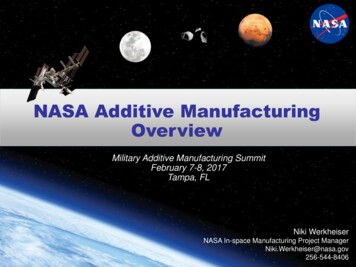
Transcription
NASA Additive ManufacturingOverviewMilitary Additive Manufacturing SummitFebruary 7-8, 2017Tampa, FLNiki WerkheiserNASA In-space Manufacturing Project ManagerNiki.Werkheiser@nasa.gov256-544-84061
Agenda NASA’s Journey to Mars – Where will Additive Manufacturing Contribute? In Space Manufacturing Initiative (ISM)– 3D Printer International Space Station Technology Demonstration InitialResults– ISM Elements– Additive Construction with Mobile Emplacement (ACME) and AdditiveConstruction for Expeditionary Structures (ACES)– External In-Space Manufacturing and Assembly Projects– ISM Roadmap Additive Manufacturing of Liquid Rocket Engine Components– Additive Manufacturing Demonstrator: Liquid Propulsion System– Proposed Engineering and Quality Standard for Additively ManufacturedSpaceflight Hardware– Additive Manufacturing Structural Integrity Initiative (AMSII) Summary2
Additive Manufacturing Path to ExplorationEARTH RELIANTPROVING GROUNDEARTH INDEPENDENTEarth-Based Platform Certification & Inspection ProcessDesign Properties DatabaseAdditive Manufacturing AutomationIn-space Recycling TechnologyDevelopmentInternational External In-spaceSpace StationManufacturing and Repair AM Rocket EngineDevelopment, Test,and Certification AM for Support Systems (e.g., ECLSS)Design, Development, TestAsteroidsSpace LaunchSystemSpace-Based PlatformCommercialCargo and Crew 3D Print Tech Demo Additive ManufacturingFacility On-demand Parts Catalogue Recycling Demo Printable Electronics Demo In-space Metals Demo AM Propulsion Systems- RS-25- Upper Stage Engine Habitat SystemsPlanetary Surfaces Platform Additive ConstructionTechnologies Regolith Materials - Feedstock AM In Space Propulsion Systems- Upper Stage- Orbiters- Landers Habitat Systems3
Additive Manufacturingat Marshall Space Flight CenterIn Space Manufacturing Initiative4
3D Printer International Space Station (ISS)Technology Demonstration - Preliminary ResultsMechanical PropertyTest Articles A total of 21 parts were printed on ISS, including the uplinked ratchet handle.Inspection and testing of all articles included:Structured light scanningsDensity Functional ToolsX-ray and CT scanMechanical testingMicroscopyMechanical property differences observed between flight and ground samplesAdditional ISS prints in Spring 2016 will enable additional mechanicalproperties data and support hypotheses evaluationLessons Learned have been incorporated into the next generation 3D Printerfor ISS – Additive Manufacturing Facility (AMF) by Made In Space5
In-Space Manufacturing ElementsMaterial Characterization Database DevelopmentCompression Testing ofMechanical Flight Sample 7/21/15 Objectives: Characterize and document any microgravityeffects on printed parts and resulting mechanical properties Develop design-level database for microgravity applications Additional on-orbit prints of engineering test articles areplanned with ISS (3D Printer and AMF) All datasets will be available through the MSFC Materials andProcesses Technical Information System (MAPTIS)OGS AAAInlet AdaptorOn-demand ISM Utilization Catalogue Development Objective: Develop a catalogue of approved parts for in-spacemanufacturing and utilization Joint effort between MSFC AM M&P experts, space systemdesigners, and JSC ISS Crew Tools Office Documenting on-orbit printing process with users and ISSProgram (safety, human factors, etc.) Developing V&V/Quality Control/Certification process andprocess for Candidate Part inclusion in catalogueFreedom360VirtualRealityRig6
In-Space Manufacturing ElementsAMF - Additive Manufacturing Facility (SBIR Phase II-Enhancement) withMade In Space Commercial printer for use on ISS Incorporates lessons learned from 3D Printer ISS Tech Demo Expanded materials capabilities: ABS, ULTEM, PEEK Anticipated launch in Spring 2016AdditiveManufacturingFacilityIn-space Recycler ISS Tech Demonstration Development (SBIR 2014) Objective: Recycle 3D printed parts into feedstock to help close logistics loop Phase I recycler developments completed by Made In Space and TethersUnlimited Phase II SBIR (2014) awarded to Tethers Unlimited for the In-space Recyclerfor proposed ISS Technology Demonstration in FY2017Launch Packaging Recycling Phase I SBIR (2015) Objective: Recycle launch packaging materials into feedstock to help closelogistics loop (3 proposals selected for award)Tethers Unlimited SBIR toDevelop ISS Recycler Tech DemoIn-space Printable Electronics Technology Development Collaborating with Xerox Palo Alto Research Center (PARC), NASA AmesResearch Center, and AMRDEC Roadmap developed targeting ISS technology demonstration Printing a Radio Frequency Identification (RFID) antenna for assessment on theRFID Enabled Autonomous Logistics Management Tech Demo Additive ultracapacitors have been developed, tested, & patented utilizingMSFC Innovation Funds3D Printed RFID Antenna, layersPrinted/packagedultracapdevice7
Collaborative Additive Construction ProjectsAdditiveConstruction withMobile Emplacement(ACME)Shared Vision: Capability to print custom-designedexpeditionary structures on-demand, in the field,using locally available materials.AutomatedConstruction ofExpeditionaryStructures (ACES)8
Collaborative Additive Construction Projects StatusAdditive Construction with Mobile Emplacement (ACME)PlanetaryCandidate BinderRegolith-basedMaterialsConcrete Sorel-type cement(Mg0-based) Sulfur cement Polymers / trash Portland cementManual feedGantryMaterialsPortlandCementDry GoodFeedLiquidStorageStorageSubsystemsACME PlanetaryStructureAccumulatorContinuous Deliveryand Mixing SystemCOTSCOTS ConcreteMixerPump (not ntTrialsACES 2NozzleACME 3ACE3 3GuardShack(6’ x 6’ x8’)Automated Construction of Expeditionary Structures (ACES)Synergistic technologies for planetary and terrestrial use9
Technology Development forExternal In Space Manufacturing and AssemblySpace Technology Mission Directorate’s Tipping Point Projects – Robotic In-Space Manufacturing and Assembly ofSpacecraft and Space Structures Dragonfly: On-Orbit Robotic Installation and Reconfiguration of Large Solid RF ReflectorsSpace Systems Loral of Palo Alto, California– Public-Private Partnership for Robotic In-Space Manufacturing and Assembly of Spacecraft and Space StructuresOrbital ATK of Dulles, Virginia– Project provides the next generation of performance advancements in GEO ComSats: more apertures for greatergeographic coverage variation, reconfigurable apertures for mission/fleet versatility, larger apertures for greaterthroughput, and mission enabling unique optics.Project will perform an integrated ground demonstration including robotically deployed rigid backbone and weldingusing precision alignment.Versatile In-Space Robotic Precision Manufacturing and Assembly System - Made in Space, Inc. of Moffett Field, CaliforniaArchinaut: In-Space Manufacturing & AssemblyArchinaut enables autonomous manufacturing and assembly of spacecraft systems on orbitFeedstock StoresEnable On OrbitManufacturingConfigurable as aFree Flyer or an IntegralSpacecraft SystemRobotic ManipulatorsIntegrate Functional Componentsand Install Assembled SystemsAdditive Manufacturing DeviceCreates Large, Complex StructuresExternal In-Space Manufacturing (EISM) is a joint program between the Defense Advanced Research ProjectsAgency (DARPA) Tactical Technology Office and the NASA Advanced Exploration Systems (AES) Office– Broad Area Announcement solicitation to be released in Spring 201610
In-space Manufacturing Exploration TechnologyDevelopment RoadmapEarth-basedDemos: Ground & ISSExplorationMarsAsteroidsPlastic PrintingDemoMat.Char.3D Print Tech DemoGround Analogs Multiple FDMZero-Gparabolic flights(1999-2013) System Studies& ground Testsfor MultipleMaterials &Technologies Verification &Cert. Processdevelopment Material &PrinterCharacterizationDatabase AutonomousProcess Dev. AdditiveConstruction:Simulant Dev.&GroundDemos2014 In-space:3DPrint: FirstPlastic Printeron ISS TechDemo NIAC ContourCrafting NIAC PrintableSpacecraft Small Sat in aDay AF/NASASpace-basedAdditive NRCStudy ISRU Phase IISBIRs Ionic Liquids 015 - 2017 3D Print DemoABS Ops Add. Mfctr.Facility UltemOps (AMF) In-space UtilizationCatalogue PartCert & Testing RecyclerDemo NASA/DARPAExternal Inspace BAADemo In-spaceMaterialDatabase FutureEngineerSTEMChallenge(s)Metal PrintingFab Lab Self-repair/replicateDigital External InMfctr. space Mfctr2018 - 2024ISS: “Fab Lab”Utilization/FacilityFocus In-space RecyclerDemo Integrated FacilitySystems forstronger types ofextrusion materialsfor multiple usesincluding metals &various plastics EmbeddedElectronics TechDemo Synthetic BiologyDemo Metal DemoOptions ACME GroundDemosLunarLagrangePoint2025-352035 Lunar, LagrangePlanetaryMars Multi-MaterialFabLabsSurfacesFab Lab Initial Provision & UtilizePoints FabRobotic/Remotein situ resources TransportMissionsfor feedstockvehicle and FabLab: Provides Provisionsites wouldon-demandfeedstockneed Fabmanufacturing of Evolve to utilizing capabilitystructures,in situ materials Additive(naturalConstruction electronics, & partsutilizing in-situ andresources,& Repair ofex-situ (renewable)synthetic biology) largeresources. Includes Product: Ability to structuresability to inspect,produce, repair,recycle/reclaim, andand recycle partspost-process as& structures onneededdemand; i.e.autonomously to“living off theultimately provideland”self-sustainment at Autonomous finalremote destinations.milling tospecification* Green text indicates ISM/ISRU collaborationISS Serves as a Key Exploration Test-bed for the Required Technology Maturation & Demonstrations11
Additive Manufacturingat Marshall Space Flight CenterAdvanced Manufacturing Demonstrator:Liquid Propulsion System12
Additive manufacturing relatedTransforming Liquid Propulsion Systems DDT&E with AMProject Objectives Reduce the cost and schedule required for newengine development and demonstrate it through acomplete development cycle.– Prototype an engine in less than 2.5 years.– Use additive manufacturing to reduce part cost,fabrication time, and overall part count.– Adopt Lean Development approach. Focus on fundamental/quick turn analysis to reducelabor time and cost and move to first developmentunit Get hardware into test fast so that test data can beused to influence/refine the design Advance the TRL of additive manufactured partsthrough component and engine testing.Develop a cost-effective prototype engine whosebasic design can be used as the first developmentunit for an in-space propulsion class engine.13
Strategic Vision for Future AM Engine SystemsBuilding Experience“Smart Buyer” to enableCommercial PartnersDefining the DevelopmentPhilosophy of the Future Integrating Design withManufacturing3D Design Models andSimulations IncreaseProducibilityTransforming Manual toAutomated ManufacturingDramatic Reduction inDesign Development, Testand Evaluation (DDT&E)CyclesBuilding FoundationalIndustrial BaseBridging the gapbetween the presentand future projects thatare comingEnabling & DevelopingRevolutionary TechnologyTransferring “Open Rights”SLM Material Property Data& Technology to U.S.Industry14
Game-Changing Aspects of Prototype Additive EngineState of the Art forTypical Engine Developments DDT&E TimePrototype Additive Engine – 7-10 years Hardware Lead Times– 2-4 years – 3-6 Years TestingEngine Cost Applicability– Design for particular mission bya particular contractor– Often proprietaryTesting– Testing occurs early in the DDT&E cycle – 20 - 50 Million Hardware Lead Times– 6 Months– Late in the DDT&E cycle DDT&E TimePrototype Cost– 3-5 Million Applicability– Provide relevant data to multiplecustomers (SLS, Commercial partners,other government agencies)– Flexible test bed configuration canaccommodate other’s hardware / designconcepts15
Reduction in Parts Count for Major HardwareMOVThrust StructurePart Count (Approx): 1 vs. 6MFV (Hidden)InjectorPart Count (Approx): 1 vs. 5Part Count (Approx): 6 vs. 255FTPMixer (Hidden)Part Count: 2 vs. 8Part Count (Approx): 22 vs. 40OTPMCCPart Count (Approx):41 vs. 80OTBVCCV(Hidden)Part Count (Approx): 1 vs. 5Note: Part counts examples arefor major piece parts and do notinclude bolts, nuts, washers, etcPart Count (Approx): 1 vs. 5TurbineDischargeDuctRegen Nozzle16
Future OutlookFundamental Additive Manufacturing M&P DevelopmentPushMaterial Properties& NDEStandards & SpecsCertificationRationaleMethane Prop.SystemsPullLean & Aggressive Development PhilosophyPayloads & SatellitesParallel & Congruent ActivitiesRP EngineNuclear PropulsionMPSComponentsRelevant Environment TestingUpper Stage EngineLPS Prototype EngineRS-25CCPBuilding Foundational Additive Manufacturing Industrial Base17
Additive Manufacturingat Marshall Space Flight CenterProposed Certification Approach for AdditivelyManufactured Rocket Engine Spaceflight Hardware18
AM in the Human Exploration and Operations PortfolioExploration Systems DevelopmentORION and SLSCommercial Crew ProgramDRAGON V2Requirement choices dictate how we embrace, foster,and protect the technology and its opportunities19
Managing Opportunity and Risk Opportunity– Additive Manufacturing (AM) offers revolutionary opportunities inmechanical design innovation, cost savings, and schedulereductionRisks– Lack of governing requirements (M&P)– Process control – how to ensure AM process is repeatable,reliable, and in control (M&P, PM)– Process sensitivity :: unknown failure modes (M&P, PM)– Inspection and acceptance testing (PM)– Rapidly evolving technology(M&P, PM)– Too easy, too cheap ubiquitous, lack of rigor (M&P)– AM related failure tarnishes the technology (M&P)M&P Materials and Processes EngineersPM Project Management20
Engineering and Quality Standard for AM SpaceflightHardware TailoringGoverning standardsAM DesignPart ClassificationStructural AssessmentFracture ControlQualification TestingPart Development PlansProcess ControlsMaterial PropertiesFinishing, Cleaning, RepairAllowancesPart Inspection and Acceptance21
Key Knowledge Gaps and Risks Available standards will not mitigate AM part risk to a level equivalent to otherprocesses for some time to come! Known Unknowns needing investment:– Unknown failure modes :: limited process history– Open loop process, needs closure or meaningful feedback– Feedstock specifications and controls– Thermal processing– Process parameter sensitivity– Mechanical properties– Part Cleaning– Welding of AM materials– AM Surface improvement strategies– NDE of complex AM parts– Electronic model data controls– Equipment faults, modes of failure– Machine calibration / maintenance– Vendor quality approvalsKnowledge gaps exist in the basic understanding of AM Materials and Processes,creating potential for risk to certification of critical AM Hardware.22
Additive Manufacturing Structural Integrity Initiative(AMSII) Project Overview Goal for Certification of AM flight hardware at NASA– Implement a unified, data-driven, cross-program approach to the certification of structural AMspaceflight hardware. AMSII Project Objectives– Provide the foundational knowledge required to qualify the Selective Laser Melting (SLM)process for structural hardware in spaceflight applications.– Validate the qualification and certification methodology presented in the MSFC draft, Engineeringand Quality Standard for Additively Manufactured Spaceflight Hardware. Participants– ARC, GRC, LaRC, MSFC, NASA NDE Working Group (NNWG) Tasks1. Establish feedstock controls and maximum recycle limits for Alloy 718 powder. Identify powdercontrol metrics for inclusion in standard.2. Apply statistical process controls to powder fusion systems. Evaluate methodology atcommercial vendors having mature SLM alloy 718 processes3. Derive statistically-based design allowables for SLM alloy 718 and compare with traditional Abasis methodology. Publish design allowables for SLM alloy 718 based on a minimum fivepowder lots.4. Design and build analogue engine articles to evaluate platform-to-platform variability; establish“first article” inspection methodology; and interrogate critical features.5. Characterize defect structures of SLM alloy 718 material built within and at the limits of theprocess window. Provide test articles to NNWG for NDE studies. Evaluate in-situ processmonitoring methods to detect defects and/or build anomalies.23
In-Space Manufacturing Summary In-space manufacturing is a critical capability needed to support NASA’sdeep space exploration missions– Increase in reliability– Reduction in logistics burden (make it or take it)– Recycling capabilities– Flexibility in design NASA has taken the first step towards in-space manufacturing capabilityby successfully demonstrating 3D print technology on ISS The journey through development and proving ground trials is a long one– Foundational technologies are yet to be demonstrated– Design for repair culture needs to be embraced– Applications need to be validated Additive construction offers significant potential for expeditionarystructures for the Army and NASA and, in addition, commercial,humanitarian assistance and disaster relief possibilities. Excellentopportunity for demonstration of public/private partnerships.24
Additive Manufacturing of Rocket Enginesfor Human Space Exploration Summary AMD-LPS is catalyst for culture change– Demonstrated game changing aspects of cost and schedulereduction– Dramatic reduction in Design, Development, Test and Evaluation(DDT&E) cycle time– Established technology testbed for future developmentsCertification approach for additively manufactured rocket enginecomponents developed by MSFC– Center-level AM requirements released for broad review in July 2015– Requirements allow innovation while managing risk– Defines the expectations for engineering and quality control indeveloping critical AM partsAdditive Manufacturing Structural Integrity Initiative (AMSII) is anAgency level cooperative effort designed to address foundationalknowledge gaps in certification requirements to better manage AMrisk25
NASA In-space Manufacturing Project Manager Niki.Werkheiser@nasa.gov 256-544-8406 NASA Additive Manufacturing . RFID Enabled Autonomous Logistics Management Tech Demo Additive ultracapacitors have been developed, tested, & patented utilizing . Industry Integrating .