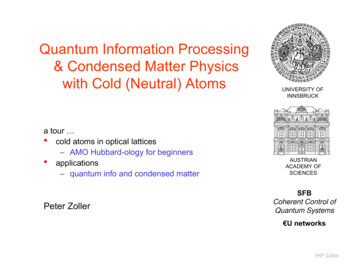
Transcription
Quantum Information Processing& Condensed Matter Physicswith Cold (Neutral) Atomsa tour cold atoms in optical lattices– AMO Hubbard-ology for beginners applications– quantum info and condensed matterPeter ZollerUNIVERSITY OFINNSBRUCKAUSTRIANACADEMY OFSCIENCESSFBCoherent Control ofQuantum Systems U networksIHP Zoller
a sneak preview:cold atoms in optical latticesIHP Zoller
Cold atoms in optical latticeslaser Loading cold bosonic or fermionicatoms into an optical lattice Atomic Hubbard models withcontrollable parameters– bose / fermi atoms– spin models optical lattice as a regulararray of microtraps for atomsOptical latticelasernonresonantlaserBloch bandAC Stark shifttunnelingoptical lattice as array of microtrapsIHP Zoller
Cold atoms in optical latticeslaser Loading cold bosonic or fermionicatoms into an optical lattice Atomic Hubbard models withcontrollable parameters– bose / fermi atoms– spin modelsoptical lattice as a regulararray of microtraps for atoms Example: Bose Hubbard modelH XJαβ b†α bβα6 β1 X † † Ubα bα bα bα2αkinetic energy:hopping b , b † interaction:onsite repulsionbosonsIHP Zoller
Cold atoms in optical latticeslaser Loading cold bosonic or fermionicatoms into an optical lattice Atomic Hubbard models withcontrollable parameters– bose / fermi atoms– spin models atomic physics: features– microscopic understanding ofHamiltonian– controlling & "engineering"interactions / Hamiltonian– "engineering Hubbard Hamiltonians"optical lattice as a regulararray of microtraps for atoms Example: Bose Hubbard modelH XJαβ b†α bβα6 β1 X † † Ubα bα bα bα2αkinetic energy:hopping b , b † interaction:onsite repulsionbosonsIHP Zoller
Cold atoms in optical latticeslaser Loading cold bosonic or fermionicatoms into an optical lattice Atomic Hubbard models withcontrollable parameters– bose / fermi atoms– spin models atomic physics: features– microscopic understanding ofHamiltonian– controlling & "engineering"interactions / Hamiltonian– adding internal degrees offreedom"engineering Hubbard Hamiltonians"optical lattice as a regulararray of microtraps for atoms filling the lattice with "qubits"IHP Zoller
Cold atoms in optical latticeslaser Loading cold bosonic or fermionicatoms into an optical lattice Atomic Hubbard models withcontrollable parameters– bose / fermi atoms– spin modelsoptical lattice as a regulararray of microtraps for atoms additional aspects / features– controlled lattice loading, e.g. unitfilling– measurement– – adding phonons – adding (controlled) dissipation IHP Zoller
Cold atoms in optical latticeslaser Loading cold bosonic or fermionicatoms into an optical lattice Atomic Hubbard models withcontrollable parameters– bose / fermi atoms– spin models atomic physics: features– microscopic understanding ofHamiltonian– controlling & "engineering"interactions / Hamiltonian– adding internal degrees offreedomoptical lattice as a regulararray of microtraps for atoms additional aspects / features– controlled lattice loading, e.g. unitfilling– measurement– – adding phonons – adding (controlled) dissipation "Atomic Hubbard toolbox"IHP Zoller
Why? condensed matter physics & quantum information condensed matter physics– strongly correlated systems: high Tc etc.– time dependent, e.g. quantum phasetransitions– – exotic quantum phases (?) quantum information processing– new quantum computingscenarios, e.g. "one way quantumcomputer""quantum simulator„ qubits on a lattice-analogue simulators- digital simulators entangling qubits via "Ising"(cluster state)experiments [Bloch et al. 2001, T. Esslinger et al., J. Denschlag et al.]– Superfluid-Mott Insulator Quantum Phase Transition– spin dependent lattice & entanglement– molecules IHP Zoller
1. “Analogue simulation” of cond mat Hubbard models We can build Hubbard models directly to simulate condensed mattermodels with controllable parametersExample:Thigh T c superconductivityH t (ci σ c jσ c jσ ciσ ) U ni ni i , j ,σspinliquidsiAFM SCdopingSolving open problems where theory does not give an answer:– ground state; strange quantum phase & properties– exotic excitationsIHP Zoller
2. and Lattice Spin Models: topological quantum computing exotic spin models Examples:KitaevDoucot, Feigelman, Ioffe et al.xxzzZZXX YYℓ 1ℓ 1z zx xH I spin i 1 j 1 J i,j i,j 1 cos i,j i 1,j protected quantum memory:degenerate ground states as qubitsH II spin J xj xk J x links Jz yj yky links zj zkz linksIHP Zoller
3. Digital Quantum Simulator – an example Idea: build a Hamiltonian as a time-averaged effective Hamiltonianfrom one and two qubit gateswe will show that we cando this “easily” with atomsExample: given Ising:H J2 b 〈a,b a z z we want to simulate the Heisenberg model:H J2 b a b a b 〈a,b a x x y y z zIsing HIsingle qubitrotations U(t)IHP Zoller
Raussendorf & Briegel, PRL 20014. Measurement based quantum computing Cluster state as a quantum resource: exploit quantum correlationsInformation processing via measurement of single qubits on a lattice2D cluster stateIHP Zoller
How to create a cluster state? prepare quantum memory in product state s x a C awith each qubit in state 1 0 z 1 z 2 switch on Ising interactionH int g t a,b 14 a z b z for a given time t 0 g t dt 2D cluster state c exp i t 10 4 b g t dt a,b a z z dt a C a This can be implemented efficiently with a spin-dependent optical lattice.IHP Zoller
Measurement based quantum computing Cluster state as a quantum resource: exploit quantum correlationsInformation processing via measurement of single qubits on a latticeIHP Zoller
Measurement based quantum computing Cluster state as a quantum resource: exploit quantum correlationsInformation processing via measurement of single qubits on a latticeflow of informationquantum gateIHP Zoller
Quantum Gates a a b a b Controlled-NOTtruth table CNOT:flow of information 0 0 0 0 0 1 0 1 of 1 1 Û1 1 0 rotationa single qubit 1 1 1 0 U1IHP Zoller
AMO Hubbard "toolbox" Optical lattices– basic ideas, properties & special topics Hubbard models– naive derivation & microscopic picture, spin models, validity Lattice loading & Measurements Time-dependent aspectsIHP Zoller
1. Optical lattices: basics AC Stark shift e nonresonantlaserAC Stark shiftΔE I eg g red detunedblue detuned I E 2 decoherence: spontaneous emissionΔE g Δ2good e laser1 24 Δ 1 iΓ spontaneousemissionΓ g bad E g g E g i 12 g Δ Γ 1-typical off-resonant lattice : γ sec 1In a blue detuned lattice this can bestrongly suppressedIHP Zoller
standing wave laser configurationlaser E E 0sin kze i t c. c.laseroptical potentialBloch bandtunnelingoptical lattice as array of microtrapsV x V 0 sin 2 kx k 2 Schrödinger equation for center of mass motion of atom i t x, t 2m 2 V x x, t 2IHP Zoller
1D, 2D and 3D lattice configurationslasersquare latticeRemarks:optical potentials generated by latticebeams with different frequencies addup incoherentlyinterferometric stability (!?)lasertriangular latticeIHP Zoller
harmonic background potential (e.g. laser focus, magnetic trap)undo by inverse harmonicpotential e.g. magnetic field superlattice random potentials– add more lasers from random direction or speckle pattern Single atom coherent dynamcis studied – Wannier-Bloch– quantum chaos: kicked systemsIHP Zoller
Jaksch et al 19982. Bose Hubbard in optical lattice: naïve derivation dilute bose gasH Zµ¶2h̄ψ† ( x) 2 VT ( x) ψ( x) d3 x2mZ1 g ψ† ( x)ψ† ( x)ψ( x)ψ( x) d3 x2collisions validity: dilute gas, a s a 0 /2 optical lattice4 a s 2mg ψ( x) Xαscattering lengthw( x xα ) bαWannierfunctionsIHP Zoller
Hubbard modelH J i,j b †i b j kinetic engery:hopping12U i b †i b †i b ib i i i b †i b iinteraction:onsite repulsionU g Z w( x) 4 d3 xfeature: (time dep) tunability from weakly to strongly interacting gasvalidity IHP Zoller
parameters approx. SF-Mott transition:recoil energy E R 2 k 2 /2m, V 0 9E RNa: E R 25 KHz, J 1 KHz, U 10 KHz,Rb: E R 3. 8 KHz . validity:a s a 0 /2, U Bloch and T 0 :kT J, U Bloch,density n 10 14 10 15 cm 3 (three particle loss)IHP Zoller
Hubbard model: microscopic picture HubbardUJ h̄ωtrapsolve in n 1,2,3, particle sectorconnect by tunneling (e.g in a tightbinding approx)n 2 atoms on one lattice site: moleculemolecular problem with added opticalpotential n 3 atoms on one lattice site: e.g. Efimov-type problem[n n {max} 3 killed by three body etc. loss]IHP Zoller
Julienne et al.two atoms on one site 2 2 2m 2R 2 2r 2U1212 2m 2 R 2 cm R E cm cm R 2 r 2 V r r E r h̄ωtrap12 2 r 2v 2 3vv 1v 0harmonicapproximation Born Oppenheimerpotentials including trapC6r6(schematicnot too scale)De 10 12 Hz 5 nmscattering length as a0trap size a0IHP Zoller
two atoms on one siteUh̄ωtrap12 2 r 2v 2 3vv 1v 0"2 atoms":onsite interactionU "shift"IHP Zoller
two atoms on one sitephotoassociationresonanceh̄ωtrapUΩ12 2 r 2v 2 3vv 1v 0"2 atoms":AC Starkshift opticalFeshbach resonanceUeff U bg Δ"molecule"1 24 ΔΔH U bg 1 24 Δ b †2 b 2IHP Zoller
Hubbard model including molecules HamiltonianH J b b †i b j 1 U b b †i b †i b ib i2 i,j i J m m†i mj 1 U m m†i m†i m imi Δm †i mi2 i,j atoms moleculeii 1 m †i b i b i h.c.2iRemarks:we have derived this only for sector:2 atoms or 1 moleculeinelastic collisions / loss for 2atoms and 1 molecules (?)IHP Zoller
Remark: quantum phases of "composite objects" molecular BEC via a quantum phase transitionatomic BECswitch onoptical latticemolecular BECquantummeltquantumfreezeMott insulator:pattern loadingphotoassociationconverts atoms100% to molecules molecular Mott insulatoroptical lattice:array of microtrapsIHP Zoller
Remarks:Straightforward generalization of these Hubbard derivations to bosons and / or fermions two-component mixtures of bosons / fermions dipolar gases / Hubbard models via heteronuclear molecules (longrange dipolar forces)Complaints: the time scales for tunneling are pretty long decoherence: spontaneous emission, laser / magnetic fieldfluctuations, Other ideas for interactions optical dipole-dipole interactions however loss Rydberg-Rydberg interactions in a static electric field (huge)μ1μ2V dip r IHP Zoller
e.g. Duan et al.Spin models optical lattice bosons in a Mott phase H J i,j , b †i b j 〈n i 〈n i 112U i, n i n i 1 U i n i n i H 〈i,j z zi zj xi xj yi yj J2Upretty small XXZ-model zi n i n i xi b † i b i b †i b i i i b † i b i b †i b i yideas for higher order H σ σ σ interactions IHP Zoller
Preparation of the lattice for quantum computing:one atom per lattice site superfluid – Mott insulator quantum phase transition in optical latticesIHP Zoller
Laser control: kinetic vs. potential energy shallow lattice : weak laserU small J largeweakly interacting system:J U(kinetic energy interactions)laser parameters(time dependent)deep lattice: intense laserstrongly interacting system:J U(kinetic energy interactions)U largeJ smallIHP Zoller
Quantum phase transition Hamiltonianexample: gasno commoneigenvectorskineticenergyinteractionenergyg 0: dilute gasg : strongly correlated systemIHP Zoller
Quantum phase transition Hamiltonian crossing the critical point (temperature T 0)no commoneigenvectorsEnergy eigenstate of H1 eigenstate of H0critical point why interesting?– fundamental interest– engineer interesting states . e.g entangled statesIHP Zoller
BH: Fischer et al. 1989optical lattice: Jaksch et al. 1998Superfluid – Mott insulator quantum phase transition N atoms in M lattice sites: (temperature T 0)α superfluid J U competing interactions Blochband2Jdelocalized atoms: BEC³ N³ N†††bk 0 vaci b1 . . . bM vacikIHP Zoller
Mott phase: J Ucommensurate filling N Mexcitations: gapped UUrobust!b†1 b†2 . . . b†M vaciincommensurate filling N / Mexcitations: J superfluidMott coreIHP Zoller
1D “exact” calculationsU/J 1Superfluid and Mottin 2D trapWe achieve loading with exactly1,2, atoms!n 2n 1C. KollathJ. von DelftU. SchollwöckU/J 10density:“wedding cake”U/J 17yJaksch et al. 1998xIHP Zoller
Signatures of the Superfluid – Mott insulator transition spatial correlation functionαsuperfluidMottββααoff-diagonal long range order:interferenceno interferenceexp signatureIHP Zoller
freezesuperfluidMottmeltsuperfluidspatial correlation functions (theory):βoff-diagonallongrange orderinterferenceαNO interferenceinterferenceI. Bloch, T. Hänsch et al., Nature Jan 3 2002IHP Zoller
3. Optical Lattices continued multiple ground states & spin-dependent lattices2P3/22P1/2σ σ F 22S1/2F 1shiftshift 2P2P1/23/2 laser frequency2P2P1/23/2IHP Zoller
multiple ground states & spin-dependent lattices2P3/22P1/2σ σ2S1/2F 1 /2 /2F 2 /2Gk /2 /2 e ikz i t /2 e ikz i tE cos kz /2 sin kz /2 IHP Zoller
multiple ground states & spin-dependent lattices2P3/22P1/2σ2S1/2σ F 2F 1displace via polarization angleIHP Zoller
Two component Hubbard models hopping via Raman transitions nearest neighbor interactionlaser inducedRaman hoppingoverlappingwavefunctions laser inducedRaman hoppinginternal statestwo lattices can bemoved timedepIHP Zoller
Adding “magnetic fields” effective magnetic field viarotation effective magnetic field vialattice designΩieJαβ Jαβ eRβα lA·dsee: fractional quantumHall effectieeH lA·d eiφ/φ0 eiαπaccumulate phase whenwalking around a plaquetteIHP Zoller
Jaksch & PZ 2003 effective magnetic fields: configurationyΩ1Ω*2xyspatially varying phasesof the Raman lasersequivalent to a homogeneousmagnetic fieldIHP Zoller
HP Büchler et al., PRL2005 two particle hopping Uring exchangeenergyconservationIHP Zoller
4. Coherent manipulations & entangling atomsIHP Zoller
Spin-dependent lattices trapping potential depends on the internal stateinternal states we can move one potential relative to the other, and thus transport thecomponent in one internal stateAlkali atomfinestructuremove via laserparametersIHP Zoller
interactions by moving the lattice colliding the atoms “by hand”atom 1atom 2internal stateseiφ eiφ eiφ eiφ10 1010 10 1move0collision “by hand“ Ising type interaction (as the building block of the UQS)H J2 b 〈a,b a z znearest neighbor, next to nearest neighbor .IHP Zoller
Universal Quantum Simulator(specialized quantum computing) Feynman: simulating a quantum system with a classical computer is hardExample: condensed matter– spin models– Hubbard models2N complex coefficients Feynman’s Universal Quantum Simulator (UQS):Feynman, Lloyd, .UQS controlled quantum device which efficientlyreproduces the dynamics of any othermany-particle quantum system (with shortrange interactions)IHP Zoller
UQS: a simple examplerealized by movable opticallattice given Ising: simulate Heisenberg model:H J2H J2 b 〈a,b a zz b a b a b 〈a,b a x x y y z zIsing HI 13p3 V1 1 Np2 V3 p1 V2 1312U(t)1312 1 i y N 1 i x Nadding: magnetic fields, random potentials etc.IHP Zoller
A. MicheliA. DaleyD. JakschPZPRL Oct 20045. Atomic Quantum Switch / Amplifier / Read Out1D quantum gasqubit as a switchIHP Zoller
Quantum OpticsMesoscopic AMO "single atom quantum" mirrorsfor 1D quantum gasinteractingCavities and CQEDa, a †Mott / BECbosonic atoms single atom as a cavity mode: bosonicatom: spin-1/2mirrorqubit spin-1/2 Questions – how?– (time dependent) dynamics?– quantum gate / switch– qubit read out Jaynes-Cummings model / quantumstate engineering dissipative / open quantum system /measurement: e.g.QND experimental realization: microwave (Haroche, ) optical (Kimble, Rempe, Feld, )IHP Zoller
Mesoscopic AMO "single atom quantum" mirrorsfor 1D quantum gas qubit “0” BLOCK:Mottqubit “1” OPEN:Quantum Computing quantum gate / switch / quantumnondemolitionqubit atom x BEC atoms y BECQND x x y x c x x y x c x x x y Mott BEC "amplify" spinentangled quantum phasesqubit read outIHP Zoller
Mesoscopic CMPMesoscopic AMO atomic qubit spin transport through quantum dot /Coulomb blockadeorspin up:reflectionspin down:transmission"coherent spintronics""single atom transistor""single electron transistor"(e.g. read out of a charge qubit)IHP Zoller
Mesoscopic AMO "single atom quantum" mirrorsfor 1D quantum gasPhysical Realization realization: 1D optical latticeBEC JJIHP Zoller
How? collisional interactions compare: Cavity QED e site "0"atom g a, a †cavity r density-densityH Uab a †0 a 0 b †0 b 0 spinH a †0 a 0 ̂ z H a † a g 〈g a † a ̂ zAC-Starkshift a † a, H 0QND photon numbergoal: large interactionsanswer: Feshbach or photoassociation resonances- large scattering length- better: infinite scattering lengthnew physics: EIT-type quantuminterference to kill transportIHP Zoller
Micheli et al.Atomic Switch by Quantum Interference tunneling through the quantum dot?J efftunneling ? process in an optical latticebeforeduringafterRaman laser 2 atoms molecule exactly on resonance:AC Stark splitting optical trap 2 atoms molecule 2 atoms molecule internuclear separationIHP Zoller
blocking by quantum interferencebeforeduringEnergyafter 2 atoms molecule 0 atom atom atom atom 2 atoms molecule J eff J1E 1 2J J1E 1 !J 0 (for E 0)2we kill the transport by quantum interference:“infinite repulsion” (EIT)IHP Zoller
Time dependent many bodydynamics [Exact] Solution of time dependent many body Schrödinger equationfor up to 100 atoms– numerical: time dep DMRG-type method G. Vidal, PRL 2003– semianalytical: hard core bose gas numerical results for N 30 atoms on 61 lattice sitesswitch openxtimeatomic densityswitch closed timexxxcurrent-voltage characteristics for transport IHP Zoller
Exact numerical time dependent many body in 1D method: G. Vidal, PRL 91, 147902 (2003)efficient classical simulation of slightly entangled quantumcomputationsmeasure of entanglement: e.g. spin systemSchmidt decompositionBA B A 1 A Schmidt coefficients H nd A A is eigenvector of reduced density matrix rank A of A is a natural measure of entanglement: maxpossible AE log2 A partitions Aproperty: 0 E 12n log2 d and E 0 for product stateIHP Zoller
computational basis: c i ,i , ,i i 1 i 2 i n 1 2i1ninparticular decomposition of coefficients: i nc i1 ,i2 , ,in 1 n Γ 11 i1 1 Γ 21 i22 2 Γ 32 i33 Γ nn 1n tensors Γ 1 , ,Γ n n 1 vectors i 0, 1, , d 1 1, , 2 n coefficients c 2 2 ngeneric state if E n, then decomposition uninteresting (O ne n ) if E logn, then poly n parameterspropagation of the time dependent Schrödinger equation: propagate Γand λIn our case of the Hubbard model: χ 8we can integrate the time dependent Schrödinger equation exactly forup to a few hundred (!?) particles (D. Jaksch & A. Daley) IHP Zoller
6. Dissipative Dynamics in Lattices: Cooling etc.IHP Zoller
Rabl et al. PRL 2003Healing defects & Pattern loading Getting rid of the last defects ?– cooling– filteringfilling thehole? loading spatial patterns fidelity of loading 1:104 or 105getting rid of theextra atom?IHP Zoller
Cooling via Superfluid Immersion We immerse the lattice system in an external BECΓa bBEC asreservoirBose condensate as a reservoiratoms “a” see the lattice, bose reservoir atoms “b” see no latticeCooling via (laser assisted) collisional interactionsRemarks: We will be able to cool to temperatures lower than the reservoir Analogy with laser cooling: photon - phononIHP Zoller
Cooling via Superfluid Immersion ModelBEC BA-B collisionsBEC as T 0 reservoir to cool qubit motionRemarks: We will be able to cool to temperatures lower than the reservoir Cooling within a Bloch band Analogy with laser cooling: photon - phononA. J. Daley, P. O. Fedichev, and P. Zoller, Phys. Rev. A 69, 022306 (2004)IHP Zoller
Cooling model Atom in a Harmonic trap interacting with a superfluid reservoirDensity-Density interaction: Energy dissipated as Bogoliubov excitations: Master Equation ! occupation probabilities pm:IHP Zoller
cooling process: excitation of phonons in the superfluidṗ n master equation occupation:SF as a reservoirBEC supersonic regime: sound velocity u velocity of trapped atom 1 02 1. 2 10 2 a ab1 0 a 3ab l 0cooling time 10 oscillator cyclesRubidium BEC: 0 10 14 cm 3 a bb 100a 0m b u 2 / 2 2 3. 7 10 2 s 1 2 10 5 s 1 ̇ n g 2ab 0 m 3/2 42 n 3/2energy dissipationIHP Zoller
BEC as T 0phonon reservoir Radiation field:photon reservoirPhonon reservoir Radiation field / vacuum modes Spontaneous emissionBEC (T 0)phonons bosonic excitations qparticlesphonons qSympathetic cooling: collision e ΓBEC g IHP Zoller
A reminder optical pumping ri g 1 systemmixed statesystempure state g 2 laser coolingRemark 2:phonon(T finite)ionlaser sys env sys〈 ′envspontaneousemission(T 0)laserRemark 1:trapspontaneousemissionlaser e Γ spontaneous g emission(T 0)"low frequency / high-temperature" systemdissipative "high-frequency" / T 0 systemPhoton dissipation / spontaneous emission not useful for lattice loading. We need adifferent reservoir.IHP Zoller
Cooling within the Bloch Band Ideas taken from laser cooling below therecoil limit (Chu & Kasevich; Aspect,Cohen-Tannoudji)Question: Can we cool dilute/ weakly interacting atoms in the latticewithin a Bloch band?Idea: Combine Raman excitation to a higher band with cooling via anexternal reservoir gas.ωω 4J14J00 π0πqaA. Griessner, A. J Daley, D. Jaksch, and P. Zoller, in preparationIHP Zoller
Bloch Band Raman Cooling: Pulses We can use a series of time dependent pulses to engineer theexcitation profile, via Raman detuning, Δ, wavenumber difference δq,and pulse shape [Ω(t)].A sequence of pulses can then excite all atoms but a few near q 0.Ω(t)ΔP(q)ωω 4J1δq4J00 π0πqa π0πIHP Zoller
Bloch Band Raman Cooling: Discussion Features:– the atoms in the lowest Bloch band move frictionless in the BEC (subsonic superfluidity), i.e. a BEC temperature larger than the atom temperaturewill not heat– by laser assisted transfer to the higher Bloch band the emitted “phonon“ isin the particle excitation branch, i.e. supersonic, and cooling is possible– Thus we are able to cool to temperatures lower than the temperature of thereservoir BEC.Typical Values: Tf 10-2J in time Θ 30ms for 87Rb!!ωω 4J14J00 π0πqaIHP Zoller
& Condensed Matter Physics with Cold (Neutral) Atoms a tour cold atoms in optical lattices - AMO Hubbard-ology for beginners applications - quantum info and condensed matter SFB Coherent Control of Quantum Systems U networks Peter Zoller. IHP Zoller a sneak preview: cold atoms in optical lattices.