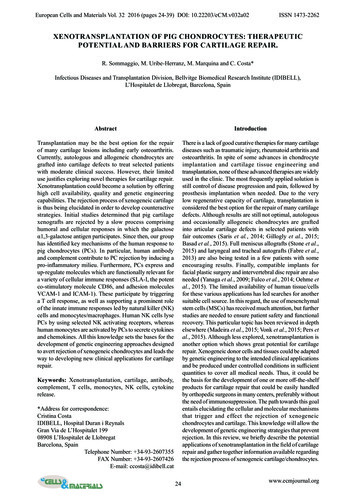
Transcription
EuropeanCells etandISSN 1473-2262R Sommaggioal.Materials Vol. 32 2016 (pages 24-39) DOI: 10.22203/eCM.v032a02Barriers to chondrocyte xenotransplantationXENOTRANSPLANTATION OF PIG CHONDROCYTES: THERAPEUTICPOTENTIAL AND BARRIERS FOR CARTILAGE REPAIR.R. Sommaggio, M. Uribe-Herranz, M. Marquina and C. Costa*Infectious Diseases and Transplantation Division, Bellvitge Biomedical Research Institute (IDIBELL),L’Hospitalet de Llobregat, Barcelona, SpainAbstractIntroductionTransplantation may be the best option for the repairof many cartilage lesions including early osteoarthritis.Currently, autologous and allogeneic chondrocytes aregrafted into cartilage defects to treat selected patientswith moderate clinical success. However, their limiteduse justifies exploring novel therapies for cartilage repair.Xenotransplantation could become a solution by offeringhigh cell availability, quality and genetic engineeringcapabilities. The rejection process of xenogeneic cartilageis thus being elucidated in order to develop counteractivestrategies. Initial studies determined that pig cartilagexenografts are rejected by a slow process comprisinghumoral and cellular responses in which the galactoseα1,3-galactose antigen participates. Since then, our grouphas identified key mechanisms of the human response topig chondrocytes (PCs). In particular, human antibodyand complement contribute to PC rejection by inducing apro-inflammatory milieu. Furthermore, PCs express andup-regulate molecules which are functionally relevant fora variety of cellular immune responses (SLA-I, the potentco-stimulatory molecule CD86, and adhesion moleculesVCAM-1 and ICAM-1). These participate by triggeringa T cell response, as well as supporting a prominent roleof the innate immune responses led by natural killer (NK)cells and monocytes/macrophages. Human NK cells lysePCs by using selected NK activating receptors, whereashuman monocytes are activated by PCs to secrete cytokinesand chemokines. All this knowledge sets the bases for thedevelopment of genetic engineering approaches designedto avert rejection of xenogeneic chondrocytes and leads theway to developing new clinical applications for cartilagerepair.There is a lack of good curative therapies for many cartilagediseases such as traumatic injury, rheumatoid arthritis andosteoarthritis. In spite of some advances in chondrocyteimplantation and cartilage tissue engineering andtransplantation, none of these advanced therapies are widelyused in the clinic. The most frequently applied solution isstill control of disease progression and pain, followed byprosthesis implantation when needed. Due to the verylow regenerative capacity of cartilage, transplantation isconsidered the best option for the repair of many cartilagedefects. Although results are still not optimal, autologousand occasionally allogeneic chondrocytes are graftedinto articular cartilage defects in selected patients withfair outcomes (Saris et al., 2014; Gillogly et al., 2015;Basad et al., 2015). Full meniscus allografts (Stone et al.,2015) and laryngeal and tracheal autografts (Fabre et al.,2013) are also being tested in a few patients with someencouraging results. Finally, compatible implants forfacial plastic surgery and intervertebral disc repair are alsoneeded (Yanaga et al., 2009; Fulco et al., 2014; Oehme etal., 2015). The limited availability of human tissue/cellsfor these various applications has led searches for anothersuitable cell source. In this regard, the use of mesenchymalstem cells (MSCs) has received much attention, but furtherstudies are needed to ensure patient safety and functionalrecovery. This particular topic has been reviewed in depthelsewhere (Madeira et al., 2015; Vonk et al., 2015; Pers etal., 2015). Although less explored, xenotransplantation isanother option which shows great potential for cartilagerepair. Xenogeneic donor cells and tissues could be adaptedby genetic engineering to the intended clinical applicationsand be produced under controlled conditions in sufficientquantities to cover all medical needs. Thus, it could bethe basis for the development of one or more off-the-shelfproducts for cartilage repair that could be easily handledby orthopedic surgeons in many centers, preferably withoutthe need of immunosuppression. The path towards this goalentails elucidating the cellular and molecular mechanismsthat trigger and effect the rejection of xenogeneicchondrocytes and cartilage. This knowledge will allow thedevelopment of genetic engineering strategies that preventrejection. In this review, we briefly describe the potentialapplications of xenotransplantation in the field of cartilagerepair and gather together information available regardingthe rejection process of xenogeneic cartilage/chondrocytes.Keywords: Xenotransplantation, cartilage, antibody,complement, T cells, monocytes, NK cells, cytokinerelease.*Address for correspondence:Cristina CostaIDIBELL, Hospital Duran i ReynalsGran Via de L’Hospitalet 19908908 L’Hospitalet de LlobregatBarcelona, SpainTelephone Number: 34-93-2607355FAX Number: 34-93-2607426E-mail: ccosta@idibell.cat24www.ecmjournal.org
R Sommaggio et al.Barriers to chondrocyte xenotransplantationCurrent Clinical NeedsCartilage is unable to develop a satisfactory healingresponse after injury or disease. Even small damageproduced by traumatic injury is difficult to repair. Purechondral defects, such as those that do not penetrate theunderlying subchondral bone in joints, are unable to repairby themselves (the lack of vessels does not allow thefast access of macrophages and native MSCs) and maypass undetected (cause no pain without innervations).Full-thickness chondral and osteochondral defectsproduce pain and inflammation, but do not have a betteroutcome. Notably, hyaline cartilage lesions, unlesstreated appropriately, generate a fibrous scar that hasdifferent structure, composition and inferior mechanicalproprieties than the original cartilage. Furthermore, theselesions often progress towards OA affecting the entirejoint. Interestingly, fibrocartilage, such as in menisci,also does not repair spontaneously. Therefore, the greatmedical needs and the prospect of better therapies havepromoted intense research in cartilage transplantation andtissue engineering, especially for the cure of traumaticjoint injuries in athletes. As mentioned, other indicationsunder study are nasal, auricular and laryngeal/trachealreconstructions, as well as intervertebral disc repair. Ascheme of current clinical needs for cartilage repair isshown in Fig. 1.Cartilage defects or disease can affect all kinds of cartilage.The main types of cartilage are: 1) Hyaline cartilage, themost abundant, which is located at the end of long bones,anterior ends of ribs, nose, parts of the larynx, trachea,bronchi, bronchial tubes and the embryonic/fetal skeleton.It provides flexibility and support, and in the joints reducesfriction and absorbs shock. 2) Elastic cartilage, located onthe top of the epiglottis, in the auricle of the ears and inthe Eustachian tubes. It provides strength and elasticity,and maintains shape. 3) Fibrous cartilage, the strongest ofthe three, composes the pubic symphysis, intervertebraldiscs and menisci.The origin of cartilage defects can be traumatic injury(acute or chronic), surgical resection associated withmalignancies, and in some cases congenital (microtia/anotia for defective or missing ear/s). Regarding cartilagediseases, the most common is osteoarthritis (OA)followed by rheumatoid arthritis (RA). OA is an agingrelated disease, especially prevalent in obese patients,causing disability in many people worldwide (GlynJones et al., 2015; Musumeci et al., 2015). It affectsthe articular cartilage, but also all structures of weightbearing joints. The inflammatory response associatedwith this disease induces cartilage deterioration throughabnormal remodeling of extracellular matrix and loss ofjoint structure (Malemud, 2015; Musumeci et al., 2015).RA is a chronic and systemic inflammatory disorderwith autoimmune and innate-immunity componentsthat principally attacks the joints (Arend and Firestein,2012; Catrina et al., 2016). RA produces synovitis thatoften progresses to destruction of articular cartilage andankylosis of the joint. Clinical symptoms which differ fromOA are the fast and potentially early onset, the symmetry ofaffected joints (in both sides) and the presence of systemicsymptoms such as fatigue. Both of these diseases aremostly managed with anti-inflammatory agents, includingexpensive biological therapies such as anti-TNF for RA.Despite their therapeutic efficacy, there is still a pressingneed for better treatments.Currently Available Therapies for Cartilage RepairSeveral therapeutic options are available for the repairof damaged articular cartilage. Microfracture, usedfor promoting subchondral bleeding and bone marrowstimulation, is the simplest procedure for treating articularchondral defects. It is recommended in the case of smalllesions ( 2 cm2), as it basically results in fibrocartilage(Oussedik et al., 2015). For this reason, some teams areworking on a related technique called autologous matrixinduced chondrogenesis that combines microfracturewith the implantation of a cover scaffold that stabilizesthe clot (Lee et al., 2014). The impact of this particularstrategy remains to be clarified after longer experience.Fig. 1. Scheme of the current clinical needs for cartilage repair categorized by type of cartilage, affected structure andgeneral cause of pathology.25www.ecmjournal.org
R Sommaggio et al.Barriers to chondrocyte xenotransplantationis restricted for economical and technical reasons to a fewcenters treating a limited number of patients. The mostaccepted approaches utilize autologous chondrocytes,although implantation of allogeneic chondrocytes isalso feasible. The autogeneic transplantation comprisesthe autologous chondrocyte implantation (ACI) andthe next-generation procedure called matrix-induced ormatrix-assisted ACI (MACI) because it involves the useof a filling material (Madeira et al., 2015). ACI and MACIboth require the harvest of thin slides of cartilage fromminor weight-bearing areas of the joint cartilage, whichare subjected to enzymatic digestion for subsequent exvivo expansion of chondrocytes for several weeks andfinal implantation into the defect. Significant and durablebenefits have been demonstrated for patients subjected tothese procedures in terms of diminished pain and improvedfunction. Clinical values of repair, such as the degree ofintegration of donor cartilage, macroscopic appearance andmechanical assessment, are consistently good. RegardingACI in particular, up-to-20-years repair has already beendocumented in some cases and the use of a porcinederived membrane to cover the defect has diminishedthe inconveniencies of the first-generation ACI (whichinvolved a periostal flap) (Gillogly et al., 2015). Althoughthe experience with MACI is shorter in time, it has beendemonstrated to be more effective than microfracture forknee defects larger than 3 cm2 (Saris et al., 2014). For now,the available experience shows that MACI is technicallyeasier than ACI, but it is unclear whether it is superior interms of quality of repair and clinical benefit. The existenceof different matrices and associated products, as well asmedical centers for its application adds complexity anddifficulty for evaluating efficacy (Madeira et al., 2015).Nonetheless, either ACI or MACI seem to be the firstchoice for treating this type of lesion, whereas otherapproaches could be applied for patients failing to respondwell to these therapies (Basad et al., 2015).Despite these promising clinical results, the use ofthese advanced therapies carries a number of limitations.One major issue is the high cost of the procedure. Anotheris the low tissue availability and the loss of phenotypeof the chondrocytes during expansion (Madeira et al.,2015). As a result, the quality of the cartilage generated iscompromised (Cucchiarini et al., 2014). Early magneticresonance studies indicated the presence of fibrous tissuein the repair areas after ACI, which was later confirmedby quantitative analyses (Långsjö et al., 2010). In otherstudies, the chondrocyte implantation occurred at a slowerrate than in osteochondral transplantation and the graftshowed an inhomogeneous hyaline appearance (Horaset al., 2003). Other considerations for these techniquesinclude the patient age, the rehabilitation regimens,postoperative joint alignment and surgical history (Bertaet al., 2015). The lack of a consistent repair with hyalinecartilage after ACI or MACI has led to the proposal ofcombination therapy, such as microfracture with MACI(Cucchiarini et al., 2014). It is an interesting concept,but it does not imply a clear reduction in costs, unless theisolation and/or culture of chondrocytes is avoided.For larger defects, a more frequently-used approach isosteochondral graft transplantation that is commonly basedon implantation of an autograft obtained from a healthy andlow-stress area in the same joint. Depending on the severity,shape and overall size of the damage, multiple plugs oruse of allogeneic tissue may be required to adequatelyrepair the joint. Mosaicplasty, in particular, involves theimplantation of multiple autologous osteochondral plugsthat replace damaged cartilage on the joint surface. Asdescribed by Berta et al. (2015), this technique offerseffective management of small and medium-sized focaldefects although it does not result in good hyaline cartilage.Allotransplantation is particularly considered forsettings that require whole structures such as menisci,large number of cells and/or when other techniques ofcartilage repair have failed (Stone et al., 2015; Gracitelliet al., 2015). Despite the clinical benefit attained withmeniscus allotransplantation and osteochondral allografttransplantation (Stone et al., 2015; Gracitelli et al., 2015),some concerns have been raised about the potentialdetrimental effects of the elicited immune response.Evidence is accumulating of the immune-privilegedproperties of cartilage, but isolated chondrocytes andespecially the bony portion of the osteochondral grafts aremore immunogenic (Smith et al., 2015). Early work pointedout that allogeneic chondrocytes may elicit an immuneresponse when injected heterotopically in rats (Romaniuket al., 1995). In this model, new cartilage-like tissuewas formed, but over time it was destroyed displayingan infiltrate of mononuclear immune cells (comprisedmainly by macrophages shortly after transplantation anda large population of NK and T cells at later stages). Onthe other hand, harvest and storage of the donor tissue ina timely manner can be difficult. Consequently, limitationsassociated with cryopreservation or prolonged refrigeratedstorage have been described. such as reduced viabilityand metabolism of chondrocytes (Berta et al. 2015). Insummary, there are also disadvantages for therapies basedon chondrocyte/cartilage allotransplantation.Cartilage diseases very often lead to a point of painand loss of function that is only solved by utilizing amedical device. Orthopedic prostheses made of stainlesssteel and titanium alloys in addition to other materials arefrequently used for joint replacements (Dearnley, 1999).Spinal fixation devices are also being considered asprosthetic solutions when intervertebral discs are failing(Slone et al., 1993). As advantages, these orthopedicimplants are immediately available and are designedto be adapted and fixed within minutes leading to fastreconstruction. However, the process of implantation ofprosthetic devices is costly, highly traumatic and can leadto multiple complications, such as infection, inflammationand pain. Furthermore, such prosthetic implants have alimited lifespan (Glyn-Jones et al., 2015) and pose the riskof inducing biomaterial hypersensitivity (Mitchelson et al.,2015).Transplantation of human chondrocytesStrategies based on transplantation of chondrocytesare currently the most successful therapies for articularcartilage repair. Nevertheless, its use in clinical practice26www.ecmjournal.org
R Sommaggio et al.Barriers to chondrocyte xenotransplantationState of the Art in Xenotransplantationminutes to hours after xenotransplantation of non-transgenicpig organs through the massive deposition of xenoreactivenatural antibodies (XNA) and complement on the donorendothelium resulting in hemorrhage, thrombosis, ischemiaand necrosis (Vadori and Cozzi, 2015; Cooper et al., 2015).These XNA are mainly IgM that bind the carbohydrateantigen Gal, which is highly expressed in pig tissues andsynthesized by the α1,3-galactosyltransferase (α1,3-GT)(Vadori and Cozzi, 2015). Humans produce abundantnatural anti-Gal antibodies because they lack a functionalα1,3-GT and as a result do not express Gal residues.Notably, HAR has been precluded by eliminating Galfrom pig tissues by homologous recombination and nucleartransfer/cloning technology, as well as by expression ofhuman complement regulatory proteins (Costa Vallés andMáñez Mendiluce, 2012; McGregor et al., 2012; Cooperet al., 2016). Now, the genetically-modified pig organstransplanted into non-human primate models succumb toAHXR within weeks to months. AHXR involves a stronghumoral response with participation of elicited antibodies,complement activation and thrombosis. It is characterizedby the presence of an innate immune cellular infiltrate(macrophages and NK cells) and a type-II endothelialcell-activation phenotype that promotes fibrin depositionand intravascular thrombosis (Byrne et al., 2013; Vadoriand Cozzi, 2015; Cooper et al., 2015). Understanding themolecular interactions between the human innate immunecells and the xenograft is highly relevant to establishprotective interventions (Wang and Yang, 2012). Finally,systemic inflammation is also observed in this setting(Ezzelarab et al., 2015). Thus, the process of AHXRis the most pressing problem in xenotransplantation ofsolid organs today. Nevertheless, additional carbohydrateantigens recognized by XNA (initially termed non-Gal)have been identified in the pig, and the effect of theirelimination (Estrada et al., 2015) remains to be determinedin preclinical studies.The complement system plays a relevant role in thecourse of any inflammatory response (Carroll and Sim,2011). In AHXR, the classical pathway triggered byantibody deposition initially generates membrane-boundC4b and C3b as well as the production of anaphylatoxinsC4a and C3a. C3 activation will also trigger the alternativepathway, which will further amplify the complementcascade. C4b, C3b and C3 breakdown products bindseveral complement receptors expressed on immune cellspromoting B cell activation and phagocytosis (Carroll andSim, 2011). The three complement-activation pathways,the classical, alternative and lectin pathways, convergein the formation of C5 convertases that generate theterminal membrane attack complex (C5b-9) and themost potent anaphylatoxin, C5a, leading to cytolysisand inflammation (John et al., 2007; Sacks and Zhou,2012). Thus, anaphylatoxins cause enhanced vascularpermeability, chemotaxis, inflammation, generation ofcytotoxic oxygen radicals and histamine release frommast cells. A T-cell-mediated response against pig antigenstakes place within days after xenotransplantation. Theactivation of T cells during xenograft rejection is mediatedthrough the T cell receptor (TCR) and co-stimulatorysignals conserved across species, involving both directXenotransplantation has gained attention in the last decadesas it could be used for the treatment of many diseasescharacterized by tissue loss or failure (Ekser et al., 2012).Based on the transplantation of cells, tissues and organsacross different species, it has the potential to overcomethe severe shortage of human cells, tissues and organs forclinical transplantation (Ekser et al., 2015). Its applicabilityhas gained feasibility with the recent advancements madein the field of animal genetic engineering (Estrada et al.,2015; Yang et al., 2015; Cooper et al., 2016; Niemann andPetersen, 2016). Xenotransplantation offers an unlimitedsupply of cells that can be obtained under very high qualitystandards (Ekser et al., 2015). Accordingly, hormones anddecellularized or devitalized tissues of animal origin arealready being used in the clinic for therapeutic purposes(Vadori and Cozzi, 2015). New therapies could bedeveloped in the future by using xenogeneic cells andtissues selected by their regenerative capacity and adaptedto the intended clinical application by genetic engineering(Costa et al., 2003; Sommaggio et al., 2015).The pig is now considered the best animal source forxenotransplantation because it is domesticated, reproducesin large scale and has a primate-like size of organs andphysiology. Consequently, major efforts and advancementshave been made to genetically modify pigs to the germ-linelevel using various technologies (Lai et al., 2002; Estrada etal., 2015; Yang et al., 2015). Thus, the pig has been chosenas the most appropriate animal source not only for solidorgan xenotransplantation, but also for the developmentof xenogeneic cellular therapies (Ekser et al., 2012).Immune rejection is the main hurdle to overcome forxenotransplantation to become a broad clinical reality.Three types of rejection could be assigned to solid organxenotransplatation depending on the time of rejection:hyperacute rejection (HAR), acute xenograft rejection(AXR) comprising acute humoral xenograft rejection(AHXR) and acute cellular xenograft rejection, and in afew cases of long-term survival, there are pathologicalfeatures consistent with chronic xenograft rejection(Cooper et al., 2015). HAR basically results from ahumoral response. In general, humoral responses arethose mediated by antibodies (produced by B cells) andtheir effector functions through complement activation,promotion of phagocytosis and antibody-dependentcell-mediated cytotoxicity (ADCC). In contrast, cellularresponses are mediated by T cells that can develop directeffector functions (cytotoxicity) or enhance the activity ofother cells such as B cells and macrophages (Griesemer etal., 2014). As mentioned, AXR comprises both humoraland cellular responses, but the balance is shifted towardsa predominant humoral response. Humoral and cellularmechanisms also cause rejection of xenogeneic cellsand tissues. Importantly, many molecules and pathwaysinvolved are shared by the rejection processes of thedifferent xenografts in spite of relevant particularities foreach cell/tissue type.The humoral arm of the immune response is criticalin rejection of vascularized xenografts causing HAR andAHXR in non-human primates. HAR takes place within27www.ecmjournal.org
R Sommaggio et al.Barriers to chondrocyte xenotransplantationTable 1. Potential targets for intervention to genetically modify the pig chondrocytes for use in xenogeneictherapies for cartilage repair.Potential therapeutic targetsGal antigenNon-Gal antigensHuman complement regulatory proteinsPig VCAM-1Pig ICAM-1Pig IL-6Pig IL-8Human IL-1αHuman TNFαPig CD86Human CD47Human CD200OtherFunctionCarbohydrate phenotypeComplement regulationAdhesion moleculesCytokines/chemokines secreted by PCsCytokines acting on PCsCo-stimulatory moleculesInhibitory moleculesOxidative stress, apoptosis, cell activationand are influenced by intrinsic properties that conferimmune privilege. As a start, the fact that cartilage isavascular certainly facilitates its protection from immuneattack. Consequently, pig cartilage is not subjected to afast rejection process equivalent to HAR of vascularizedorgans. On the contrary, it is slowly rejected by a delayedprocess, comprising humoral and cellular responses, thatprogresses over time from the edges towards the inside(Stone et al., 1997; Costa et al., 2003). Our group hasbeen working during the last decade on elucidating themolecular basis of this cartilage rejection process (Costaet al., 2008; Sommaggio et al., 2009; Sommaggio et al.,2012; Sommaggio et al., 2013). Here, we will review thiswork together with that of others and include new resultsfrom our group. A list of potential targets for interventionis provided in Table 1.and indirect pathways (Griesemer et al., 2014; Vadoriand Cozzi, 2015; Higginbotham et al., 2015). The variousimmunosuppressive protocols currently available or inadvanced development are considered sufficient to controlthe T-cell immune response against the xenograft (Cooperet al., 2016). In fact, the cellular mechanisms that lead torejection of cellular grafts are probably very similar andhave been successfully controlled in some experimentsresulting in long-term survival (i.e. pig pancreaticislets) (Hering et al., 2006). Notably, blockade of theCD40-CD154 co-stimulatory pathway with anti-CD40antibodies is producing very encouraging results in solidorgan xenotransplantation (Higginbotham et al., 2015).Interestingly, the development of cell therapies for immunemodulation, such as infusion of expanded regulatory Tcells or mesenchymal stem cells (Griesemer et al., 2014;Higginbotham et al., 2015), seems especially attractivefor combinatorial approaches with xenogeneic cellulartherapies. In summary, great advances have been madein the xenotransplantation field since the use of the firstgenetically modified pigs. Although the immunological andnon-immunological responses triggered by the xenograftstill avert its clinical application, the tools to overcomethese barriers are now available. Therefore, key moleculesinvolved in xenograft rejection can be identified andmodified to attain long-term engraftment.Humoral responseDeposition of human XNA initiates the humoral responsetowards pig cartilage (Sommaggio et al., 2013). XNAbind to pig chondrocytes (PCs) by mainly recognizingthe carbohydrate antigen Gal, ubiquitously present on pigcells (Galili et al., 2013; Vadori and Cozzi, 2015). Earlystudies were initially conducted to overcome this effectby modifying the pig cartilage following two differentstrategies. First, Stone et al. (1998) demonstrated thatpig meniscus and articular cartilage specimens incubatedwith α-galactosidase resulted in complete removal of theGal epitopes. Despite this, most animals implanted withα-galactosidase-pretreated grafts produced antibodiesagainst other pig cartilage antigens. Notably, the cellularresponse and the proportion of T lymphocytes was lowerwithin the α-galactosidase-treated xenografts than inuntreated cartilage (Stone et al., 1998). Later studiesproduced similar results when using cartilage fromtransgenic pigs expressing human α1,2-fucosyltransferase(HT), which leads to a dramatic reduction in Gal expression(Costa et al., 2003). Notably, subcutaneous transplantationof HT cartilage in Gal-deficient mice induced no anti-Galantibody response and resulted in lower titers of total antipig antibodies when compared to identical mice receivingMechanisms of Xenogeneic Cartilage RejectionHumoral and cellular responses lead within weeks tomonths to rejection of pig cartilage transplanted intoprimates (Stone et al., 1997; Stone et al., 1998). Similarobservations have been made in a small animal model(Costa et al., 2003). Although none of the studies reportedto date specifically determines the rejection process ofxenogeneic chondrocytes transplanted orthotopicallyinto articular defects of non-human primates, theavailable results are certainly relevant for many cartilagereconstruction applications. The rejection mechanisms ofxenogeneic cartilage are highly particular to this tissue28www.ecmjournal.org
R Sommaggio et al.Barriers to chondrocyte xenotransplantationFig. 2. Scheme of the mechanisms triggered by human antibodies and complement on PCs. Antibody depositionleads to complement activation and opsonization facilitating recognition and phagocytosis by macrophages. Thecomplement cascade ends with the formation of the membrane attack complex (MAC/C5b-9) resulting in cytolysisand cell death of a small proportion of chondrocytes and local anaphylatoxin generation. Most chondrocytes respondto C5a and C5b-9 by up-regulating activation markers (MHC class I, VCAM-1 and ICAM-1) and secreting IL-6and IL-8. C3a, C5a and IL-8 promote migration and activation of immune cells that contribute to the inflammatorymilieu by releasing multiple inflammatory mediators including TNFα that result in extracellular matrix degradation.The loss of extracellular matrix is enhanced by the up-regulation of catabolic enzymes by IL-6 and C5b-9. C5a andTNFα contribute to local T cell activation.SLA (for swine leukocyte antigen) class I and vascularcell adhesion molecule 1 (VCAM-1) and intercellularadhesion molecule 1 (ICAM-1), as well as secretion ofinterleukin-6 (IL-6) and interleukin-8 (IL-8) (Sommaggioet al., 2013). These effects are mainly mediated by C5ageneration and formation of the membrane attack complexand promote a pro-inflammatory milieu that is consistentwith the cellular immune infiltrates observed in the graftedxenogeneic cartilage which contain predominantly T cellsand macrophages (see below for detailed information).This mechanism also explains (at least in part) the reducedcellular response observed in xenografts devoid of Galantigen as they show diminished antibody reactivity andelicited antibody response of the recipient. The presenceof early eosinophilic infiltrates within xenotransplantedcartilage (Stone et al., 1997) may be caused as well byanaphylatoxin release. In fact, we see eosinophils incartilage grafted in Gal-knockout mice treated with cobravenom factor (which triggers anaphylatoxin release), butnot in grafts of mice pretreated with an anti-C5 blockingantibody (Brokaw and Costa, 2015). Remarkably, thesefindings (summarized in Fig. 2) allow the development ofappropriate genetic engineering strategies to counteract theeffects of antibody and complement, as rece
strategies. Initial studies determined that pig cartilage xenografts are rejected by a slow process comprising humoral and cellular responses in which the galactose α1,3-galactose antigen participates. Since then, our group has identified key mechanisms of the human response to pig chondrocytes (PCs). In particular, human antibody