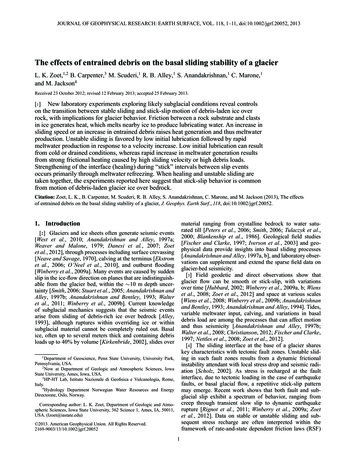
Transcription
JOURNAL OF GEOPHYSICAL RESEARCH: EARTH SURFACE, VOL. 118, 1–11, doi:10.1002/jgrf.20052, 2013The effects of entrained debris on the basal sliding stability of a glacierL. K. Zoet,1,2 B. Carpenter,3 M. Scuderi,1 R. B. Alley,1 S. Anandakrishnan,1 C. Marone,1and M. Jackson4Received 23 October 2012; revised 12 February 2013; accepted 25 February 2013.[1] New laboratory experiments exploring likely subglacial conditions reveal controlson the transition between stable sliding and stick-slip motion of debris-laden ice overrock, with implications for glacier behavior. Friction between a rock substrate and clastsin ice generates heat, which melts nearby ice to produce lubricating water. An increase insliding speed or an increase in entrained debris raises heat generation and thus meltwaterproduction. Unstable sliding is favored by low initial lubrication followed by rapidmeltwater production in response to a velocity increase. Low initial lubrication can resultfrom cold or drained conditions, whereas rapid increase in meltwater generation resultsfrom strong frictional heating caused by high sliding velocity or high debris loads.Strengthening of the interface (healing) during “stick” intervals between slip eventsoccurs primarily through meltwater refreezing. When healing and unstable sliding aretaken together, the experiments reported here suggest that stick-slip behavior is commonfrom motion of debris-laden glacier ice over bedrock.Citation: Zoet, L. K., B. Carpenter, M. Scuderi, R. B. Alley, S. Anandakrishnan, C. Marone, and M. Jackson (2013), The effectsof entrained debris on the basal sliding stability of a glacier, J. Geophys. Earth Surf., 118, doi:10.1002/jgrf.20052.1. Introductionmaterial ranging from crystalline bedrock to water saturated till [Peters et al., 2006; Smith, 2006; Tulaczyk et al.,2000; Blankenship et al., 1986]. Geological field studies[Fischer and Clarke, 1997; Iverson et al., 2003] and geophysical data provide insights into basal sliding processes[Anandakrishnan and Alley, 1997a, b], and laboratory observations can supplement and extend the sparse field data onglacier-bed seismicity.[3] Field geodetic and direct observations show thatglacier flow can be smooth or stick-slip, with variationsover time [Hubbard, 2002; Winberry et al., 2009a, b; Wienset al., 2008; Zoet et al., 2012] and space at various scales[Wiens et al., 2008; Winberry et al., 2009b; Anandakrishnanand Bentley, 1993; Anandakrishnan and Alley, 1994]. Tides,variable meltwater input, calving, and variations in basaldebris load are among the processes that can affect motionand thus seismicity [Anandakrishnan and Alley, 1997b;Walter et al., 2008; Christianson, 2012; Fischer and Clarke,1997; Nettles et al., 2008; Zoet et al., 2012].[4] The sliding interface at the base of a glacier shareskey characteristics with tectonic fault zones. Unstable sliding in such fault zones results from a dynamic frictionalinstability attendant with local stress drop and seismic radiation [Scholz, 2002]. As stress is recharged at the faultinterface, due to tectonic loading in the case of earthquakefaults, or basal glacial flow, a repetitive stick-slip patternmay emerge. Recent work shows that both fault and subglacial slip exhibit a spectrum of behavior, ranging fromcreep through transient slow slip to dynamic earthquakerupture [Rignot et al., 2011; Winberry et al., 2009a; Zoetet al., 2012]. Data on stable or unstable sliding and subsequent stress recharge are often interpreted within theframework of rate-and-state dependent friction laws (RSF)[2] Glaciers and ice sheets often generate seismic events[West et al., 2010; Anandakrishnan and Alley, 1997a;Weaver and Malone, 1979; Danesi et al., 2007; Zoetet al., 2012], through processes including surface crevassing[Neave and Savage, 1970], calving at the terminus [Ekstromet al., 2006; O’Neel et al., 2010], and outburst flooding[Winberry et al., 2009a]. Many events are caused by suddenslip in the ice-flow direction on planes that are indistinguishable from the glacier bed, within the 10 m depth uncertainty [Smith, 2006; Stuart et al., 2005; Anandakrishnan andAlley, 1997b; Anandakrishnan and Bentley, 1993; Walteret al., 2011; Winberry et al., 2009b]. Current knowledgeof subglacial mechanics suggests that the seismic eventsarise from sliding of debris-rich ice over bedrock [Alley,1993], although ruptures within overriding ice or withinsubglacial material cannot be completely ruled out. Basalice, often up to several meters thick and containing debrisloads up to 40% by volume [Kirkenbride, 2002], slides over1Department of Geoscience, Penn State University, University Park,Pennsylvania, USA.2Now at Department of Geologic and Atmospheric Sciences, IowaState University, Ames, Iowa, USA.3HP-HT Lab, Istituto Nazionale di Geofisica e Vulcanologia, Rome,Italy.4Hydrology Department Norwegian Water Resources and EnergyDirectorate, Oslo, Norway.Corresponding author: L. K. Zoet, Department of Geologic and Atmospheric Sciences, Iowa State University, 362 Science 1, Ames, IA, 50011,USA. (lzoet@iastate.edu) 2013. American Geophysical Union. All Rights Reserved.2169-9003/13/10.1002/jgrf.200521
ZOET ET AL.: DEBRIS EFFECT ON SLIDINGof the friction coefficient with slip following a perturbation[Marone, 1998a]. The parameter a – b represents the changein steady-state friction with slip velocity, with positivevalues indicating so-called velocity-strengthening behaviorand negative values representing velocity-weakening behavior. The stability of frictional slip is determined, to firstorder, by the ratio of b – a over Dc [Rice, 1983]. Velocitystrengthening friction produces only stable frictional sliding,whereas velocity-weakening friction can produce unstablesliding and thus stick-slip behavior.[8] From rate-and-state friction theory, in the velocitystrengthening regime where steady-state friction increaseswith sliding velocity, faster sliding on an interface requiresmore work, which requires higher driving stress and thustends to damp velocity perturbations in response to stressperturbations. This effect combines with atomic-scaled processes to ensure that the friction direct effect a is positive[e.g., Marone, 1998a; Beeler et al., 2008; Li et al., 2011].The friction evolution effect b describes the extent to whichcontact junctions evolve with slip and time following a perturbation from steady state. For extended solid surfaces, theaverage asperity contact lifetime is given by the ratio ofcontact junction dimension divided by slip velocity. Underconditions of velocity weakening friction, where steadystate friction decreases with sliding velocity, contact junction size decreases with increasing slip velocity because atthe higher slip rate, contacts are smaller and/or composedof weaker chemical bonds. Other effects such as increasedlubrication or asperity breakage can further weaken theinterface. Such rate-and-state dependent friction processeslead to velocity-strengthening and velocity-weakening of aninterface, and are represented by a and b, respectively. Thesign of the difference a – b determines whether the interface as a whole is velocity-strengthening and slides stably,or velocity-weakening, which is a necessary condition forstick-slip instabilities.[9] In the context of glacial slip, RSF describes variations in frictional strength of the interface between iceand its underlying rock or sediment layer. The strengthof that interface changes with changes in the populationand lubrication of contacts through breakage, melting orother processes. During the pre-seismic (“stick”) phase ofthe seismic cycle, the strength of the shearing ice/debriszone increases with time at a rate that can be describedby RSF laws. The rate of frictional restrengthening (frictional healing) is governed by time, shear stress, and loadingvelocity [e.g., Karner and Marone, 2001]. Frictional healing results from processes such as creep-induced growthof the contact area [Dieterich and Kilgore, 1994], porosity loss and densification, and strengthening of chemicalbonds within highly-stressed contact junctions [Li et al.,2011]. Many of these processes vary strongly with temperature, and in subglacial environments, freezing of lubricatingmeltwater is likely to play a role in frictional healing ofdebris-laden ice on bedrock [Robin, 1976; Goodman et al.,1979]. Ice also may strengthen through re-entrainment ofdebris melted out during a slip event, through regelation andfreeze-on processes. Such strengthening can contribute tostick-slip behavior [Zoet et al., 2012]. The parameter ˇ isused to quantify the rate at which these frictional healingmechanisms occur, and is estimated through slide-hold-slidetesting (detailed later in this paper).[Dieterich, 1979a; Ruina, 1983; Scholz, 1998]. RSF analysis has been used extensively in earthquake studies [Marone,1998a; Scholz, 1998; Carpenter et al., 2011], but much lesscommonly in cryospheric studies [e.g., Rathbun et al., 2008;Fortt and Schulson, 2009].[5] In this paper, we present results on the frictionalconstitutive properties of debris-bearing and clean glacialice from experiments conducted in a laboratory setting.We focus in particular on the frictional strength, slidingstability, and processes of frictional restrengthening (socalled frictional healing) following stick-slip failure eventsin glaciological settings. We describe a series of laboratory shearing experiments conducted on natural and synthetic glacial ice with a range of debris contents (0–50%by weight) and at a temperature range from –6ı C to thepressure melting point (pmp). Applied stresses and otherexperimental conditions were chosen to be representative ofsubglacial conditions.1.1. Friction Constitutive Laws for Seismic andAseismic Glacial Sliding[6] The rate-state friction laws [Dieterich, 1979a, 1979b;Ruina, 1983; Rice, 1983] describe time- and slip-dependentchanges in frictional strength, and have been used extensively to describe a range of seismic and aseismic slipbehavior on tectonic faults [Marone, 1998a; Scholz, 1998;Rubin, 2011]. In the context of RSF, fault slip events during the seismic cycle, are described by variations in thefrictional strength, , with slip velocity, V, and a statevariable, :ÂV (V, ) 0 a lnV0àb à  ÃV V Pln –DcDcV0 DcÃ(1)(2)where 0 is a reference friction coefficient at a referenceslip velocity, V0 , V is the fault slip velocity, a and b aredimensionless empirical constants, and Dc is the criticalslip distance. Dc is the e-folding slip distance necessary torenew the asperity contacts after a velocity perturbation,and has been related to the size of frictional contact junctions [e.g., Rabinowicz, 1951; Dieterich, 1979a; 1981], orthe porosity, or width of shear localization bands [Maroneand Kilgore, 1993; Marone et al., 2009]. is a state variable that describes the nature of the area of contact at a giventime. Dieterich [1979a] suggested that the state variablecan be interpreted as the average life time of the contacts.Equation (1) describes the relationship between friction,velocity and state. Equation (2) describes the evolution ofstate with slip and time following a slip perturbation. RSFlaws have been generalized to account for changes in normal stress, variable shear localization dimension, and othervariables [Marone, 1998a], but we focus here on the basicform of the laws.[7] The RSF parameter a is a measure of the initialchange in the friction coefficient that results from a suddenchange in sliding velocity, and b represents the evolution2
ZOET ET AL.: DEBRIS EFFECT ON SLIDING[11] Samples of ice were held in a specially designedloading platen and subjected to direct shear against a rocksurface in a modified double-direct shear configuration(Figure 1). Experiments were conducted at constant normalstress, in the range 0.5–1.25 MPa (the main experimentslisted in Table 1 were all run at 1.25 MPa), which waschosen to simulate the weight of the glacier (140 m ofice) on its bed. The drained experimental system preventswater pressure buildup at the interface, and so producesan effective pressure similar to that for a well-drained140 m-thick glacier. Effective pressure is important in controlling subglacial friction [Cuffey and Paterson, 2010].Normal stress was maintained at a constant value using afast-acting hydraulic servo controller with feedback fromcustom made load cell and calibrated to NBS standards.Normal and shear forces are accurate to 5 N and measuredwith precision of 5 N. Shear and normal displacement weremeasured to 0.1 m using direct-current displacementtransducers (DCDT) mounted directly on the loading rams.Samples were sheared by driving the vertical loading ramat constant velocity (Figure 1). A maximum shear displacement of 25 mm is attainable in this configuration. All datawere recorded with a 24 bit digitizer operating at 10 kHz,with data averaged and saved at a rate from 1–1000 Hz,depending on imposed sliding velocity.[12] We constructed ice samples that contained 0–50%debris by weight to represent basal ice with varyingdebris loads. Where access to basal ice has been available[Kirkenbride, 2002], basal debris loads of 0–40% by volume(0–68% by weight) have been observed. The samples wereconstructed after the model of Emerson and Rempel [2007].[13] We used both natural and simulated glacial debris.Debris was chosen with a bimodal (Figure 2) particle-sizedistribution to replicate typical debris entrained in basalice [Kirkenbride, 2002]. The particular debris used consisted primarily of amphibole schist, appropriate for glaciation over a crystalline bedrock. Debris grains larger than1.25 mm ( 101 of the minimum dimension) were removed,following the standard convention in soil testing.[14] Preparation of the samples consisted of crushingdebris-free ice until no grains larger than 2 mm in diameterremained. Ice grains were then placed in a container with aknown debris mass, and the debris-ice mixture was agitateduntil all
L. K. Zoet,1,2 B. Carpenter,3 M. Scuderi,1 R. B. Alley,1 S. Anandakrishnan,1 C. Marone,1 and M. Jackson4 Received 23 October 2012; revised 12 February 2013; accepted 25 February 2013. [1] New laboratory experiments exploring likely subglacial conditions reveal controls on the transition between stable sliding and stick-slip motion of debris-laden ice over rock, with implications for glacier .