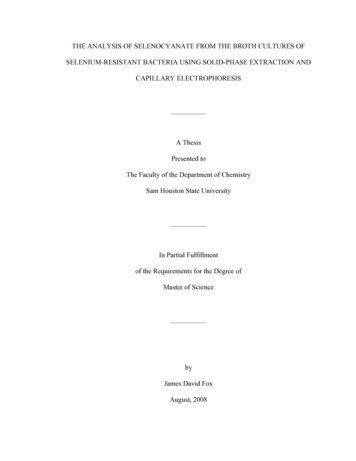
Transcription
THE ANALYSIS OF SELENOCYANATE FROM THE BROTH CULTURES OFSELENIUM-RESISTANT BACTERIA USING SOLID-PHASE EXTRACTION ANDCAPILLARY ELECTROPHORESISA ThesisPresented toThe Faculty of the Department of ChemistrySam Houston State UniversityIn Partial Fulfillmentof the Requirements for the Degree ofMaster of SciencebyJames David FoxAugust, 2008
THE ANALYSIS OF SELENOCYANATE FROM THE BROTH CULTURES OFSELENIUM-RESISTANT BACTERIA USING SOLID-PHASE EXTRACTION ANDCAPILLARY ELECTROPHORESISbyJames David FoxAPPROVED:Dr. Thomas ChasteenThesis DirectorDr. Darren L. WilliamsDr. Chi Chung YuApprovedDr. Jaimie Herbert, DeanCollege of Arts and Science
ABSTRACTFox, James David, The analysis of selenocyanate from the broth cultures of seleniumresistant bacteria using solid-phase extraction and capillary electrophoresis. Master ofScience (Chemistry), August, 2008, Sam Houston State University, Huntsville, Texas.The purpose of this research was, in part, to develop a method for the preconcentration and extraction of selenocyanate from the liquid cultures of seleniumresistant bacteria using solid-phase extraction and capillary electrophoresis withphotodiode array detection. An environmental soil bacterium (130404) growing on acomplex medium displayed an inherent resistance to various forms of selenium and hadthe ability to convert them into elemental and insoluble selenium and intoorganoselenides. Experiments using ion chromatography-inductively coupled plasmamass spectrometry indicated that this bacterium was also able to convert small quantitiesof selenate into selenocyanate.Experiments also examined the effect of various selenium-containing species onthe growth of 130404 and E. coli clone (1VH) by examining the optical density of theculture over time. Similar experiments were done with a Bacillus spp. to determine suchfactors as nitrate dependence and ampicillin resistance.The headspace of liquid cultures of 1VH and the Bacillus was also examinedusing solid-phase microextraction and gas chromatography with fluorine-induced sulfurchemiluminescence detection. Both organosulfides and organoselenides were observedin the headspace of selenium-amended cultures, indicating their ability to bioprocess theselenium-containing species.iii
Keywords: solid-phase extraction, capillary electrophoresis, selenocyanate, selenateApproved:Dr. Thomas G. ChasteenThesis Directoriv
ACKNOWLEDGEMENTSThe journey I have made thus far has been both wonderful and arduous; however,I would have never made it alone. I would like to thank my mother Cathy, my fatherDavid and my sister Chelsey. Their love and encouragement have carried me through thehighs and lows, and they have always supported my endeavors. I would like to thank mygrandmother Charline. Her weekly phone calls provided encouragement, love and thehumor I needed to shrug off the fatigue in times of stress. I would like to thank mymentor Dr. Thomas Chasteen; he has continued to provide a wealth of knowledgeconcerning chemistry, culture, music and film. Finally, I would like to thank myacademic family at the CFS. The late nights at Stardust, the music, the never-endingstream of clever repartee and pranks are what make the days fly by.v
TABLE OF CONTENTSPageABSTRACT. iiiACKNOWLEDGEMENTS.vTABLE OF CONTENTS .viLIST OF TABLES .viiiLIST OF FIGURES.ixCHAPTER I: INTRODUCTION.1Analytical Techniques for Detecting Selenium Compounds.4Gas Chromatography with Fluorine-Induced Sulfur Chemiluminescence Detection.4Capillary Electrophoresis (CE) .5Solid-Phase Extraction (SPE) .7Microorganisms Used.8Relevance of This Research.8CHAPTER II: EXPERIMENTAL .10Bacterial Growth .10Headspace Sampling .11CE Samples .12IC-ICP-MS Analysis .14Solid-Phase Extraction .15CHAPTER III: DATA AND RESULTS.18Bacterial Growth Curves .18Headspace Analysis.26vi
IC-ICP-MS.35Solid Phase Extraction and Capillary Electrophoresis .35CHAPTER IV: DISCUSSION .43Bacterial Growth Curves .43Headspace Analysis.44IC-ICP-MS.46Solid-Phase Extraction and Capillary Electrophoresis.47CHAPTER V: CONCLUSIONS AND FUTURE WORK.51LITERATURE CITED.53APPENDIX: Chemical Abstract Service Registry Numbers .56VITA.57vii
LIST OF TABLESTablePage1 Comparison of the specific growth rates in selenium-amendments for organisms grownin LB medium.232 Comparison of the specific growth rates for Bacillus spp.253 Summary of the IC-ICP-MS results. .35viii
LIST OF FIGURESFigurePage1 The extraction apparatus.162 The extraction process. .173 Growth data for E. coli 1VH in LB medium. .194 Growth data for E. coli 1VH in LB medium with 10.0 mM selenite.195 Growth data for E. coli 1VH in LB medium with 10.0 mM selenate. .206 Growth data for E. coli 1VH in LB medium with 10.0 mM selenocyanate. .207 Growth data for 130404 in LB medium. .218 Growth data for 130404 in LB medium with 1.0 mM selenite.219 Growth data for 130404 in LB medium with 1.0 mM selenate. .2210 Growth data for 130404 in LB medium with 1.0 mM selenocyanate. .2211 Growth data for Bacillus spp. in TSN3. .2312 Growth data for Bacillus spp. in TSN1. .2413 Growth data for Bacillus spp. in TSB.2414 Growth data for Bacillus spp. in TSN3 with ampicillin. .2515 The headspace of E. coli 1VH in LB medium. .2716 The headspace of E. coli 1VH in LB medium with 1.0 mM selenite. .2817 The headspace of E. coli 1VH in LB medium with 1.0 mM selenate.2918 The headspace of E. coli 1VH in LB medium with 1.0 mM selenocyanate.3019 The headspace of Bacillus spp. in TSN3 medium.3120 The headspace of Bacillus spp. in TSN3 medium with 1.0 mM selenite.3221 The headspace of Bacillus spp. in TSN3 medium with 1.0 mM selenate. .33ix
22 The headspace of Bacillus spp. in TSN3 medium with 1.0 mM selenocyanate. .3423 1.0 mM selenocyanate sample in CE.3624 SPE using methanol and water solvation mixture.3725 SPE using acetonitrile and water solvation mixture.3726 Sample with selenocyanate and sodium chloride.3827 Sample with 1.0 mM selenate and selenocyanate, yeast extract and digest of casein. 3928 Sample with 1.0 mM selenocyanate in LB medium eluted with water and methanol. 3929 Sample with 1.0 mM selenocyanate eluted with sodium chloride in buffer.4030 130404 in LB medium with 1.0 mM selenate at 3 hours.4131 130404 in LB medium with 1.0 mM selenate at 7 hours.4132 130404 in LB medium with 1.0 mM selenate at 10 hours.42x
1CHAPTER IINTRODUCTIONFor the most part, the role of selenium in our world is subtle. At 0.05 ppm, it isthe sixty-sixth most abundant element in the earth’s crust (Greenwood and Earnshaw,1997), and is most commonly found in the environment in the water-soluble oxyanionsselenite (SeO32-) and selenate (SeO42-). Despite its relatively small abundance, seleniumplays an important biological role in most, if not all animals in the form of the aminoacids selenocysteine and selenomethionine, as well as the proteins formatedehydrogenase and thioredoxin reductase. In fact, people living in areas of lowenvironmental selenium concentration have been observed to fall ill with diseases relatedto selenium deficiency in substantial numbers (Chasteen and Bentley, 2006; Chasteen andBentley, 2002). This biological role of selenium also extends to plants andmicroorganisms. Though rare, several strains of bacteria require unusually largeconcentrations of selenium (236-7896 mM) in order to survive (Lindblow-Kull et al.,1982).Despite its biological role, the toxic and carcinogenic effects of selenium havebeen studied since 1842 (Moxon et al., 1943; Nelson et al., 1943). More recently, it hascome to the attention of scientists that the accumulation of selenium does not stop withremediating plants, but continues up the food chain into human diets as well. Extensivestudies have been carried out to examine the accumulation of selenium in biologicalsystems (Dumont et al., 2006).Research in this area has been fueled largely by concerns over the ecologicalrepercussions of regions with a naturally high selenium concentration such as the
2Kesterson National Wildlife Reserve in the San Joaquin Valley in California. It isthought that the contamination here originates from Cretaceous marine sedimentary rock.Via weathering and irrigation drainage, water-soluble forms of selenium eventually findtheir way to hydrologically-closed basins. In 1983, this accumulation of selenium wasfound to be responsible for the 64% deformity and death rate of embryos and hatchingsof aquatic birds in the region. So profound was the phenomena that it was eventuallydubbed “The Kesterson Effect” (Presser, 1994).Unfortunately, selenium contamination is not limited to natural causes. Othermajor sources include copper and oil refinement (Greenwood and Earnshaw, 1997).While selenate and selenite are the two major selenium-containing species produced,selenocyanate (SeCN-) has also been of interest. Special attention was given to theremoval of selenocyanate from oil refinery wastewater via its deselenation andprecipitation with elemental iron (Meng et al., 2002). However, studies by Miekeley etal. (2005) suggest that selenocyanate is a natural component of oil, and therefore anothermethod may be desired.Much attention has been given to proposed methods for cleaning seleniumcontaminates from toxic sites. Some plants are capable of converting water-solubleselenium species into methylated volatile compounds. It was determined that such plantscould be instrumental in the cleanup of polluted sites (Meija et al., 2002; Kahakachchi etal., 2004). The construction of artificial wetlands has been shown to successfully reducethe overall concentration of water-soluble selenium species in wastewater from a coal gasstripping plant by 64-79%. This was accomplished by using plants native to the westernUnited States that have an innate ability to accumulate large amounts of selenium. Of the
3plants tested, several displayed no inhibition of growth when introduced to thewastewater (Ye et al., 2003). Such bioaccumulation of selenium has also been noted inseveral strains of yeast (Wrobel et al., 2005) as well as algae (Larsen et al., 2001).In addition to phytoremediation, bioremediation also presents an alternativecleanup method. Several microorganisms exhibit a resistance to water-soluble forms ofselenium and are able to bioprocess them via reduction and methylation. In these cases,the organism sometimes apparently uses selenate or selenite as the terminal electronacceptor in its metabolic cycle. Volatile methylated selenides have been observed in theheadspace of such anaerobic bacterial cultures (Chasteen et al., 1990). A mechanism forthe process that leads to the organo-metalloids found in bacterial headspace has beenproposed (Challenger, 1945; Chasteen, 1993). In addition to this, many microorganismsare able to reduce a substantial portion of the soluble selenium to which they’re exposedto Se0, which can be seen as a red precipitate in the liquid culture (Losi andFrankenberger, 1997) and is substantially less toxic than the initial selenium oxyanions.This process is affected in some organisms by the presence of nitrate as an electronacceptor (Oremland et al., 1999), thus lowering the overall yield of selenium conversion.Bioremediation has been used successfully in some cases using bacteria isolated fromrice-straw from the Broadview Water District in California (Zhang et al., 2003; Zahir etal., 2003).While resistant to selenium, some microorganisms exhibit selenium-dependentspecific growth rates (SGR). This allowed researchers to assess the relative toxicity ofvarious selenium species by examining the specific growth rate of bacterial cultures
4dosed with them (Strotmann et al., 1994; Yu et al., 1997), as well as selenium’s effect onpersister cells (Harrison et al., 2005).Analytical Techniques for Detecting Selenium CompoundsA number of instrumental techniques have been applied in the search for variousselenium species. X-ray absorption near-edge structure (XANES) spectroscopy has beensuccessfully implemented in the detection of various forms of selenium, includingelemental, aqueous and volatile forms (Pickering et al., 1995; Sarret et al., 2005). Ionchromatography–inductively coupled plasma–mass spectrometry (IC-ICP-MS) has alsobeen used in the detection of trace amounts of water soluble selenium species. Thismethod has the added benefit of low detection limits (0.04-0.09 ng) in the presence ofcomplex samples (Miekeley et al., 2004). Hydride generation-atomic absorptionspectroscopy (HG-AAS) was also used with positive results. In a study by Losi et al.(1997), the microbiological conversion of selenate to selenite and then to elementalselenium was observed.For this research, several different methods of selenium speciation were applied:Gas Chromatography with Fluorine-Induced Sulfur Chemiluminescence DetectionThere are a number of benefits in using gas chromatography with fluorineinduced sulfur chemiluminescence detection (GC/SCD) to look for volatile organo-sulfur,-selenium, and -tellurium compounds. Given their non-polar nature and relatively largedifferences in boiling points, volatile methylated selenides have been found to separateeasily on several different gas chromatographic columns (Basnayake et al., 2001; Meija
5et al., 2002; Hunter and Kuykendall, 2004; Swearingen, Jr. et al., 2006). In the SCD,gaseous, elemental fluorine is produced in situ by flowing sulfur hexafluoride across an1100 V volt discharge at approximately 26 kHz of alternating current. A substantialportion of the resulting gas phase fragments reform as F2 and continue into the reactionvessel. It is there that they react with methylated chalcogens to produce excited-statehydrogen fluoride, which, upon de-excitation, emits a photon that is detected via aphotomultiplier tube. The pressure in the reaction vessel is maintained at less that onetorr to ensure low collisional deactivation.Because of the intense reaction of methylated chalcogens with F2 compared toother molecules, the background signal for this instrument remains low enough and theselectivity high enough to obtain detection limits in the picogram range for this family ofcompounds (Chasteen, 1993).Capillary Electrophoresis (CE)Much work has been done in the task of separating and detecting various watersoluble selenium species using capillary electrophoresis. Some methods include couplingCE with inductively-coupled plasma-atomic emission spectroscopy (ICP-AES) (Deng etal., 2007), conductivity detection (Kubá et al., 2004) or simple ultraviolet/visibleabsorption spectroscopy (Walker et al., 1996). CE has provided an efficient means ofseparating and detecting these selenium species in solution. It is because of this that CEseems ideal for use with broth cultures of selenium-resistant bacteria. Recently, work byPathem et al. (2007) showed the time-dependant conversion of selenate to selenite andelemental selenium by a strain of Escherichia coli.
6The principles on which electrophoresis operates are simple, yet effective. Themethod’s capillary, similar to a capillary column in GC, is made of fused silica with apolyimide coating for thermal and electrical insulation. The inner diameters usuallyrange from 25-75 µm and the length is between 50-75 cm. Because of this, the samplevolume needed is typically 1-50 nL. This allows smaller overall sample sizes. ForUV/Vis detection, a small portion of the polyimide coating is removed so that light canpass through with minimal absorbance by the capillary itself. This is the capillarywindow.Before separation can occur, the capillary is filled with a buffer solution that actsto ionize the silanol groups along its interior. The more basic the buffer solution, themore ionization occurs thus raising the overall negative charge along the capillary walls.This buffer solution will also act as an electrolyte to carry the current during separation.Positively charged ions will coat the interior of the capillary in two layers: one that isaffixed to the wall and one that will move in the electric field. This second layer causes amass movement of molecules within the capillary, thus establishing the electro-osmoticflow (EOF). Typically the cathode is on the same end as the detector, causing positivelycharged molecules to move toward this electrode the fastest, followed by neutral and thennegatively charged molecules dragged along by the EOF.In these experiments, a photodiode array was used for detection, allowing for thesimultaneous monitoring of absorbance values between 190 and 300 nm. As themolecules pass the capillary window, their absorbance was measured as a function oftime and wavelength. This was useful for distinguishing between CE peaks whoseretention times are similar but differ in their absorbance profiles.
7In the case of the water-soluble selenium species, negatively charges ions are ofinterest. Therefore a modification to the EOF was necessary. To accomplish this, asurfactant (tetradecyltrimethylammonium bromide) was used to coat the interior of thecapillary, reversing its charge. Once this was done, the polarity of the electric field wasalso reversed. This caused anions to elute first, followed by neutral molecules and thencations.With broad linear ranges and low detection limits, CE is ideal for the monitoringof trace amounts of selenium species produced by selenium-resistant microorganisms.Solid-Phase Extraction (SPE)While CE provides low detection limits, it may be necessary to pre-concentratethe samples in order to better see them in the electropherogram at even lowerconcentrations or in the presence of interfering species. In order to accomplish this, SPEwas utilized to collect selenocyanate from broth cultures of selenium-resistant organisms.To this day, SPE is typically used as a cleanup method for complex biologicalsamples (Shimelis et al., 2007). However, this method was shown to be effective atretaining and recovering analytes when, among others, Monohan et al. (1995) used stronganion exchange (SAX) SPE in order to collect the herbicide Dacthal for analysis via GCwith electron capture detection.This research made use of aminopropyl-coated SPE cartridges. The pKa of theamine group on the cartridges was approximately 9.8. Solvation of the cartridgeprotonated the amine groups to generate a positive charge and ionic binding betweenthem and negatively charged components of the solvation buffer. Once solvated, a
8sample was passed through the cartridge, at which time negatively charged seleniumcontaining species attached to the amino groups and were retained on the cartridge’s solidphase. Then, a buffer (pH 11.8) was passed through, causing the deprotonation of theamino groups and the elution of the analytes. This allowed an amount of analyte to becollected and deposited into a smaller volume of liquid, effectively raising theconcentration.Microorganisms UsedFor this research, data from three different metalloid-resistant, anaerobicmicroorganisms were examined: 130404 (a species of the genus Alcaligenes), Bacillusspp. and Escherichia coli 1VH. The organism 130404 was provided by collaboratingresearchers at the University of Santiago, Chile. E. coli 1VH was prepared by Araya etal. (2004). The Bacillus spp. was isolated in the Chasteen research group at Sam HoustonState University and its genus determined in Chile. All organisms display some degreeof selenium resistance as measured by their ability to grow in millimolar solutions ofselenium oxyanions.Relevance of This ResearchMuch instrumentation has already been successfully applied to examine theconversion of water-soluble selenium into other forms. However, many of these methodshave looked solely at the conversion to elemental or volatile selenium. Interesting workexamining the intermediate biological steps as selenium-containing oxyanions arebioprocessed to less oxidized forms has been reported (Walker et al., 1996; Pathem et al.,
92007). This may be important in understanding the final destination of selenium as it isprocessed by these resistant organisms.IC-ICP-MS experiments by Gürleyük et al. (2006) indicated that one possiblebiological product that had been overlooked was selenocyanate. Until this point, theoccurrence of selenocyanate in contaminated areas was attributed to one of two things:the reduction of other selenium species via environmental chemistry or the presence ofoil waste. Locating a microbiological source of selenocyanate may prove useful to thoseinterested in the removal of selenium from areas of high concentration.For the work in this thesis, a method was developed to examine thebacteriological production of selenocyanate using solid-phase extraction and capillaryelectrophoresis. In addition to this, the relative toxicities of the various water-solubleselenium species and the production of volatile headspace gases was also determined.
10CHAPTER IIEXPERIMENTALBacterial GrowthReagentsThe chemical reagents used in these experiments were obtained from thefollowing sources: pancreatic digest of casein and yeast extract from Difco Laboratories(Detroit, MI, USA); tryptic soy broth from Mediatech, Inc. (Herndon, VA, USA); sodiumselenite, sodium selenate and sodium hydroxide from Sigma-Aldrich (Milwaukee, WI,USA); potassium selenocyanate from Acros Organics (New Jersey, USA); potassiumnitrate from EM Science (Gibbstown, NJ, USA); sodium chloride from BDH (WestChester, PA, USA); agar from Amresco (Solon, OH, USA). All water used in theexperiments was purified via a RiOs 3 water purification system from Millipore(Billerica, MA, USA).Bacterial Cultures and Growth MediaBacterial cultures of Escherichia coli 1VH and the Gram negative bacterium130404 were grown anaerobically in Lauria-Bertani (LB) medium at 37º C in a waterbath. The LB medium was prepared by adding 10.0 g of pancreatic digest of casein, 5.0g of yeast extract and 5.0 g of sodium chloride to 1.0 L of water and mixing thoroughly.If needed, the pH of the solution was adjusted to 7.0 with 1.0 M sodium hydroxide. Thismedia was then distributed into flasks and sterilized at 121º C and 15 psi for 20 min.Once cooled, the media was inoculated with 1VH from a stab culture using a sterile loopand allowed to incubate for 24 hours. Plate cultures were prepared by adding 20 g of
11agar to 1.0 L of LB medium and sterilized as above. Before cooling, the agar wasdistributed to Petri dishes and amended with 1.0 mM sodium selenite. These plates werestreaked using a sterile loop dipped in samples of the prepared liquid cultures andallowed to incubate for 24-36 hours (until single, red bacterial colonies could be seen).Precultures were prepared by inoculating LB medium with a single colony and allowed itto incubate for 24 hours. Experimental cultures were made by distributing sterile LBmedium into test tubes and inoculating with a 1:10 v/v inoculum from the preculture.Experimental cultures were amended with sodium selenate, sodium selenite or potassiumselenocyanate to yield a final concentration of 1.0 or 10.0 mM.The Bacillus spp. was grown anaerobically in tryptic soy medium with a 0.3%nitrate content (TSN3) at 30º C in a water bath. TNS3 was prepared by adding 10.0 g oftryptic soy broth (TSB) and 3.0 g of potassium nitrate to 1.0 L of water and mixedthoroughly. Plate cultures, precultures and experimental cultures were made with TSN3using the same steps as above.Headspace SamplingApparatusFor the headspace analysis, a Hewlett-Packard 5890 Series II gas chromatograph(GC) with a Sievers 300 fluorine-induced sulfur chemiluminescence detector (SCD)(Ionics Instruments, Boulder, CO, USA) was used. The GC was equipped with a DB-1column (100% polydimethylsiloxane, 5.0 µm stationary phase) that measured 30 m x0.32 mm i.d. (J&W Scientific, Folsom, CA, USA). Data acquisition was accomplishedusing a Hewlett-Packard 3396 Series III integrator. The headspace samples were
12collected using 75 µm Carboxen -polydimethylsiloxane (PDMS) solid-phasemicroextraction (SPME) fibers from Supelco (Bellfonte, PA, USA).GC ParametersHelium was used as a carrier gas at a flow rate of 1.0 mL per minute. All sampleswere injected in splitless mode into a 275º C injection port. The split valve opened at2 min. The initial oven temperature was held at 30º C for 2 min and then raised15º C/min until a final temperature of 275º C was reached. This temperature was held for5 min.SPME SamplingCultures (10 mL liquid) used for headspace samples were grown in 16 mL testtubes capped with open-top screw caps with Teflon /silicone liners purchased formAlltech (Deerfield, IL, USA). The SPME syringe was used to pierce the septa and thefiber was then exposed to the volume of gases above the liquid culture for approximately30 min in order to adsorb the headspace gases. The fiber was then retracted and thenintroduced into the injection port of the GC and exposed for the duration of thetemperature program (initiated immediately after exposure of the fiber).CE SamplesReagentsThe reagents used in the CE analysis were obtained from the following sources:potassium dihydrogen phosphate (KH2PO4) from Sigma-Aldrich;tetradecyltrimethylammonium bromide from Acros Organics; concentrated hydrochloric
13acid (Mallinckrodt, Paris, Kentucky, USA); and methanol from Burdick & Jackson(Muskegon, MI, USA).ApparatusA P/ACE MDQ CE system (Beckman Coulter, Fellerton, CA, USA) was used inall CE experiments. This system was equipped with an auto sampler and temperaturecontrol for the sample trays and capillary. Fused silica capillaries (also purchased fromBeckman Coulter) with dimensions of 57 cm total length, 50 cm effective length, 75 µmi.d. and 375 µm o.d. were used.CE AnalysisAll CE samples were run according to the methods developed by Pathem et al.(2007).The run
the analysis of selenocyanate from the broth cultures of selenium-resistant bacteria using solid-phase extraction and capillary electrophoresis