreina, J.; Gontarz, A.; Giannoulis, C.; Nguyen, V.K.; Stavropoulos, P.; Sinceri, B.Publication date:2013Permanent s / license:In Copyright - Non-Commercial Use PermittedThis page was generated automatically upon download from the ETH Zurich Research Collection.For more information, please consult the Terms of use.
15.3 Smart Manufacturing Execution System (SMES):The Possibilities of Evaluating the Sustainability of a Production Process12343J. Larreina , A. Gontarz , C. Giannoulis , V.K. Nguyen , P. Stavropoulos , B. Sinceri125Industrial Management and Innovation Unit, IK4-Tekniker, SpainInstitute of Machine Tools and Manufacturing (IWF), Swiss Federal Institute of Technology, Switzerland3Laboratory for Manufacturing Systems and Automation (LMS), University of Patras, Greece45CADCAMation SA, SwitzerlandProcess Research Department, Centro Ricerche Fiat, ItalyAbstractSustainability in production processes is mandatory in the manufacturing environment due to restrictions suchas legislation. Conventional Manufacturing Execution Systems (MES) don’t really support environmentalsustainable goals but are actually the most suitable background for an extension towards sustainabilitymonitoring, control and assessment. This paper introduces a new MES generation which is enhanced withinteroperable data acquisition, analysis and optimization in line with sustainability goals.A harmonization work on metrics and indicators, aligned with the Triple Bottom Line, is presented based on ananalysis of industrial users’ requirements within machining process context. Also, a framework forsustainability evaluation through a specific architecture is introduced.Based on an industrial use case, the given approach is set in the context of the FoFdation project which alsoaddresses the integration among manufacturing IT systems towards overall lifecycle management.Keywords:Data acquisition, Green manufacturing, Manufacturing Execution System (MES), Sustainability evaluation,Triple Bottom Line1 INTRODUCTIONSustainability in production processes is mandatory in themanufacturing environment due to constraints such aslegislation, market and company goals. The market factorexplains why there is a growing need for better monitoringand awareness of manufacturing processes to address bothenvironmental and productivity optimization issues. Qualityimprovement, production cycle reduction must nowadays bemanaged while also taking into account new concurrentthresholds such as energy consumption and carbon footprintindicators.Conventional Manufacturing Execution Systems (MES) don’treally support environmental sustainable goals but areactually the most suitable background for an extensiontowards sustainability monitoring, control and assessment.This paper introduces a new generation of MES which isenhanced with interoperable data acquisition, analysis andoptimization in line with sustainability goals.A harmonization and clarification work on metrics andindicators, aligned with the Triple Bottom Line, is presentedbased on the analysis of industrial users’ requirements andliterature research within machining process context.Furthermore, a framework that suggests how to implementsustainability evaluation through a specific architecture andthe required metrics description is introduced.The given approach, based on an industrial use case, is set inthe context of the FoFdation project (www.fofdationproject.eu) which also addresses the integration with PLMERP towards overall lifecycle management.The paper introduces an analysis and proposal for asustainability evaluation framework in which the IT solution isbased. A comprehensive set of existing sustainability metricsand indicators have been considered and categorizedaccording to different aspects such as the Triple Bottom Linedefinition. Out of such metrics and indicators, the frameworkwas chosen to foster the implementation of sustainabilityimprovements and support decision makers in assessing theimpact and effects of these measures not only on theenvironmental requirements but also on the overall businessperformance.After a review on existing commercial systems (dedicateddata collection tools, MES like tools and specialized systemsfor energy management), the Smart Manufacturing ExecutionSystem (SMES) solution is presented. The conceptual ideafor such a solution was based on a conventional MES,adapted and enhanced with new hardware architecture inorder to directly collect information on resource consumptionfrom the machines. The proposed architecture allows for thecombining of information on the execution of themanufacturing operations and resource consumption data,leading to an improved awareness of the manufacturingoperations performance. The data collection features arecompleted with an analysis through an Online AnalyticalProcessing (OLAP) system which allows the investigation ofsustainability parameters in a multidimensional space, andthen the optimization of the production by a scheduler thatschedules production orders according to an energy efficiencystrategy.The resulting prototype was set up as a demonstrator in theCentro Ricerche Fiat (CRF) labs for validation purposes ofSMES data collection features and details of this validationare given below.As a conclusion, the difficulties which were encounteredduring the implementation and installation are stated and thethThe 11 Global Conference on Sustainable Manufacturing517
challenges for the future mentioned. The results obtainedallow to explore new concepts for online and automaticoptimization towards a sustainable manufacturing process.22.1SUSTAINABILITY EVALUATION FRAMEWORKMetrics and indicatorsSustainable manufacturing and related energy efficiency arebecoming an important topic in industry. Still, in the case ofenergy efficiency, neither machine tool users nor buildershave a clear picture of their energy use in production [1, 2].This fact is due to individual evaluation approaches which aredependent on the field of industry, motivation, and individualmanufacturing processes and needs; the Ford ProductSustainability Index (PSI) [3], for instance, defines a set ofindicators based on the ISO 14040 (Life Cycle Assessment LCA). Those indicators are selected from the point of view ofthe automotive industry. A common approach on a higheraggregated level is given by the Global Reporting Initiative(GRI) [4]; as this method is globally recognized and issupposed to be generally valid, but it is also controversiallydiscussed [5]. Another large scale approach is given by theSustainable Value [6]; it evaluates the resource allocation andits effects in money units for economical, ecological andsocial resources in line with the Triple Bottom Line definition.These examples illustrate various selections of metrics andindicators for the evaluation of sustainability with theirdependencies, individual needs and information and levels ofapplication, e.g. LCA or organizational levels, as indicated by[7-9]. Based on this application variety a selection tool wasdeveloped by the FoFdation project where more than 120indicators and metrics were grouped together according tothree aspects in order to support users’ selections: Life CycleAssessment status, application level within the factory and theTriple Bottom Line definition. This grouping has led to keyperformance indicators (KPIs), Environmental PerformanceIndicators (EnPIs) and Measurement Values (MVs) (Figure 1)and enables various users to set their metrics.in manufacturing, Bunse et al. [11] concludes that variousenergy efficiency performance measures already exist in theaggregated sector, e.g. plant, but these performancemeasures are not necessarily suitable to assess energyefficiency performance of single manufacturing processes orthe machine tool. Furthermore, appropriate energy efficiencymetrics and measurement frameworks on machine, processand plant level, and energy efficiency benchmarks onmachines and equipment for monitoring and optimization aremissing. Emerging new sensor technologies and smartembedded devices enable operation based processmeasurements and therefore can provide accurateinformation for monitoring the production performance,Karnouskos et al. [12].In the FoFdation project a framework was chosen that is ableto integrate energy efficiency measures in an adequateperformance assessment system for manufacturingcompanies on the shopfloor level. This approach can fosterthe implementation of energy efficiency improvements andsupport decision makers in assessing the impact and effectsof energy efficiency measures not only on the environmentalrequirements but also on the overall business performance.Figure 2: Elements of Sustainable Evaluation Framework inthe FoFdation project based on Bunse et al. [11]3SMART MANUFACTURING EXECUTION SYSTEMThe SMES aims for leveraging an existing MES to reduce theeconomical and environmental impact in manufacturingthrough reducing cycle times, rework, materials, energy,emissions, wastes and scraps.3.1Figure 1: Clustering of metrics and indicators within theFoFdation projectAs the strategic level mainly focuses on awareness andcontrol and is dependent on the CRF use case, special focusfor metrics and indicators was given on the shopfloor formachine tool energy evaluation according to ISO14955 [10].2.2Sustainable performance measurementAs the assessment of the sustainable performance in the fieldof energy efficiency in production has become a major assetState of play in data collection systemsSustainability evaluation must lie on a proper IT system fordata collection. A review on commercial solutions revealsthree main kinds of systems devoted to such a purpose:dedicated data collection tools, MES like tools, andspecialized systems for energy management.Most dedicated data collection tools focus only on datamonitoring and availability. Their objective is to deliver theinformation, store it in a base, and visualize it through tables,graphs and reports, e.g. Predator MDC , RF-SMART,Scytec Hosted DataXchange , IntegraNet , Acumen’sAutomated Shop Data Collection SW, Catalyst PDC ,Q*ADC, etc. They typically use standard database and/orspreadsheet formats for storage and they collaborate withMES and ERP systems for delivering the requested data.518
Higher level MES like tools focus mainly on theproduct/assets information collection such as product trackinginformation, BOM, assets utilization, equipment states,resource availability, etc., e.g. EZ-MES. Some of these toolsgo beyond production data collection and reporting as theyprovide some extra modules/functions that supportsemiautomatic scheduling and ’what if‘ scenarios, e.g.FACTIVITY, Litum, Acumen’s Job Tracking SW.Specialized systems that focus on sustainability issues arealso available from several manufacturers. Most of thesystems focus mainly on energy management, although theymay also support other sustainability metrics (e.g. SchneiderElectric, Siemens WinCC, Rockwell Automation EEM, GEEnergy Management).As a consequence of the analysis performed, an existingconventional Manufacturing Execution System (MES) hasbeen selected as a basis for the adopted solution within theFoFdation project context. Although not fully compliant uringExecution Systems are recognized as the most appropriatesolutions towards a comprehensive approach for sustainablemonitoring, controlling and assessment [13].The selected MES has been adapted and improved with anew system (FoF-EMon) that enables the data collection andmonitoring of environmental resource consumption on themachine tool. The combination of the MES and FoF-EMonresults in a package that relates information on the executionof the manufacturing operations to environmental resourceconsumption data. Such a combination leads to an improvedawareness of the manufacturing operations performancethrough a configurable set of sustainability KPIs.3.2ArchitectureIn the figure below, the architecture for the conceptualsolution of SMES is shown.ERPSMESERP ConnectorFoFͲEMonͲMES adapterMESB2MMLHTTP POSTspecific modules to provide higher connectivity with othersystems, i.e. the ERP connector to integrate with the ERP,and an OPC UA client to give the SMES the capability toconnect to any system that supports this de factocommunication technology standard. An adapter relates theMES to FoF-EMon which collects data directly from themachine tool components with internal and external sensorsand combines simulations too to save costs on the appliedsensors. FoF-EMon monitors the consumption of the differentparts of the machine tool and provides categorizedinformation for the shopfloor.Regarding analysis and optimization, the architecture includesa feature for Online Analytical Processing (OLAP) whichallows the consolidation and analysis of sustainability datacollected on the shopfloor in a multidimensional space, basedon the SMES database, and a scheduler to scheduleproduction orders according to an energy efficiency strategy.Based on operations research and artificial intelligence, itapplies a newly developed dispatching rule, Less EnergyConsumption (LEC), that allows production schedules to beobtained that lead the enterprise to operate on the lowestenergy consumption possible.3.3Environmental sustainability data collectionA key element for energy efficiency evaluation andoptimization is seen in the accurate quantification of theenergetic behavior on the shopfloor, machine tool level [8,14, 15] and its components. For this reason a data acquisitiontool for Energy Monitoring (FoF-EMon) on the machine tool aspart of the Smart Manufacturing Execution System (SMES)was developed within the FoFdation project. FoF-EMon isunderstood as a crosslink between machine control and theManufacturing Execution System (MES). Based on ISO14955[10], FoF-EMon collects all relevant energetic information,including electrical components and compressed air andmedia flow from external sensors and from the machine toolcontrol and simulations for a detailed resource evaluation.With a sampling rate of 5Hz all energetic relevant information,including peak power, are collected, synchronized anddisplayed (Figure 4). This data is then processed, analyzedand transmitted with a constant rate of 1Hz to the MES.FoFͲEMonOPCͲUA ClientWeb servicesData collectionMessage BrokerOLAP DriverOLAPAnalysis and optimizationWeb servicesSchedulerFigure 3: SMES ArchitectureThe architecture includes a centralized integration point(Message Broker) which coordinates the information flowamong all modules within the SMES. It consists of aninteroperable platform that operates through Web Servicesand SOAP protocol and utilizes a message driven mechanismfor information exchange. Key elements in the architectureare those related to data collection and analysis andoptimization. As part of data collection, the existingconventional MES is devoted to collect manufacturingoperations execution data from the shopfloor. It incorporatesFigure 4: Example of data provided by FoF-EMon3.4Production execution data collectionFor the collection of production execution data an MESsystem has been adapted. The MES is able to collectessential data (work order, actual times and quantities,machine failures and times incurred, traceability data, qualitydata, etc.) both automatically and manually through an HMIon industrial PCs on the shopfloor, and incorporates datacoming from the machine through the FoF-EMon. The FoFEMon-MES adapter provides information on resource519
consumption (energy, compressed air) from the FoF-EMon.This information is aggregated and stored in the SMESdatabase for subsequent analysis. The consumptioninformation is stored by time, piece, event/machine status, butalso in relation to work order, part number, material(traceability) and process (how it was machined). Thisaggregation facilitates the analysis that relates energyconsumption data with production data which is thenpresented through an OLAP based dashboard that showsrelevant KPIs with their target and actual values.Nevertheless, the MES itself also has the ability to analyzethe performance of the production site in terms ofsustainability through sustainability metrics and PIs. The MESincludes a dashboard where the operator and line responsiblestaff can check the status of sustainability performance in acertain machine. It contains information on the productionorder, its status, metrics and PIs to assess the performance:processing times, setup times, downtimes; producedquantities (good, scrap, and their causes); energyconsumption (categorized by compressed air, machineconditioning, machining, process cooling and conditioning,waste handling, tool handling); and several indicators such asOEE, availability, performance and quality or the energyconsumption ratio (relationship between energy used formachining compared to the energy for auxiliary systems),which gives an idea of the effectiveness of the machine tooland the efficiency of the energy used.In order to collect data on the shopfloor, information from theERP related to the scheduled work orders has to be obtainedby the MES. It also needs to feed back the ERP withinformation of actual production data collected on theshopfloor. Such information is exchanged through adeveloped ERP connector based on Business ToManufacturing Markup Language (B2MML) schemas, theXML implementation of the ANSI/ISA-95 family of standards,known internationally as IEC/ISO 62264. In addition to this,the MES includes a work order sequencer to enable it tochange the production schedule downloaded from the ERP orfrom the scheduler within the SMES, due to last minutechanges (emergencies, lack of resources or material, etc.).The MES also includes an HMI for production supervision.The corresponding HMI shows the lines and machines undercontrol and gives quick information on the status of themachines, but also detailed information on sustainabilityperformance for each machine on the shopfloor.3.5Analysis and optimizationThe collection of data for further analysis at a later point intime requires the use of a repository with a structure tosupport a significant amount of data per machine tool.Implementation of this approach to a larger production scalethat will lead to the big data problem is not considered in thispaper. In this approach, the collected data are applied to theidentified sustainability metrics and act alongside decisionsupport systems. Moreover, the resulting values can befurther visualized in dashboard applications or by usingcommercial spreadsheet applications to perform personalizedanalysis. The metrics values are accessible by other systemssuch as the IMPACT scheduler to optimize the plan of thenext period based on the energy consumption of the lastproduction period.sustainability at different levels; at machine level (microoptimization) with FoF-EMon and at production line level witha scheduler (macro-optimization).Online Analytical Processing (OLAP)The amount of data is quite significant and restricts theselection of the storage system. Online Analytical Processing(OLAP) tools are the foundation of data warehouses [16, 17]supporting analysis of enormous amounts of data in rationalresponse times. In this paper we considered the RelationalOLAP (ROLAP) variation as production performances arestored in a traditional Relational Database ManagementSystem (RDBMS). Furthermore, the lowest level of productioninformation is considered to be the collected information fromthe machine tools. OLAP tools structure information in cubesconsisting of dimensions, measures and facts. The cube isorganized as follows. There are 8 dimensions: (D1) machinetool extends to the hierarchical levels [18], (D2) part, (D3)operation, (D4) work orders, (D5) operators, (D6) time (D7),machine components and (D8) machine status, and 4 facts:(F1) cycles of machine tools including yield quantities andprocessing times, (F2) energy consumption of each machinefunction, (F3) production demand and (F4) machine statuses.Table 1 indicates the number of measures implemented ineach fact table. Additionally, 22 calculated measures ofaggregate raw data i.e. Overall Equipment Efficiency (OEE),Machine Utilization and Machine Efficiency.Table 1: Facts and Measures#FactF1Machine CyclesMeasures4F2Machine Energy11F3Machine Status5F4Machine Power4F5Production Demand4This implementation gives us the opportunity to correlatemeasures such as availability of machines with Mean Time toRepair (MTTR) as shown in Figure 5 more efficiently, orcorrelate the energy consumption of a factory with thedemand volume or the Quality Ratio of facilities. Moreover,the potential to explore the correlation possibilities is left tothe end user by selecting the appropriate measures forvisualization from dashboard however, the dashboard itself isnot included in the current paper.Figure 5: Availability as a Function of Mean Time To Repair[18]This chapter shows the SMES approach for analysis of thecollected data through OLAP and for the optimization of520
FoF-EMonFor micro optimization, standby monitoring was introduced inthe FoFdation project. As the energy used in machine standbycan raise up to 43% of the total energy consumed [19], it isessential to address the non value added standby energyconsumption which is independent from quality and safetyrelated issues. According to the monitored data and analysison FoF-EMon certain components can be switched off inconformity with predefined rules. In the given case the coolingcompressor indicated in Figure 3 can be switched off after 15seconds when the machine is in standby without any negativeeffects on productivity or quality in the given manufacturingprocess. Further optimization on the micro-optimization levelis seen in the predictive service and maintenance bydetecting inefficient components according to Gontarz et al.[20].The presented level of detail in Figure 3 from FoF-EMon canbe used not only for micro-optimization, e.g. active machinetool and machine components’ switch off [21], but for macrooptimization as well. In combination with higher aggregatedinformation, such as from the MES, optimized productionscheduling can be performed.SchedulerProduction scheduling has been addressed by manyresearchers over previous decades. Typical schedulingaddresses the problem of allocating jobs and tasks to anumber of machines. Energy Aware Scheduling aims tooptimize either an existing schedule [22] or to follow certainmethodology [23] to obtain the minimum possible energyconsumption. In this paper, we consider the IMPACTscheduler [18]; a multicriteria scheduler based on MADEMAmethod [24, 25, 26], as part of the macro-optimizationstrategy. IMPACT assigns tasks to resources by typicaldispatching rules i.e. Shortest Processing Time (SPT),Earliest Due Date (EDD) etc. However, it can produce aschedule based on a multicriteria method with various factorssuch as cost, quality, flowtime etc. The model of the proposedscheduler has been extended to include the operating andidle power on each machine tool of the production shopfloor.In addition, the energy consumption criterion has beenintegrated in order to evaluate the energy consumptionperformance of the schedule. Moreover, we introduce a LessEnergy Consumption (LEC) dispatching rule to assign tasksto machine tools based on their estimated energyconsumption. Energy aware scheduling minimizes the energyusage and enhances its efficiency.4VALIDATIONIn a case study, the FoFdation-SMES prototype includingFoF-EMon was implemented in the CRF labs on a 5 AxisMilling machine tool. The implementation included thecollection of ERP information, machine status and the fulldetails of power consumption of the machine toolcomponents, e.g. pumps, fans, and motors. By knowing theenergetic- and control behavior of each machine toolcomponent in combination with production execution andERP information, quality- and safety-irrelevant componentscan be controlled by this system. In this context, a coolingcompressor switch off message was successfully generatedto reduce the power consumption during standby by 12 kW.Together with a reduced air supply, (4,8 kW) an automatedreduction of up to 50% on the total machine tool powerconsumption during standby is possible with no negativeeffects on productivity or quality. This implementation is seenas a data acquisition and control tool for all relevantsustainable information related to resource consumption onthe machine tool, and proves that the system is able to reactto and control certain components to not only monitor butactively increase the environmental performance on theshopfloor with related effects on higher aggregated levels aswell.The validation process did not consider the analysis andoptimization implementations which will be tackled in asecond phase. The case will be extended to severalmachines in order to deal with the macro-optimizationapproach.5CONCLUSIONSThe presented work is based on a demo approach that hasbeen built in a pragmatic way to clearly highlight a verypractical solution as a proof of concept to address an industryuse case where the main requirements are the manufacturingplanning process optimization, and the efficiency of theenergy consumption in production processes. For this astraightforward approach to achieving sustainability goals hasbeen developed through leveraging an existing MES’sfunctionality to manage raw materials and resources, such asenergy. The usage of MES systems for sustainabilityenhancement opens the door to improve and obtainincreased gains in resource optimization.Therefore the adopted solution is ideal for improving resourceutilization – not only in terms of using less material but also byproviding better information on how those resources shouldbe used. The MES has been enhanced with resourceconsumption data collection based on a versatile and easy toinstall system called FoF-EMon, which includes a dashboardto monitor energy consumption. These results are running asa prototype with both the adapted MES system and the FoFEMon, and their link. This data collection infrastructure isused by the SMES to optimize the usage of resourcesthrough the analysis optimization modules.The main difficulties encountered in the implementation of thedata collection system have been the fact that the machineused is a nonstandard machine tool with multiple retrofits andno up-to-date specifications. A future challenge for the FoFEMon application is a streamlined and market ready setupprocedure for individual implementation. Currently thisapplication is seen as a prototype solution which stronglydepends on the goal system architecture. Other importantissues are affordable sensors, stability and reliability on boththe hardware and software side, improved dashboard andfurther development in the awareness tool according to theISO14955 and/or others. For the production execution datacollection side, represented by the MES, the main challengesare an easier and faster adaptation to customer functionalrequirements and the usage of its OPC UA interface in afuture factory environment where this technology will bewidely spread and, therefore, allows for seamless andinteroperable resource consumption data collection from anyequipment on the shopfloor.These results allow us to explore new concepts for online andautomatic optimization towards a sustainable manufacturingprocess, taking into consideration all manufacturing factors.521
J. Larreina, A. Gontarz, C. Giannoulis, V.K. Nguyen, P. Stavropoulos, B. SinceriFor further progression, the macro-optimization approach willconsider not only energy consumption but also other aspectsof sustainability according to the Triple Bottom Line,especially, social ones. Also, the SMES validation will beextended to a scenario with multiple machines.[13]Rockwell Automation, 2009, Manufacturing ExecutionSystem for Sustainability. Extending the scope of MESto achieve Energy Efficiency and Sustainability Goals.[14]Avram, O., Xirouchakis, P., 2010, Evaluating the usephase energy requirements of a machine tool system.6Journal of Cleaner Production, 19/2011:699-711.ACKNOWLEDGMENTSThe work reported in this paper was partially supported byCEC / FP7 NMP-ICT Programme, ’The Foundation for theSmart Factory of the Future-FoFdation‘, (FP7-2010-NMP-ICTFoF- 260137).[15]Gontarz, A., Weiss, L., Wegener, K., 2010, onaMultichannelmachinetool,inInternational Conference on Innovative TechnologiesIN-TECH Proceedings of the International Conference7[1]on Innovative Technologies, Editor: Prague, CzechREFERENCESRepublic. 499-502.Dietmair, A., Verl, A., 2009, Energy ConsumptionForecasting And Optimisation For Tool Machines. MM[16]Science Journal, 3:63-67.[2]with Applications, 38/4:3743-3756.Peroni, M., Polignano, V., 2012, CRF Use Case Internal document of the FoFdation project WP1.2 and[17]in data warehouse and OLAP systems. Data &Schmidt, W.-P., 2006, Life Cycle Tools within Ford ofKnowledge Engineering, 70/8:732-752.Europe's Product Sustainability Index. Case Study FordS-MAX & Ford Galaxy (8 pp). The International ting[19][20][21]Gutowski, T., et al., 2005, Environmentally benign[22]manufacturing: Observations from Japan, Europe 'Driscoll, E., O'Donnell, G.E., 2013, Industrial powerand energy metering – a state-of-the-art review. tion systems. CIRP Annals - ManufacturingMelnyk, S.A., Stewart, D.M., Swink, M., 2004, MetricsmeasurementWeinert,Methodology for planning and operating energy-efficientof Cleaner Producti
15.3 Smart Manufacturing Execution System (SMES): The Possibilities of Evaluating the Sustainability of a Production Process J. Larreina 1, A. Gontarz 2, C. Giannoulis 3, V.K. Nguyen 4, P. Stavropoulos 3, B. Sinceri 5 1 Industrial Management and Innovation Unit, IK4-Tekniker, Spain 2 Institute of Machine Tools and Manufacturing (IWF), Swiss Federal Institute of Technology, Switzerland