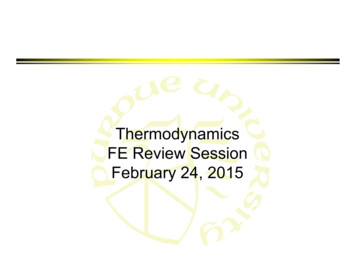
Transcription
ThermodynamicsFE Review SessionFebruary 24, 2015
What is Thermodynamics? Application of three governing principles to individualcomponents or systems under consideration» Mass Balance» Energy Balance» Entropy Balance
Types of Systems Isolated systems have neither mass nor energy interactionswith surroundings Closed systems have energy interactions (heat transfer andwork) but no mass interactions Open systems have energy interactions (heat transfer andwork) as well as mass interactions
Property and State Property is a particular characteristic of a given system» Extensive properties are dependent on the amount ofmass in the system (m, V, U, etc.)» Intensive properties are not dependent on the amount ofmass in the system (P, T, etc.)» Specific properties are extensive properties per unitmass and indicated by lower case letters (v V/m, u U/m, etc.)Divide the system into smaller parts todetermine extensive or intensive State is defined using a set of properties State Principle: Need values of two, independent, intensiveproperties to fix state for simple compressible substance
Some Properties Absolute Pressure P (lbf/in2 or Pa) Absolute Temperature T (R or K) Specific Volume v (ft3/lbm or m3/kg) Specific Internal Energy u (Btu/lbm or kJ/kg) Specific Enthalpy h u Pv (Btu/lbm or kJ/kg) Specific Entropy s (Btu/lbm-R or kJ/kg-K) Specific Heat at Constant Pressure Cp (Btu/lbm-R or kJ/kg-K) Specific Heat at Constant Volume Cv (Btu/lbm-R or kJ/kg-K)
Energy Energy consists of the microscopic internal energy (U) andthe macroscopic mechanical energy (KE and PE) Internal energy is related to microscopic kinetic energy(translation, vibration, rotation, electronic motions) pluschemical and nuclear energy Mechanical energy is related to bulk velocity and elevation isa gravitational fieldE U KE PE
Entropy Level of disorganization (disorder) in the systemEntropy is related to disorder(randomness) Q dS T Thermal energy is disorganized21internally reversible For isothermal, reversible heat transfer only (e.g. Carnotcycle or reversed Carnot cycle): Q T S Entropy change calculations for pure substances requireproperty tables while for solids or liquids or ideal gasessimple equations can be used
P-v and T-v DiagramsNotice orientation of lines of constant T (on P-v diagram) andlines of constant P (on T-v diagram)
Property Evaluation Table
Compressed Liquid When P Psat at given T or T Tsat at given P P and T are independent Property tables may/may not be available v (T, P) vf at T u (T, P) uf at T h (T, P) hf at T (vf at T)(P – Psat at T) s (T, P) sf at T C p Cv C u C T h u v P s C ln(T2/T1)Only absolute temperature (K or R)
Two Phase Mixture In two-phase mixture, liquid and vapor exist in equilibrium P and T are not independent Quality is defined as the mass fraction of vapor phasemx y y x y y m mv xv (1 x)v v xvu xu (1 x)u u xuh xh (1 x) h h xhs xs (1 x) s s xsgfgfggfgffggfffffgfffgfgfg
Superheated Vapor When P Psat at given T or T Tsat at given P P and T are independent No approximations; property tables must be used
Compressible (Real) Gas Use reduced pressure and reduced temperature to obtaincompressibility factor using chartReduced PressurePP PReduced TemperatureRCritical TT TRCriticalCompressibility factor is defined asPvZ RTIdeal gas (Z 1) when PR 0 at any TRor when TR 2 at small values of PRAir can usually be consideredideal at temperatures above 266 Kat moderate pressures because itscritical temperature is 133 K
Compressibility Factor Chart
Ideal Gas Ideal Gas Equation of State (Z 1) Applicable at low pressure and high temperature relative tocritical point i.e. low density conditionsPv RTPV mRT nRTAbsolute temperature (K or R)Absolute pressure not gage pressureRR Mft-lbfBtuR 1545 1.986lbmol-Rlbmol-RJ 8314kmol-K
Ideal Gas (contd.) For an ideal gas, internal energy and enthalpy depend onlyon the absolute temperature Use ideal gas tables to find property changes h h hP s s s R lnP u u u2 120021121 For constant specific heat assumption u C T T vAbsolute temperature (K or R) s C lnp T T21 R ln2 P P211 C lnv h C T T p T T212 R ln1 V V21
Special Processes Constant pressure (isobaric) P constant Constant volume (isochoric) V constant Constant temperature (isothermal) T constant No heat interaction (adiabatic) Q 0 Constant enthalpy (isenthalpic) h constant Constant entropy (isentropic) s constant Adiabatic and reversible (isentropic) s constant
Isentropic Process – Ideal Gas Process in which disorder (entropy)remains unchanged “ideal” processConstant Specific HeatsAbsolute temperature (K or R)T P T P 2211( k 1 ) / k v v k 112Variable Specific HeatsRelative Pressure (Not Reduced Pressure)pP pPr22r11Relative Volume (No Reduced Volume)v V v v V vr222r111Relative pressure and relative volumelisted in air ideal gas tables; function of T only
Open System Energy Balance Conservation of energy statement for control volumeh u Pv i.e. must consider flow work (Pv)dEVV Q W m h gz m h gz 22 dt22CVCVCVinletsexits Mass entering the system increases energy content whilemass leaving the system decreases energy content Use appropriate sign convention for heat and workinteractions» Heat transfer into system positive; heat transfer fromsystem negative» Work into system negative; work out of system positive
Open System Entropy Balance For an open system, entropy changes occur due to heattransfer at the boundary, entropy production owing toirreversibilities, and entropy transfer associated with massinflow and outflowMass leaving reduces disorderMass entering increases disorderQ dS m s m s S TdtCVCVinletsiiexitseegenCVDue to heat transfer at boundaryAbsolute temperature (K or R)Entropy generation due to irreversibilitieswithin the system
Closed System Energy Balance Special case with no inlets or exits (control mass)Q W E U PE KEWork Interaction(W)Heat Interaction(Q)Closed System(E U PE KE)No Mass Interaction(m constant) Use appropriate sign convention for heat and workinteractions» Heat transfer into system positive; heat transfer fromsystem negative» Work into system negative; work out of system positive
Closed System Entropy Balance For a closed system, entropy changes occur due to heattransfer at all relevant boundaries and entropy generationEntropy generation due to irreversibilitiesdue to irreversibilitieswithin the systemDue to heat transferat boundaryQ S STgenbAbsolute temperature (K or R)
Clausius Inequality For cycles ( S 0), we can write:Results from applying theentropy balance to a cycleQ Q S T T genb Absolute temperature (K or R)bIncrease of entropy principle requires that total change inentropy of the universe must always be positiveSgen ,total S Stotalsystem Ssurroundings Stotal must always be zero (ideal reversible process) or positive ( real irreversibleprocess); Ssystem and Ssurroundings can be positive, negative, or zero
Energy Interactions – Work For a reversible (quasi-equilibrium) process in open system:W VdP21W V P P V constant21Work is represented by the area underprocess curve on P-v diagram projectedon the pressure axisP constantW 0n PV PV W n 1PV n constant1122
Energy Interactions – Work (contd.) For an ideal gas inside open system: VW PV ln VT constant2111 P PV ln P 1112T constantPV PV1122 P k PV PV kW RT 1 k 1k 1 P Pv k constant1122211( k 1 ) / k
Energy Interactions – Work (contd.) For a reversible (quasi-equilibrium) process in closed system:W PdV2b1Work is represented by the area underprocess curve on P-v diagram projectedon the volume axisV constantW 0bW P V V P constantb21PV PVW n 1PV n constant1b122
Energy Interactions – Work (contd.) For an ideal gas inside closed system:W 0W P V V TT PPVV TTP constantV constantbbV constant1212P constant VW PV ln VT constant2b111T constantPV PV112221212 P PV ln P 11121
Energy Interactions – Heat Transfer Conduction and Fourier’s Law T» Conductive heat flux: q k xwhere,T1"condImportant mostly in solids since ksolid kliquid kgasxqcond”– qcond” Heat flux in the direction of temperaturegradient (W/m2)– k Thermal conductivity in the direction of heattransfer (W/m-K)– dT/dx Temperature gradient (K/m) T» Conductive heat transfer rate:qqAkA where, x– qcond Heat transfer rate due to conduction (W)– A Area normal to temperature gradient (m2)"condcondT2
Energy Interactions – Heat Transfer (contd.)Ts Convection and Newton’s Law of Cooling» Convective heat flux: q "convwhere, hconv T T u , T sImportant at solid-fluid (liquid or gas) interface– qconv” Heat flux at the surface (W/m2)– hconv Convection heat transfer coefficient (W/m2-K)– Ts and T Temperature of surface and fluid,respectively» Convective heat transfer rate:q q A h A T T"convconvsconvss where,– qconv Heat transfer rate due to convection (W)– As Area of surface (m2)
Energy Interactions – Heat Transfer (contd.) at high temperatures; no medium required unlikeRadiation Importantconduction/convection» Radiative heat flux: "44qrad Ts Tsurrwhere,– qrad” Net radiation heat flux (W/m2)– Emissivity of the given surface– Stefan-Boltzmann constant (W/m2-K4)– Ts and Tsurr Absolute temperature (K) of surface andsurrounding, respectively Only for a gray surface in a large» Radiative heat transfer rate: isothermal surrounding q q A A T T"44 where,– qrad Heat transfer rate due to radiation (W)– As Area of surface (m2)radradssssurr
Some SSSF Devices Nozzles and Diffusers»»»»Single inlet and single exitNo work interaction (passive devices)Usually adiabaticNo potential energy changeVVh h 2222iieeNozzle increase KE (velocity) with decrease in enthalpy (pressure)Diffuser increase enthalpy (pressure) with decrease in KE (velocity)
Some SSSF Devices (contd.) Turbines and Compressors»»»»Single inlet and single exitUsually adiabaticUsually no potential energy changeUsually no kinetic energy changew h hi ePumps handle incompressible fluids (liquids)w c T T v P P ieieTurbine decrease pressure (enthalpy) to produce work output (hi he)Compressor to increase pressure (enthalpy) require work input (hi he)
Some SSSF Devices (contd.) Throttling Valves»»»»»Single inlet and single exitNo work interaction (passive device)AdiabaticNo potential energy changeNo kinetic energy changeh hP PEnthalpy remains constantPressure drops from inlet to exitieeFor ideal gas, no change in temperature from inlet to exitFor an incompressible fluid, temperature increases from inlet to exitFor a two-phase mixture, temperature decreases from inlet to exiti
Some SSSF Devices (contd.) Heat Exchangers»»»»»»Multiple inlets and multiple exitsNo work interaction (passive device)Usually no heat transfer to/from surroundingsNo potential energy changeExit Fluid 1No kinetic energy changeFluid streams do not mixInlet Fluid 2Exit Fluid 2In the absence of stray (to outside) heat transfer:Heat gained by one fluid Heat lost by the other fluidInlet Fluid 1
Some SSSF Devices (contd.) Mixing Chambers»»»»»»Multiple inlets and multiple exitsNo work interaction (passive device)Usually no heat transfer to/from surroundingsNo potential energy changeNo kinetic energy changeFluid streams mix directly
Isentropic Efficiency – SSSF DevicesExit pressure of both isentropic and actual process is sameactualturbine1isentropicWactualWi sentropi c h h h hw w122sV V 2 h h V V 2 h h22nozzle2122s122112s
Isentropic Efficiency – SSSF Devices (contd.)Exit pressure of both isentropic and actual process is samehP22a 2sw wisentropiccompressoractualP1h h h h2s211V V 2 h h V V 2 h h211diffuser2s21s22s12221
Basic Cycles – Heat Engine Q Q CNet work output cyclethermalCWQ Q QQHreversible onlyHHT THCHHeat added T TT 1 TTHthermal ,CarnotreversibleCHCHAbsolute temperature (K or R) If thermal thermal, Carnot irreversible HE If thermal thermal, Carnot reversible HE If thermal thermal, Carnot impossible HE
Basic Cycles – RefrigeratorHeat removed fromcold spaceQQCOP WQ QCCRFcycleHCWork input requiredCOPRF ,CarnotreversibleT1 T T T T 1CHCHCAbsolute temperature (K or R) If COPRF COPRF, Carnot irreversible RF If COPRF COPRF, Carnot reversible RF If COPRF COPRF, Carnot impossible RF
Basic Cycles – Heat PumpHeat supplied to maintainhigh temperatureQQCOP WQ QHHHPcycleHCWork input requiredCOPHP ,CarnotreversibleT1 T T 1 T THHCCHAbsolute temperature (K or R) If COPHP COPHP, Carnot irreversible HP If COPHP COPHP, Carnot reversible HP If COPHP COPHP, Carnot impossible HP
Carnot (Most Ideal) Cycle Carnot power cycle has two isothermalprocesses (heat addition and heatrejection) and two isentropic processes(expansion and compression)TCarnot Power Cycle Reversed Carnot cycle is the basis forrefrigeration cycles such as vaporcompression cycle Power cycles always run clockwise onproperty diagrams and refrigerationcycles always run anti-clockwise For any cycle, net work interactionequals net heat interactionHeat addition at TH1243Heat rejection at TCs
Important Cycle Concepts Carnot cycle has maximum efficiency compared to anyother cycle For internally reversible cycles, heat transfer can be easilycalculated using T s for isothermal processes For ideal cycles (all internally reversible processes), areaunder the P-v diagram represents net work For ideal cycles (all internally reversible processes), areaunder the T-s diagram represents net heat
FE Review Session February 24, 2015. What is Thermodynamics? Application of three governing principles to individual components or systems under consideration » Mass Balance » Energy Balance » Entropy Balance. Types of Systems Isolated systems have neither mass nor energy interactions with surroundings Closed systems have energy interactions (heat transfer and work) but no mass