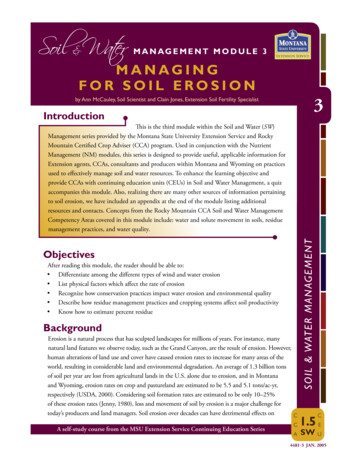
Transcription
Soil & WaterM A N AG E M E N T M O D U L E 3MANAGINGFOR SOIL EROSION3by Ann McCauley, Soil Scientist and Clain Jones, Extension Soil Fertility SpecialistIntroductionObjectivesAfter reading this module, the reader should be able to: Differentiate among the different types of wind and water erosion List physical factors which affect the rate of erosion Recognize how conservation practices impact water erosion and environmental quality Describe how residue management practices and cropping systems affect soil productivity Know how to estimate percent residueBackgroundErosion is a natural process that has sculpted landscapes for millions of years. For instance, manynatural land features we observe today, such as the Grand Canyon, are the result of erosion. However,human alterations of land use and cover have caused erosion rates to increase for many areas of theworld, resulting in considerable land and environmental degradation. An average of 1.3 billion tonsof soil per year are lost from agricultural lands in the U.S. alone due to erosion, and in Montanaand Wyoming, erosion rates on crop and pastureland are estimated to be 5.5 and 5.1 tons/ac-yr,respectively (USDA, 2000). Considering soil formation rates are estimated to be only 10–25%of these erosion rates (Jenny, 1980), loss and movement of soil by erosion is a major challenge fortoday’s producers and land managers. Soil erosion over decades can have detrimental effects onCA self-study course from the MSU Extension Service Continuing Education SeriesSOI L & WAT E R M AN AG E M E N TThis is the third module within the Soil and Water (SW)Management series provided by the Montana State University Extension Service and RockyMountain Certified Crop Adviser (CCA) program. Used in conjunction with the NutrientManagement (NM) modules, this series is designed to provide useful, applicable information forExtension agents, CCAs, consultants and producers within Montana and Wyoming on practicesused to effectively manage soil and water resources. To enhance the learning objective andprovide CCAs with continuing education units (CEUs) in Soil and Water Management, a quizaccompanies this module. Also, realizing there are many other sources of information pertainingto soil erosion, we have included an appendix at the end of the module listing additionalresources and contacts. Concepts from the Rocky Mountain CCA Soil and Water ManagementCompetency Areas covered in this module include: water and solute movement in soils, residuemanagement practices, and water quality.CC 1.5 EASWU4481-3 JAN. 2005
productivity and soil quality because the majority of soilnutrients and soil organic matter (SOM) are stored in thetopsoil, the soil layer most affected by erosion (NM 4, NM15). While temporary solutions, such as increased fertilizer,have offset some of the effects of erosion on productivity,they are not complete substitutes for topsoil (Williams andTanaka, 1996) and represent the greatest input cost forcompensating yield losses caused by erosion (Pimentel etal., 1995). Erosion also impacts the environment beyondthe farm. Runoff can carry fine sediments, nutrients (NM12), and other pollutants (SW 4) to water sources, possiblydegrading water quality. Siltation, or sedimentation, is aleading cause of stream and river impairment in Montanaand the U.S. (EPA, 2000), as it can cause disturbancesin aquatic ecosystems. These include the degradationof fish spawning grounds, the potential reduction ofrecreational activities, increased cost of domestic waterpurification and decreased life span of dams and levies.Furthermore, wind erosion reduces air quality anddamages property due to abrasion and accumulation.Thus, in order to maintain long-term productivity andpreserve soil and environmental quality, it is important tolearn and implement practices that prevent and minimizeerosion, rather than manage the effects of erosion after ithas occurred. The focus of this module is to present bestmanagement practices (BMPs) for managing erosion onagricultural lands in Montana and Wyoming.Soil Erosion ProcessesSoil erosion is the physical movement of soil particlesfrom one location to another, primarily due to forcesof water or wind. The three main phases of soil erosionare detachment, transport and deposition. The severityof erosion depends upon the quantity of soil detachedand the capacity of the wind or water force to transportit (Morgan, 1995). As both detachment and transportrequire energy (deposition occurs when energy is nolonger available), the ability of soils to erode is based on‘erosivity,’ the energy of the eroding agent (i.e., wind orwater), and ‘erodibility,’ the soil’s susceptibility to erosion.Types of erosion and the mechanics and factors affectingeach type are described below.Wind ErosionMechanicsMoving air has energy that can detach and transport soilparticles. Detachment occurs when the energy exerted bywind exceeds the forces keeping the soil particles in place,such as weight and ‘cohesion’ (SW 1). Detachment canalso occur via the impact of particles already in motiondislodging other particles. Once detached, soil can betransported in one of three ways: suspension, saltation,or creep (Figure 1). Suspension is the movement of fineparticles (mainly clay-sized particles and organic matter)high into the air and over long distances. Suspension usuallySuspensionWindSaltationCreepFigure 1. Different types of soil movement by wind. (From USDA, 1989)2Module 3 · Managing for Soil ErosionSaltation
accounts for less than 40% of wind transported particles, yetsince most nutrients are associated with these small particles,suspension can significantly impact soil fertility (Troeh etal., 1999). Saltation occurs primarily with medium-sizedgrains (e.g., fine sand) ‘bouncing’ along the surface. Theimpact of saltating particles on the surface can cause otherparticles to become detached, causing an ‘avalanche’ ofparticles in motion downwind. Saltation typically accountsfor the majority (55–70%) of total soil movement duringa wind event (Morgan, 1995). Unlike suspension, particlesmoved by saltation typically stay on site and accumulate instubble, in cultivation ridges, or along fence lines. Creepis the rolling movement of coarser sand-sized grains andaggregates over the surface. Creep can also cause particlesto become dislodged, though usually not to the extent thatsaltation does, generally accounting for only 10–25% of soilmovement (Morgan, 1995). Creep results in very little soilmovement from the original site, but since aggregates candeteriorate during movement, detached smaller particles canbe susceptible to further transport.Factors Affecting Wind ErosionesurfacesurfaHeight (inches)ceUnlike many other areas of the U.S., soil loss from winderosion is greater than soil loss from water erosion in thenorthern Great Plains. This is due to the semi-arid climateresulting in less vegetative cover and drier soils that areless cohesive and more easily carried away by wind. Otherfactors affectingwind erosion are70wind characteristics60(e.g., velocity and50ddirection), surfaceateteconditions, soil40Vegproperties, field30length, and some20cultivation practices.r10Wind velocity isBathe most important0wind factor affecting05101520Velocity (feet/second)erosion; higher windvelocity equatesFigure 2. Change in wind velocitywith height over a vegetatedto higher windsurface and a bare surface. Theenergy and erosivity.two dashed lines denote velocitywithin and above a vegetated surface, However, not all windrespectively. (Troeh et al., 1999)will cause erosion. The minimum threshold velocity forwind erosion to occur is between 8 and 30 miles per hour,depending upon soil and surface conditions (Troeh et al.,1999). Velocity is lowest near the ground due to surfaceroughness, and increases sharply with height. Thus, windvelocity over smooth soils is greater and more erosivethan over rough soils because fewer ‘obstacles’ slow windvelocity at the surface. Figure 2 shows wind velocityprofiles above a bare surface and a vegetated surface.Wind velocities are substantially lower above the vegetatedsurface for a given height due to the vegetation absorbingsome of the wind’s energy and essentially raising the‘aerodynamic’ surface to a height of approximately 70%of the plant height, causing little or no wind to occurat the soil surface (Troeh et al., 1999). Other elementscontributing to soil roughness are aggregates and ridges.Soil properties that affect erodibility include texture,moisture and aggregation. Silt and fine sand particlesare most prone to erosion due to their smaller mass thanlarger particles, and less cohesiveness between particlesthan fine, clay-like particles (Morgan, 1995). Moistureincreases cohesive forces between particles, making themmore difficult to dislodge. Aggregation reduces erosion bybinding potentially erodible particles together into largerparticles which resist detachment and transport. Aggregatestability (how well the aggregate is bound) will also affect itserodibility and is related to chemical and organic compoundsin the soil (NM 8, SW 1). Soils with 2% or greater SOMcontent are less erodible than soils with less SOM due togreater aggregate stabilization (Morgan, 1995). Field lengthand size also play a role in the amount of soil eroded. Large,continuous fields allow more material to become detachedand transported than fields that incorporate obstaclesto impede wind speed and trap moving particles. Suchobstacles may include shelterbelts, cover crops, surfaceresidue and strip farming, and will be discussed further in themanagement section.Lastly, tillage can influence wind erosion bydisturbing the soil surface, causing dislodged particlesto be more susceptible to transport by wind (Figure3). Wind erosion during tillage is most severe when soilmoisture is low, fine textured soils predominate, and fewnonerodible materials are present. In addition, tilling onsteep slopes may result in considerable soil losses.Module 3 · Managing for Soil Erosion3
Figure 3. Direct effect of tillage on wind erosion in centralMontana.Water ErosionMechanicsMechanics of water erosion are often a two-fold process.Raindrops falling on the soil surface can cause particlesto detach and splash upward (Figure 4). Upon returningto the soil, splashed particles disperse and clog soil pores,causing surface crusting and a reduction in the soil’sinfiltration rate. The pounding action of rain may alsocompact the soil, further decreasing infiltration. Whenwater is applied in excess of the soil’s infiltration rate,water will puddle and run off, leading to additionaldetachment and transport of particles by the force offlowing water. Particle transport by water requires acritical speed to effectively carry sediment; when watervelocity slows below this speed, deposition occurs.Figure 4. A magnified view of a raindrop hitting a soil surface.The raindrop impacts the soil with enough force that fine particlesare detached and splashed upward. (Photograph from Finkel, 1986)4Module 3 · Managing for Soil ErosionBecause coarse particles fall outof suspension sooner than fineparticles as water velocity slows,they are more apt to remain onthe field while fine particles aremoved farther downstream.Three main forms of watererosion are sheet, rill and gullyerosion. Sheet erosion is theremoval of a thin layer of soilfrom the surface and is causedby ‘overland’ flow movinguniformly across the surface.As the sheet erosion continues,water begins to concentrate in small channels, or rills, andrill erosion occurs (Figure 5). Rills tend to be uniformlydistributed over the field and are defined as being smallenough to be smoothed over by cultivation practices. Theconcentration of running water causes rill erosion to bemore erosive than sheet erosion. Gully erosion occurswhen larger quantities of runoff concentrate and createlarge channels in the landscape. Gullies are relativelypermanent features that cannot be removed by tillage.Factors Affecting Water ErosionWater erosion is affected by precipitation patterns, soilproperties, slope and vegetative cover. Although watercauses less overall soil erosion in the northern GreatPlains than wind, one large rain event can cause severeerosion to occur. Intensity, duration and frequency of rainevents all appear to play a role in the amount of soil thaterodes. In general, the most severe erosion occurs whenrains are of relatively short duration, but high intensity.Heavy raindrop action coupled with more water fallingthan the soil can infiltrate can lead to high surface runoffand large losses of soil. Long, low intensity storms canalso be highly erosive due to saturated soil conditionscausing increased runoff (Morgan, 1995).Soil properties affecting water erosion include thosethat influence infiltration and soil stability, such as texture,organic matter, aggregation, soil structure and tilth. Theseproperties and their effect on infiltration were discussedin SW 1. Runoff is influenced by the amount and velocityof the flow, which in turn, is dependent on the slopeof the land. Because fast moving water can carry more
sediment than slow moving water,there is a greater potential to losea larger amount of material onsteep slopes than gradual slopes.Similar to its effect on winderosion, vegetative cover reducesdetachment by interceptingraindrops and dissipating theirenergy. In addition, surfacevegetation and residue act asdams that slow water flow andpromote deposition.Estimating ErosionEstimating soil erosion is importantfor determining erosion severityFigure 5. Sheet and rill erosion on a bare field. (Photograph by L. Betts, USDA NaturalResources Conservation Service)and its influence on land use andmanagement plans. For instance,susceptibility to erosion, and prevent particle transport.four of the factors affecting the NRCS phosphorus index, andUsing BMPs that focus on these key points will be mosthence manure application rates (NM 13), are related to soileffective for managing erosion. The following gives anerosion. Erosion is often best estimated with models, due tooverview of a number of BMPs used to manage erosion inthe number of variables involved. The two most commonlythe northern Great Plains.used models for the western U.S. are the Revised UniversalResidue ManagementSoil Loss Equation (RUSLE), used to estimate water erosion,One of the most valuable tools for managing erosionand the Wind Erosion Equation (WEQ). Both RUSLE andinvolves the use of plant residues. Residue refers to anyWEQ are defined by equations where a number of factorstype of vegetative cover left remaining on the field andaffecting erosion are taken into account to determine averagemay include standing stubble, dispersed straw, livingannual erosion rates. Factors include climate, soil erodibility,vegetation or mulch. Practices that maintain residuesurface roughness, length of field, vegetative cover, and inon the surface are less susceptible to soil erosion thansome scenarios, an erosion control practice. Because somepractices that remove excess residue. Figure 6 shows theamount of erosion is inherent regardless of land use andreduction in soil lost via erosional processes as the amountmanagement, the Soil Loss Tolerable Value, or ‘T value,’ wasof residue cover increases. For example, a field with 20%developed to create a base line to compare managementcover will have a 50% reduction in soil loss compared toinduced soil erosion. The T value is the amount of soil lossa bare field. This effect of residue cover on reducing soilin tons per acre that is acceptable to maintain long-termloss occurs for a variety of reasons. As previously discussed,productivity. T values vary by soil type and are dependentsurface residue absorbs some of the energy of wind,upon soil formation factors such as climate, geography,falling raindrops and running water, so that less energyand the time required for new soil to form. For help inis directed toward the soil. Intact root systems furtherdetermining values for soil loss variables and T values for astabilize soil particles. Soil moisture is conserved underparticular area, contact your local NRCS office.residue management due to increased infiltration anddecreased evaporation as a result of less wind and moreManaging Erosioncanopy shading. Additionally, standing residue duringKey points for managing any type of erosion are to reducewinter months will capture and retain snow better thanthe erosivity of the eroding agent, decrease the soil’sModule 3 · Managing for Soil Erosion5
Stubble Height6040200020406080100Residue Cover (percent)Figure 6. Percent soil loss reduction increases withincreasing residue cover. (From Dickey et al., 1986)a bare field, leading to greater moisture retention andsoil protection. Types of residue management and theirimpact on soil erosion are discussed below.TillageA large contributor to soil erosion in the northern GreatPlains is excessive tillage on croplands. ‘Conventionaltillage,’ in which less than 15% residue cover remains(Fawcett and Towery, 2003), buries surface residues,leaving agricultural soils unprotected and exposed toerosional processes. In contrast, conservation tillage leavesmore than 30% of crop residue on the surface, whichprovides coverage and protection of the soil surface.Varying types of conservation tillage are reduced till, ridgetill, mulch till, strip till and no till. Many of these werecovered in NM 12 and NM 15.In addition to providing soil coverage, conservationtillage often leads to an improvement in soil structurebecause of reduced mechanical disturbance (Magdoffand Weil, 2004), and an increase in SOM content andaggregation. For example, Schillinger (2001) found surfaceclod mass to be, on average, twice as great in minimumtillage (3-5 tillage treatments) than in conventional tillage(8 tillage treatments). Results were attributed to less cloddestruction by tillage equipment and greater residue coverin the minimum till plots.6Module 3 · Managing for Soil Erosion 80As previously mentioned, standing residue will reduce windspeed more than residue laying flat on the ground, and isan important component of residue management. Tallerstubble decreases wind velocity at the surface, providesmore cover and improves microsite growing conditions,all of which can positively affect crop yields. Results froma northern Great Plains study found that stubble heightduring the growing season significantly affected springwheat grain yield and water use efficiency (WUE) (Cutforthand McConkey, 1997; Figure 7). Tall stubble (averageheight 15 inches) increased yield by 12% compared tocultivated (disk tilled) stubble, while yield in the shortstubble treatment (average height 6 inches) was intermediatebetween treatments. A similar trend was observed for WUE,which was significantly higher in the tall stubble treatmentscompared to the cultivated stubble. Short stubble treatmentWUE was intermediate but not significantly differentthan WUE in the other treatments. Both yield and WUEresults were attributed to favorable microclimate growingconditions provided by the stubble, such as lower surfacetemperatures and reduced evapotranspiration losses dueto decreased wind velocity on the surface. Based on theconclusions of this study, keeping stubble height as tallas practical may help maximize yield and water use anddecrease erosion in conservation tillage systems. Soil Loss Reduction (percent)100 Figure 7. Effect of cultivated, short, and tall stubble on springwheat grain yield (yellow bars) and water use efficiency (blueline). (Cutforth and McConkey, 1997)Cropping SystemsCrop-fallow systems have been a common practice in thenorthern Great Plains for replenishment of soil water andweed control. However, erosion problems have increased in
many fallow systems due to the lack of cover for extendedperiods. Flexible or annual cropping decreases soil erosionby reducing or eliminating a fallow period, thus providingvegetative cover for longer periods during the year. Anotheralternative to crop-fallow is to grow a cover crop duringfallow periods to provide soil protection and, in the longterm, increase water infiltration and reduce runoff. Flexiblecropping and cover crops are detailed in NM 15.Strip farming, the practice of growing crops instrips with fallow in-between, can decrease erosion byreducing wind speed and breaking up the field length,which reduces particle detachment by controlling soilavalanching (Figure 8). To control wind erosion, stripsshould be planted in straight lines and perpendicularto the prevailing wind. In most areas of Montana andWyoming, this means establishing strips in a north-southdirection since winds are most commonly from the west.Strip width will vary with climate, field conditions andmachinery, and can be established with the aid of WEQmodels.Residue BurningA long-standing practice for managing excess residue hasbeen to burn it. Burning allows a producer to reduceexcess residue to ease seeding and plowing, partiallycontrol plant diseases, weeds and insects, and increaseshort-term nutrient availability. However, it is believedthat repeated, long-term residue burning ( 15 years) maycause soil erosion to increase over time due to a reductionin SOM content and overall soil quality (Fasching, 2001).Figure 8. Strip farming in northern Montana. Strips are plantedin a north-south direction to reduce wind erosion. Light strips are thisyear’s stubble and dark strips are last year’s stubble.Figure 9. Example of line-transect method in a wheat residuefield.Estimating Percent Residue CoverEstimating the percentage of residue remaining on a field canhelp a producer establish a baseline value of percent residuecover and track changes with time and residue managementpractices. The line-transect method is one of the easiest andmost accurate methods to use. This method consists of layinga marked tape or rope across a representative section of thefield and counting the number of marked units (inches,feet or meters) that are directly over residue. The numberof units where residue is present, relative to the number oftotal units, results in the percentage of residue cover. For theexample shown in Figure 9, one could observe the presenceof residue at every 1 foot increment (e.g., 12”, 24”). In thiscase, 24” would be counted as a residue point, but not 12”.Based off just these two points, percent cover would be 50%.However, if every inch was counted, percent cover would becloser to 70% using the line-transect method. Because of thesmall sampling area depicted in Figure 9, neither of thesemeasurements is accurate for a large field. A measuring lengthof 100 feet is most commonly used as it is easy to count footincrements and covers enough area to be fairly representative,although other lengths and increments can be used dependingon field conditions. To increase accuracy, the measuringprocess should be repeated in three or more other areas of thesame field and averaged to obtain a general percentage for thewhole field (Shelton et al., 1998). Sampling numerous pointsover a longer distance should smooth out field heterogeneity.Other methods for estimating cover are direct observation andphotography. Although these methods can be useful when aquick approximation of cover is needed, caution should beused in utilizing them as they often under- or overestimatecoverage. See the appendix for more information on estimatingpercent residue cover.Module 3 · Managing for Soil Erosion7
Figure 10. A shelterbelt of caragana in north central Montana.Erosion Control with VegetationShelterbeltsShelterbelts placed perpendicular to the prevailing winddirection can effectively control erosion by reducingwind velocity, trapping material and breaking up fieldlengths. Belts may be composed of grasses, shrubs, ortrees. Deciding which type and variety of plant to usewill depend upon climate and moisture factors, andnutrient and pest management. For the general climateof the northern Great Plains, perennial grasses, such astall wheatgrass (Elytrigia elongata), have proved successfulat reducing wind erosion, protecting vegetation, andtrapping snow (Aase and Pikul, 1995). Caragana(Caragana arborescens), a nitrogen-fixing shrub, is anothercommon shelterbelt plant for this region due to its winterhardiness and tolerance to drought (Figure 10). Please seethe appendix for a listing of other appropriate shelterbeltplants.Vegetative Filter StripsOff-site damage from water erosion can be managedthrough the use of vegetative filter strips (VFS). Discussedin NM 12, these strips, or buffer zones, are widths ofvegetation that reduce off-field transport and depositionof sediment and other pollutants by reducing waterflow velocity and trapping sediment. Placed at thebottom of a field or just above the tail water ditch in anirrigated system, VFS have been shown to be effective for8Module 3 · Managing for Soil Erosionreducing sedimentation inrunoff water. For instance, aMontana study found thatVFS reduced sedimentationin runoff water by 7580% compared to barefallow fields (Fasching andBauder, 2001). In general,sedimentation in runoffdecreases as VFS widthincreases. The optimumwidth depends upon fieldconditions such as slopeand length, and the amountof water moving through the system; steeper slopesand larger fields draining greater amounts of water willrequire wider strips. A minimum width of 10 feet isrecommended for flat fields ( 1% slope) with a 30:1drainage area to filter strip ratio (USDA-NRCS, 1988).In addition, VFS must also be able to have enoughvegetative growth to function during the first large rain orirrigation event.To ensure adequate growth, fall may be the best time toplant a VFS.Tillage ManagementThere may be times that some limited tillage can bebeneficial in controlling erosion. For instance, a one-timetillage pass can cause aggregates and clods from belowthe surface to be turned upward, temporarily increasingsurface roughness. This type of tillage works best if thesoil is of fine to medium texture and there is adequate soilmoisture (Fenster and Gaddis, 1983). If the soil is toocoarse or dry though, tillage can exacerbate detachment.To prevent further soil loss, tillage should be avoidedduring windy periods and on highly erosive slopes.Erosion Control in Irrigated SystemsIrrigation-induced erosion can be managed by improvingirrigation practices, compacting furrows, keeping cropresidues in the furrow or using amendments. Twoamendments used to reduce erosion in irrigated systemsare gypsum and polyacrylimide (PAM). Discussed inSW 2, gypsum (CaSO4·H2O) can help flocculate (clump
Q & A #1Is PAM used in dryland systemsfor erosion control?Figure 11. The effects of PAM-treated soil water and nontreated soil water. (Photograph from NWIRSL, Kimberly, ID)together) soil particles and increase infiltration, therebyreducing surface runoff and sediment loss. PAM is awater soluble, organic substance that aggregates soil andincreases infiltration at low concentrations. It also actsas a flocculating agent that, when in a solution, causessuspended particles to draw together and settle (Figure11). In a PAM-treated system, this effect causes sedimentto fall out at the bottom of the furrow and not remainin the runoff water. The most common form of PAMused in agriculture is anionic (negatively charged), drypowder PAM. Anionic PAM has low solubility in water,making it effective for staying in place rather than beingdissolved and carried away. Dry powder can be appliedas a ‘patch’ (a 5-6 foot placement of powder on thefurrow ditch surface directly below the inflow point) ormixed in with irrigation water, which requires thoroughmixing and turbulence to distribute evenly. When usedin furrow irrigation systems, PAM has been found toreduce runoff soil loss by an average of 94% and increasewater infiltration by an average of 15%, when appliedat the NRCS recommended rate of 1 lb/ac or 10 partsper million (Lentz and Sojka, 1994). For sprinklerSome research has found PAM to work indryland/rainfed areas to reduce erosion.However, effectiveness varies. For instance,one study found that when dry granularPAM was mixed with the surface soil andsimulated rain was applied, soil loss fromtreated areas was reduced, but neither runoff or infiltration were affected (Yu et al.,2003). Thus, because of its rather low solubility and the water, labor and equipmentcosts associated with its application, PAMis likely not an economical method for erosion control on most dryland operations inMontana and Wyoming.systems, liquid PAM can be used at a rate of 2 to 4lb/ac (Bjorneberg, pers. comm.). PAM requires certainapplication and handling procedures to protect humanand environmental health, and PAM products shouldbe purchased according to the registration and labelingrequirements of your state.Managing Erosion on Grazed LandsThe main factors influencing erosion on grazed landsare type of grazing systems, stocking rate, foragetype and growth patterns, and soil characteristics.Overgrazing leaves little vegetative cover to protect thesoil from erosion (Figure 12), and heavy animal trafficcan compound the problem by compacting the soil.Rotational grazing systems may prevent overgrazing andleave vegetation intact better than continuous grazingsystems (NM 15). Forages with extensive root systemswill help stabilize soil and improve soil structure. Finetextured soils are most susceptible to compaction andshould be monitored on a yearly basis to assess infiltration(SW 4). Amendments and cultivation practices may beable to alleviate compaction in these areas.Module 3 · Managing for Soil Erosion9
Figure 12. Comparison of the effect of grazing on vegetation.Land right of fence has been grazed and land left of the fence has not.Conservation Compliance andErosion Control ProgramsA number of erosion control incentives and programshave been introduced in recent years to control erosion onhig
natural land features we observe today, such as the Grand Canyon, are the result of erosion. However, human alterations of land use and cover have caused erosion rates to increase for many areas of the world, resulting in considerable land and environmental degradation. An average of 1.3 billion tons