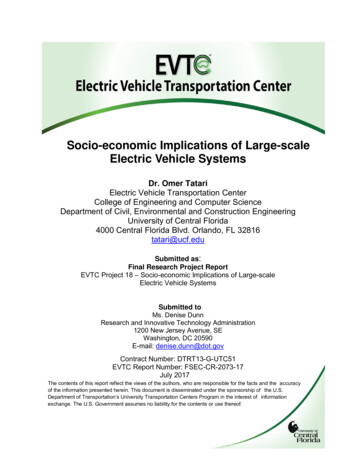
Transcription
Socio-economic Implications of Large-scaleElectric Vehicle SystemsDr. Omer TatariElectric Vehicle Transportation CenterCollege of Engineering and Computer ScienceDepartment of Civil, Environmental and Construction EngineeringUniversity of Central Florida4000 Central Florida Blvd. Orlando, FL 32816tatari@ucf.eduSubmitted as:Final Research Project ReportEVTC Project 18 – Socio-economic Implications of Large-scaleElectric Vehicle SystemsSubmitted toMs. Denise DunnResearch and Innovative Technology Administration1200 New Jersey Avenue, SEWashington, DC 20590E-mail: denise.dunn@dot.govContract Number: DTRT13-G-UTC51EVTC Report Number: FSEC-CR-2073-17July 2017The contents of this report reflect the views of the authors, who are responsible for the facts and the accuracyof the information presented herein. This document is disseminated under the sponsorship of the U.S.Department of Transportation’s University Transportation Centers Program in the interest of informationexchange. The U.S. Government assumes no liability for the contents or use thereof.
AcknowledgementsThis report is a final research report for the Socio-economic Implications of Large-scaleElectric Vehicle Systems project of the Electric Vehicle Transportation Center (EVTC) atthe University of Central Florida (UCF). The Electric Vehicle Transportation Center is aUniversity Transportation Center funded by the Research and Innovative TechnologyAdministration of the U.S. Department of Transportation. The EVTC is a research andeducation center whose projects prepare the U.S. transportation system for the influx ofelectric vehicles into a sustainable transportation network and investigate theopportunity these vehicles present to enhance electric grid modernization efforts. TheEVTC is led by UCF's Florida Solar Energy Center with partners from UCF’sDepartments of Electrical Engineering and Computer Science and Civil, Environmentaland Construction Engineering, the University of Hawaii, and Tuskegee University.The objective of the Socio-economic Implications of Large-scale Electric VehicleSystems project was to develop models to evaluate the socio-economic implications ofa large-scale electrified transportation sector. The developed model included effects ofvehicle and infrastructure safety requirements, standardization of vehicle componentsfor safety and charging, electric vehicle supply and after-market economies,displacement of petroleum fuels and impacts of sustainable development (social,environmental and economic). The work was conducted by Dr. Omer Tatari, PrincipalInvestigator and his research team of the College of Engineering and Computer Scienceat the University of Central Florida.Note is made that the results of the research from this project has been used in thefollowing five PhD dissertations:1. Onat, Nuri (2015). “Integrated Sustainability Assessment Framework for the U.S.Transportation,” Ph.D. Dissertation, Department of Civil, Environmental, andConstruction Engineering, University of Central Florida.2. Noori, Mehdi (2015) “Development of Regional Optimization and MarketPenetration Models for the Electric Vehicles in the United States,” Ph.D.Dissertation, Department of Civil, Environmental, and Construction Engineering,University of Central Florida.3. Alirezaei, Mehdi (2016). “Getting to Net Zero Energy Building: A Holistic TechnoEcological Modeling Approach,” Ph.D. Dissertation, Department of Civil,Environmental, and Construction Engineering, University of Central Florida.4. Ercan, Tolga (2017). “Sustainability Assessment Models for Electric Buses andFleets,” Ph.D. Dissertation, Department of Civil, Environmental, and ConstructionEngineering, University of Central Florida.5. Zhao, Yang (2017) “A Comprehensive Assessment of Vehicle-to-Grid Systemsand Their Impact to the Sustainability of Current Energy and Water Nexus,”Dissertation, Department of Civil, Environmental, and Construction Engineering,University of Central Florida.2
Table of ContentsTable of Contents . 3List of Tables. 6List of Figures . 7List of Abbreviations . 91.0Abstract . 122.0Introduction . 143.0State‐based Comparative Carbon and Energy Footprint Analysis of Electric Vehicles . 193.1Introduction . 193.2Methodology . 203.2.1 Vehicle Production . 213.2.2 Battery Production . 223.2.3 Vehicle operation phase . 233.3Results and Discussions . 253.3.1 State‐based Average Electricity Generation Mix Scenario. 273.3.2 State‐based Marginal Electricity Generation Mix Scenario . 283.3.3 Solar Energy Scenario . 293.3.4 Sensitivity of Key Parameters. 303.3.5 GHG Emission Reduction and Energy Saving Potentials . 343.4Conclusions and Recommendations. 364.0Socio‐Economic Life Cycle Sustainability Assessment Framework for Electric Vehicles394.1Introduction . 394.1.1 Life cycle sustainability assessment . 404.1.2 Broadening and deepening the LCSA framework . 404.1.3 Motivation and research objectives. 414.2Methodology . 424.2.1 Problem statement . 434.2.2 Indicator selection and identification of parameters . 434.2.3 System conceptualization . 454.2.4 Model validation . 484.2.5 Scenario based comparison of each vehicle type . 504.3Results and discussion . 514.3.1 Environmental impacts . 514.3.2 Economic Impacts . 554.3.3 Social Impacts . 564.3.4 Impact of feedbacks from causal loops. 594.4Conclusion . 605.0Development of a regional market penetration model for electric vehicles . 635.1Introduction . 635.1.1 Introduction and Scope of Work . 635.1.2 Life Cycle Analysis, Agent Based Modeling, and Market Penetration of Electric Vehicles . 645.2Methods . 655.2.1 Electric Vehicle Regional Optimizer (EVRO) . 663
5.2.2 Exploratory Modeling and Analysis . 675.2.3 Agent‐Based Modeling . 685.2.4 Assumptions and Preliminary Data . 685.2.5 Verification and Validation of ABM. 725.3Analysis Results . 745.3.1 Maintenance and Refueling Cost . 755.3.2 Environmental Damage Cost Results . 775.3.3 Water Footprint Results . 785.3.4 Agent Based Modeling Results . 795.4Discussion and Limitations. 826.0Applications of Vehicle‐to‐Grid Technology for Transit and School Buses. 856.1Introduction . 856.2Materials and Methods . 876.2.1 Environmental Emission Calculation Methods . 876.2.2 Air Pollution Externality Calculation Methods . 906.2.3 Cash Flow and Net Revenue Calculation Methods . 916.3Data Collection . 956.3.1 Transit and School Bus Specifications . 956.3.2 Vehicle‐to‐Grid System Specifications . 976.4Results . 976.4.1 Cash Flow Results . 976.4.2 Environmental Emission Results . 1016.4.3 Air Pollution Externality Results . 1026.5Discussion . 1037.0Getting to net zero energy building: investigating the role of vehicle to hometechnology . 1057.1Introduction . 1057.1.1 Net Zero Energy Building (NZEB) . 1067.1.2 Vehicle to Home (V2H) Technology . 1077.2Methodology . 1087.2.1 Model Development . 1097.2.2 Optimization of the Building’s Energy Performance . 1107.2.3 . Power Supply System . 1137.3Power Distribution System . 1177.4Time‐Based Electricity Pricing . 1197.5Results and Discussion . 1207.5.1 Energy Analysis Results . 1207.5.2 Electricity Consumption . 1227.5.3 Electricity to Grid . 1237.5.4 Price Comparison . 1247.5.5 Sensitivity Analysis . 1257.6Conclusion . 1288.0Socio‐economic analysis of alternative fuel‐powered Class 8 heavy‐duty trucks . 1308.1Introduction . 1308.1.1 Overview . 1308.1.2 Objective of the Study . 1304
8.2Methods and Materials . 1318.2.1 Hybrid Life‐Cycle Assessment . 1318.2.2 Monte Carlo Analysis . 1328.2.3 Life‐Cycle Inventory . 1328.3Results . 1378.3.1 Life‐Cycle Cost (LCC) Analysis Results . 1378.3.2 Environmental Emissions Results . 1388.3.3 APE Cost Results . 1408.3.4 Cost and GHG Emissions Results for Regional Electricity Consumption . 1428.4Conclusions and Discussions . 1439.0References . 1465
List of TablesTable 4-1 Model boundary . 44Table 4-2 Results of the ANOVA analysis . 49Table 4-3 Results of Normality Tests . 49Table 4-4 Results of Two Sample Kolmogorov Smirnov . 50Table 4-5 Summary of the extreme scenarios . 51Table 4-6 The differences in key parameters due to feedback effects between 2015 and2050. . 60Table 5-1 Model Parameters in EVRO Model . 69Table 5-2 Scaled Probability of Purchase . 69Table 5-3 Vehicle Purchase Price (MSRP) . 69Table 5-4 Preference Values for Each Cost Attribute (Low Income Category) . 70Table 5-5 Preference Values for Each Cost Attribute (Medium Income Category) . 70Table 5-6 Preference Values for Each Cost Attribute (High Income Category) . 71Table 5-7 Government Incentives [109,157] . 71Table 6-1 Explanations of notations and indexes. . 90Table 6-2 Specifications of bus types and V2G system. . 92Table 6-3 Average lifetime cash flow analysis of transit and school buses in CAISOregion. . 100Table 7-1 Modeled Building’s Specifications . 109Table 7-2 Optimization Analysis Iterations . 113Table 7-3 Solar Panel Characteristics. 115Table 7-4 Model Parameters . 117Table 7-5 Hourly Electricity Pricing . 120Table 7-6 Energy Analysis Results . 121Table 7-7 Temperature Data for Different Months of the Year . 121Table 7-8 Required Electricity from the Grid for Different Values of SOC . 126Table 7-9 Amount of Electricity Transferred to the Grid for Different Values of MainBattery Capacity . 126Table 8-1 Vehicle characteristics and battery specifications . 133Table 8-2 Inputs for hybrid life-cycle assessment . 1366
List of FiguresFigure 3.1 System Boundary of the Analysis . 21Figure 3.2 Life cycle impacts of each vehicle type; a) GHG emissions, b) Energyconsumption . 26Figure 3.3 State level vehicle preference according to scenario 1; a) GHG emissions, b)Energy consumption. 28Figure 3.4 State level vehicle preference in the terms of GHG emissions for scenario 2. 29Figure 3.5 LCA impacts as a function of GHG and energy intensity, a) GHG emissions,b) Energy consumption per kilometer vehicle traveled. . 31Figure 3.6 LCA impacts as a function of UF varying from 0 to 1, a) GHG emissions, b)Energy consumption per kilometer vehicle travels. . 33Figure 3.7 State-specific sensitivity results based on average electric power generationmixes a) WV, b) MN, c) VT, d) DC, e) NE, f) ID . 34Figure 3.8 Reduction potentials as a function of market penetration rates, a) percentagereduction of GHG emissions, b) Percentage reduction of energy consumption . 36Figure 4.1 Atmospheric temperature change between 1980 and 2014 . 43Figure 4.2 Causal loop diagram of the model . 46Figure 4.3 CO2 emissions from vehicle transportation a) Manufacturing Phase, b)Operation Phase, c) Total Life Cycle Emissions . 52Figure 4.4 PMF from vehicle transportation a) Manufacturing Phase, b) OperationPhase, c) Total Life Cycle . 53Figure 4.5 POF from vehicle transportation a) Manufacturing Phase, b) OperationPhase, c) Total Life Cycle . 54Figure 4.6 Annual vehicle ownership costs a) Operation Phase, b) Total Life Cycle . 55Figure 4.7 Contribution to GDP a) Manufacturing Phase, b) Operation Phase, c) TotalLife Cycle . 56Figure 4.8 Contribution to employment a) Manufacturing Phase, b) Operation Phase, c)Total Life Cycle . 57Figure 4.9 Human health impacts a) Manufacturing Phase, b) Operation Phase, c) TotalLife Cycle . 58Figure 5.1 Illustration of the EVReMP model . 66Figure 5.2 Vehicle Sales by Vehicle Technology for the Base Case (1000s/year) . 74Figure 5.3 NEMS Electricity Market Module Regions [172] . 75Figure 5.4 Maintenance and Refueling Costs of Studied Vehicles Throughout the U.S.(in Thousands of Dollars) . 76Figure 5.5 a. Maintenance and Refueling Cost of Internal Combustion Engine Vehiclefor different regions and b. Maintenance and Refueling Cost of All-Electric Vehicle fordifferent regions, both in Thousand Dollars . 77Figure 5.6 Life Cycle Environmental Damage Costs for the studied vehicles in Millionsof Dollars (a), and Water Footprints of the studied vehicles in Thousands of Gallons (b). 78Figure 5.7 Configuration of agents in the ABM model . 797
Figure 5.8 Vehicle Sales by Vehicle Technology for the Government SubsidiesScenario (1000s/year) . 80Figure 5.9 Variations in the Market Penetrations of the studied vehicles under theGovernment Subsidies scenario (1000s/year) . 81Figure 5.10 Vehicle Sales by Vehicle Technology for the Government Subsidies andWOM Scenario (1000/year) . 82Figure 6.1 Transit and school buses’ environmental emissions data collection andanalysis path. . 88Figure 6.2 Cumulative cash flow of transit diesel (average) and BE (regional) buses. . 99Figure 6.3 Cumulative cash flow of school diesel (average) and BE (regional) buses. 99Figure 6.4 Regional net revenue of V2G service for transit (a) and school (b) busoptions. 101Figure 6.5 Regional average of cumulative GHG emission savings in 2027 for transitand school battery electric (BE) bus due to V2G availability [tons]. . 102Figure 6.6 Total air pollution externalities of bus and fuel types. . 102Figure 7.1 Net Zero Energy Building. . 108Figure 7.2 Developed Methodology . 109Figure 7.3 Developed Model in Designbuilder . 110Figure 7.4 Optimization Analysis Results . 112Figure 7.5 PV System Components . 114Figure 7.6 Schematic View of Sun-Path Diagram . 115Figure 7.7 Power Distribution System Algorithm . 119Figure 7.8 Daily Energy Consumption of The Building . 122Figure 7.9 Comparison of Hourly Energy Consumption of the Building for Conventionaland NZEB Scenarios . 123Figure 7.10 Comparison of Cumulative Energy Consumption of the Building forConventional and NZEB Scenarios . 123Figure 7.11 Monthly Amount of Electricity Transferred to the Grid . 124Figure 7.12 Monthly Electricity Bill Price Comparison . 125Figure 7.13 Electricity from the Grid for Different Ranges of SOC . 126Figure 7.14 Electricity Transferred to the Grid with different ranges of main batterycapacity. . 127Figure 8.1 System boundary for hybrid-life cycle assessment. 133Figure 8.2 Life cycle costs of heavy-duty trucks . 138Figure 8.3 Life-cycle greenhouse gas emissions of heavy-duty trucks . 139Figure 8.4 Life-cycle air pollutants emissions of heavy-duty trucks . 140Figure 8.5 Life-cycle air pollution externalities . 141Figure 8.6 Regional electricity-consumption-related greenhouse gas emissions for 400kWh electricity . 142Figure 8.7 Regional life cycle cost of electricity for 400 kWh electricity . 1438
List of HEVICVIEAIPCCISO-NEISOAgent Based ModelAll-electric rangeAlternative Fuel Life-Cycle Environmental and Economic TransportationArgonne National LaboratoryAir pollution externalitiesAir pollution emission experiments and policy analysisAmerican Transportation Research InstituteBusiness as UsualBattery Electric VehicleBattery electricCorporate Average Fuel EconomyCalifornia independent system operatorsCompressed natural gasCumulative Distribution FunctionCarbon monoxideCarbon DioxideConsumer Price IndexDepartment of EnergyEnvironmental Damage CostEnergy Information AgencyEconomic Input-Output Life Cycle AssessmentExploratory Modeling and AnalysisUnited States Environmental Protection AgencyElectric Reliability Council of TexasGasoline Extended Range Electric VehicleElectric VehicleElectric Vehicle Transportation CenterElectric Vehicles Regional OptimizerElectric Vehicle Regional Market PenetrationFederal Highway AdministrationCarbon dioxide equivalentGross domestic productGlobal hectare areaGreenhouse gasThe Greenhouse Gases, Regulated Emissions, and Energy Use inTransportationGlobal warming potentialHeavy-duty TruckHybrid electric vehicleInternal combustion vehicleInternational Energy Agency’sIntergovernmental Panel on Climate ChangeIndependent system operators of new England regionInternational standards organization9
SFEltM&RMDVMJMOVE
College of Engineering and Computer Science Department of Civil, Environmental and Construction Engineering University of Central Florida 4000 Central Florida Blvd. Orlando, FL 32816 tatari@ucf.edu Submitted as: Final Research Project Report EVTC Project 18 - Socio-economic Implications of Large-scale Electric Vehicle Systems Submitted to