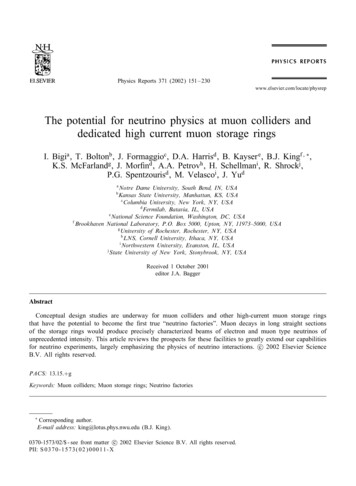
Transcription
Physics Reports 371 (2002) 151 – 230www.elsevier.com/locate/physrepThe potential for neutrino physics at muon colliders anddedicated high current muon storage ringsI. Bigia , T. Boltonb , J. Formaggioc , D.A. Harrisd , B. Kayser e , B.J. Kingf ; ,K.S. McFarlandg , J. Mor/nd , A.A. Petrovh , H. Schellmani , R. Shrockj ,P.G. Spentzourisd , M. Velascoi , J. YudaNotre Dame University, South Bend, IN, USAKansas State University, Manhattan, KS, USAcColumbia University, New York, NY, USAdFermilab, Batavia, IL, USAeNational Science Foundation, Washington, DC, USAfBrookhaven National Laboratory, P.O. Box 5000, Upton, NY, 11973–5000, USAgUniversity of Rochester, Rochester, NY, USAhLNS, Cornell University, Ithaca, NY, USAiNorthwestern University, Evanston, IL, USAjState University of New York, Stonybrook, NY, USAbReceived 1 October 2001editor J.A. BaggerAbstractConceptual design studies are underway for muon colliders and other high-current muon storage ringsthat have the potential to become the /rst true “neutrino factories”. Muon decays in long straight sectionsof the storage rings would produce precisely characterized beams of electron and muon type neutrinos ofunprecedented intensity. This article reviews the prospects for these facilities to greatly extend our capabilitiesc 2002 Elsevier Sciencefor neutrino experiments, largely emphasizing the physics of neutrino interactions. B.V. All rights reserved.PACS: 13.15. gKeywords: Muon colliders; Muon storage rings; Neutrino factories Corresponding author.E-mail address: king@lotus.phys.nwu.edu (B.J. King).c 2002 Elsevier Science B.V. All rights reserved.0370-1573/02/ - see front matter PII: S 0 3 7 0 - 1 5 7 3 ( 0 2 ) 0 0 0 1 1 - X
152I. Bigi et al. / Physics Reports 371 (2002) 151 – 230Contents1. Overview . . . . . . . . . . . . . . . . . . . . . . . . . . . . . . . . . . . . . . . . . . . . . . . . . . . . . . . . . . . . . . . . . . . . . . . . . . . . . . . . . . . . . . . . . .1.1. Introduction . . . . . . . . . . . . . . . . . . . . . . . . . . . . . . . . . . . . . . . . . . . . . . . . . . . . . . . . . . . . . . . . . . . . . . . . . . . . . . . . . . . .1.2. Experimental overview . . . . . . . . . . . . . . . . . . . . . . . . . . . . . . . . . . . . . . . . . . . . . . . . . . . . . . . . . . . . . . . . . . . . . . . . . .1.2.1. High-current muon storage rings . . . . . . . . . . . . . . . . . . . . . . . . . . . . . . . . . . . . . . . . . . . . . . . . . . . . . . . . . . .1.2.2. Event rates . . . . . . . . . . . . . . . . . . . . . . . . . . . . . . . . . . . . . . . . . . . . . . . . . . . . . . . . . . . . . . . . . . . . . . . . . . . . . .1.2.3. Neutrino production spectra and event rates in detectors . . . . . . . . . . . . . . . . . . . . . . . . . . . . . . . . . . . . . .1.2.4. Detector design considerations for MCs . . . . . . . . . . . . . . . . . . . . . . . . . . . . . . . . . . . . . . . . . . . . . . . . . . . .1.3. Physics overview . . . . . . . . . . . . . . . . . . . . . . . . . . . . . . . . . . . . . . . . . . . . . . . . . . . . . . . . . . . . . . . . . . . . . . . . . . . . . . .1.3.1. QCD and deep inelastic scattering . . . . . . . . . . . . . . . . . . . . . . . . . . . . . . . . . . . . . . . . . . . . . . . . . . . . . . . . .1.3.2. The CKM quark mixing matrix . . . . . . . . . . . . . . . . . . . . . . . . . . . . . . . . . . . . . . . . . . . . . . . . . . . . . . . . . . . .1.3.3. Precision electroweak physics . . . . . . . . . . . . . . . . . . . . . . . . . . . . . . . . . . . . . . . . . . . . . . . . . . . . . . . . . . . . .1.3.4. Rare and exotic processes . . . . . . . . . . . . . . . . . . . . . . . . . . . . . . . . . . . . . . . . . . . . . . . . . . . . . . . . . . . . . . . . .1.3.5. Charm decays . . . . . . . . . . . . . . . . . . . . . . . . . . . . . . . . . . . . . . . . . . . . . . . . . . . . . . . . . . . . . . . . . . . . . . . . . . .1.3.6. Neutrino oscillations . . . . . . . . . . . . . . . . . . . . . . . . . . . . . . . . . . . . . . . . . . . . . . . . . . . . . . . . . . . . . . . . . . . . .2. Deep inelastic scattering and QCD studies . . . . . . . . . . . . . . . . . . . . . . . . . . . . . . . . . . . . . . . . . . . . . . . . . . . . . . . . . . . . . .2.1. Background on measuring parton distribution functions and QCD with non-polarized targets . . . . . . . . . . . . .2.2. Measurement of quark parton distribution functions . . . . . . . . . . . . . . . . . . . . . . . . . . . . . . . . . . . . . . . . . . . . . . . . .2.3. Tests of perturbative QCD . . . . . . . . . . . . . . . . . . . . . . . . . . . . . . . . . . . . . . . . . . . . . . . . . . . . . . . . . . . . . . . . . . . . . . .2.4. Heavy quark production . . . . . . . . . . . . . . . . . . . . . . . . . . . . . . . . . . . . . . . . . . . . . . . . . . . . . . . . . . . . . . . . . . . . . . . . .2.5. Parton distribution functions at large Bjorken x . . . . . . . . . . . . . . . . . . . . . . . . . . . . . . . . . . . . . . . . . . . . . . . . . . . . .2.6. Examining the spin structure of the nucleon . . . . . . . . . . . . . . . . . . . . . . . . . . . . . . . . . . . . . . . . . . . . . . . . . . . . . . .2.7. Experimental setup and measurement technique . . . . . . . . . . . . . . . . . . . . . . . . . . . . . . . . . . . . . . . . . . . . . . . . . . . . .2.8. Applications of polarized parton distribution data from MCs . . . . . . . . . . . . . . . . . . . . . . . . . . . . . . . . . . . . . . . .2.9. Studying nuclear eJects with neutrinos . . . . . . . . . . . . . . . . . . . . . . . . . . . . . . . . . . . . . . . . . . . . . . . . . . . . . . . . . . . .2.9.1. Low x: PCAC and nuclear shadowing . . . . . . . . . . . . . . . . . . . . . . . . . . . . . . . . . . . . . . . . . . . . . . . . . . . . . .2.9.2. Mid-x: anti-shadowing and the EMC eJect . . . . . . . . . . . . . . . . . . . . . . . . . . . . . . . . . . . . . . . . . . . . . . . . . .2.9.3. High x: multi-quark cluster eJects . . . . . . . . . . . . . . . . . . . . . . . . . . . . . . . . . . . . . . . . . . . . . . . . . . . . . . . . .3. Studies of the CKM quark mixing matrix . . . . . . . . . . . . . . . . . . . . . . . . . . . . . . . . . . . . . . . . . . . . . . . . . . . . . . . . . . . . . .3.1. Introduction . . . . . . . . . . . . . . . . . . . . . . . . . . . . . . . . . . . . . . . . . . . . . . . . . . . . . . . . . . . . . . . . . . . . . . . . . . . . . . . . . . . .3.1.1. Current experimental knowledge of the relevant CKM matrix elements, and future expectations . . . .3.1.2. Extracting CKM matrix elements in MCs: an overview . . . . . . . . . . . . . . . . . . . . . . . . . . . . . . . . . . . . . .3.2. Analyses involving charm production: the extraction of Vcd and Vcs . . . . . . . . . . . . . . . . . . . . . . . . . . . . . . . . . . .3.3. Analyses involving bottom production: the extraction of Vub and Vcb . . . . . . . . . . . . . . . . . . . . . . . . . . . . . . . . . .3.4. Vcd Vcs via diJractive charmed vector meson production . . . . . . . . . . . . . . . . . . . . . . . . . . . . . . . . . . . . . . . . . . . . .3.5. Improved knowledge of the CKM matrix from MCs . . . . . . . . . . . . . . . . . . . . . . . . . . . . . . . . . . . . . . . . . . . . . . .4. Precision electroweak studies . . . . . . . . . . . . . . . . . . . . . . . . . . . . . . . . . . . . . . . . . . . . . . . . . . . . . . . . . . . . . . . . . . . . . . . . .4.1. Introduction . . . . . . . . . . . . . . . . . . . . . . . . . . . . . . . . . . . . . . . . . . . . . . . . . . . . . . . . . . . . . . . . . . . . . . . . . . . . . . . . . . . .4.1.1. Knowledge at the time of MCs . . . . . . . . . . . . . . . . . . . . . . . . . . . . . . . . . . . . . . . . . . . . . . . . . . . . . . . . . . .4.1.2. Goals of /xed-target electroweak physics programs . . . . . . . . . . . . . . . . . . . . . . . . . . . . . . . . . . . . . . . . . . .4.1.3. Electroweak processes with neutrinos . . . . . . . . . . . . . . . . . . . . . . . . . . . . . . . . . . . . . . . . . . . . . . . . . . . . . . .4.2. Elastic and quasi-elastic neutrino–electron scattering . . . . . . . . . . . . . . . . . . . . . . . . . . . . . . . . . . . . . . . . . . . . . . . .4.2.1. Survey of neutrino–electron scattering processes . . . . . . . . . . . . . . . . . . . . . . . . . . . . . . . . . . . . . . . . . . . . .4.2.2. Current measurements of sin2 W from neutrino–electron scattering . . . . . . . . . . . . . . . . . . . . . . . . . . . . .4.2.3. Overview of the measurement technique at a MC . . . . . . . . . . . . . . . . . . . . . . . . . . . . . . . . . . . . . . . . . . .4.2.4. Statistical sensitivity . . . . . . . . . . . . . . . . . . . . . . . . . . . . . . . . . . . . . . . . . . . . . . . . . . . . . . . . . . . . . . . . . . . . . .4.2.5. Detector design and background rejection . . . . . . . . . . . . . . . . . . . . . . . . . . . . . . . . . . . . . . . . . . . . . . . . . . .4.2.6. Flux normalization for neutrino–electron elastic scattering . . . . . . . . . . . . . . . . . . . . . . . . . . . . . . . . . . . . .4.2.7. Sensitivity to new physics processes . . . . . . . . . . . . . . . . . . . . . . . . . . . . . . . . . . . . . . . . . . . . . . . . . . . . . . . .4.3. sin2 W from deep inelastic scattering . . . . . . . . . . . . . . . . . . . . . . . . . . . . . . . . . . . . . . . . . . . . . . . . . . . . . . . . . . . . . 186186187187187188188188189190191191193194195195
I. Bigi et al. / Physics Reports 371 (2002) 151 – 2304.3.1. Previous measurements . . . . . . . . . . . . . . . . . . . . . . . . . . . . . . . . . . . . . . . . . . . . . . . . . . . . . . . . . . . . . . . . . . .4.3.2. The experimental extraction of sin2 W . . . . . . . . . . . . . . . . . . . . . . . . . . . . . . . . . . . . . . . . . . . . . . . . . . . . . .4.3.3. Detector requirements . . . . . . . . . . . . . . . . . . . . . . . . . . . . . . . . . . . . . . . . . . . . . . . . . . . . . . . . . . . . . . . . . . . .4.3.4. Estimated uncertainties . . . . . . . . . . . . . . . . . . . . . . . . . . . . . . . . . . . . . . . . . . . . . . . . . . . . . . . . . . . . . . . . . . .4.3.5. Comparisons of expected precisions from sin2 W measurements in diJerent experimental processes .4.4. Summary on sin2 W measurements at MCs . . . . . . . . . . . . . . . . . . . . . . . . . . . . . . . . . . . . . . . . . . . . . . . . . . . . . . .5. Rare and exotic processes . . . . . . . . . . . . . . . . . . . . . . . . . . . . . . . . . . . . . . . . . . . . . . . . . . . . . . . . . . . . . . . . . . . . . . . . . . . .5.1. New physics sensitivity . . . . . . . . . . . . . . . . . . . . . . . . . . . . . . . . . . . . . . . . . . . . . . . . . . . . . . . . . . . . . . . . . . . . . . . . .5.1.1. Flavor changing neutral currents . . . . . . . . . . . . . . . . . . . . . . . . . . . . . . . . . . . . . . . . . . . . . . . . . . . . . . . . . . .5.1.2. Generic four-fermion operators . . . . . . . . . . . . . . . . . . . . . . . . . . . . . . . . . . . . . . . . . . . . . . . . . . . . . . . . . . . .5.1.3. Heavy neutral lepton mixing . . . . . . . . . . . . . . . . . . . . . . . . . . . . . . . . . . . . . . . . . . . . . . . . . . . . . . . . . . . . . .5.2. Studies of low-energy QCD . . . . . . . . . . . . . . . . . . . . . . . . . . . . . . . . . . . . . . . . . . . . . . . . . . . . . . . . . . . . . . . . . . . . . .5.2.1. Le e annihilation . . . . . . . . . . . . . . . . . . . . . . . . . . . . . . . . . . . . . . . . . . . . . . . . . . . . . . . . . . . . . . . . . . . . . . . .5.2.2. W Z -photon scattering . . . . . . . . . . . . . . . . . . . . . . . . . . . . . . . . . . . . . . . . . . . . . . . . . . . . . . . . . . . . . . . . . .5.3. Conclusions on rare and exotic processes at MCs . . . . . . . . . . . . . . . . . . . . . . . . . . . . . . . . . . . . . . . . . . . . . . . . . .6. Charm decay physics . . . . . . . . . . . . . . . . . . . . . . . . . . . . . . . . . . . . . . . . . . . . . . . . . . . . . . . . . . . . . . . . . . . . . . . . . . . . . . . .6.1. Introduction . . . . . . . . . . . . . . . . . . . . . . . . . . . . . . . . . . . . . . . . . . . . . . . . . . . . . . . . . . . . . . . . . . . . . . . . . . . . . . . . . . . .6.2. Theoretical motivation for charm physics . . . . . . . . . . . . . . . . . . . . . . . . . . . . . . . . . . . . . . . . . . . . . . . . . . . . . . . . . .6.3. Probing strong interactions through charm decays . . . . . . . . . . . . . . . . . . . . . . . . . . . . . . . . . . . . . . . . . . . . . . . . . . .6.3.1. Absolute charm branching ratios . . . . . . . . . . . . . . . . . . . . . . . . . . . . . . . . . . . . . . . . . . . . . . . . . . . . . . . . . . .6.3.2. Ds ; D ; . . . . . . . . . . . . . . . . . . . . . . . . . . . . . . . . . . . . . . . . . . . . . . . . . . . . . . . . . . . . . . . . . . . . . . .6.3.3. Inclusive charm hadron decays . . . . . . . . . . . . . . . . . . . . . . . . . . . . . . . . . . . . . . . . . . . . . . . . . . . . . . . . . . . .6.4. Searches for new physics in charm decays . . . . . . . . . . . . . . . . . . . . . . . . . . . . . . . . . . . . . . . . . . . . . . . . . . . . . . . . .06.4.1. D0 –DL oscillations . . . . . . . . . . . . . . . . . . . . . . . . . . . . . . . . . . . . . . . . . . . . . . . . . . . . . . . . . . . . . . . . . . . . . . .6.4.2. CP violation in D decays . . . . . . . . . . . . . . . . . . . . . . . . . . . . . . . . . . . . . . . . . . . . . . . . . . . . . . . . . . . . . . . . .6.4.3. T odd correlations in c decays . . . . . . . . . . . . . . . . . . . . . . . . . . . . . . . . . . . . . . . . . . . . . . . . . . . . . . . . . . .6.5. Summary on charm decay physics at MCs . . . . . . . . . . . . . . . . . . . . . . . . . . . . . . . . . . . . . . . . . . . . . . . . . . . . . . . .7. Neutrino oscillation experiments with a muon storage ring neutrino factory . . . . . . . . . . . . . . . . . . . . . . . . . . . . . . . . .7.1. Status of neutrino oscillations at the time of MCs . . . . . . . . . . . . . . . . . . . . . . . . . . . . . . . . . . . . . . . . . . . . . . . . .7.2. Oscillation experiments at MCs . . . . . . . . . . . . . . . . . . . . . . . . . . . . . . . . . . . . . . . . . . . . . . . . . . . . . . . . . . . . . . . . .7.3. Matter eJects . . . . . . . . . . . . . . . . . . . . . . . . . . . . . . . . . . . . . . . . . . . . . . . . . . . . . . . . . . . . . . . . . . . . . . . . . . . . . . . . . .7.4. Detector considerations . . . . . . . . . . . . . . . . . . . . . . . . . . . . . . . . . . . . . . . . . . . . . . . . . . . . . . . . . . . . . . . . . . . . . . . . . .7.4.1. Magnetized sampling calorimeters . . . . . . . . . . . . . . . . . . . . . . . . . . . . . . . . . . . . . . . . . . . . . . . . . . . . . . . . . .7.4.2. Liquid argon TPCs . . . . . . . . . . . . . . . . . . . . . . . . . . . . . . . . . . . . . . . . . . . . . . . . . . . . . . . . . . . . . . . . . . . . . . .7.4.3. Muon detector conclusions . . . . . . . . . . . . . . . . . . . . . . . . . . . . . . . . . . . . . . . . . . . . . . . . . . . . . . . . . . . . . . . .7.4.4. Tau and electron detectors . . . . . . . . . . . . . . . . . . . . . . . . . . . . . . . . . . . . . . . . . . . . . . . . . . . . . . . . . . . . . . . .7.5. Conclusions on neutrino oscillation studies at MCs . . . . . . . . . . . . . . . . . . . . . . . . . . . . . . . . . . . . . . . . . . . . . . . .8. Summary . . . . . . . . . . . . . . . . . . . . . . . . . . . . . . . . . . . . . . . . . . . . . . . . . . . . . . . . . . . . . . . . . . . . . . . . . . . . . . . . . . . . . . . . . .Acknowledgements . . . . . . . . . . . . . . . . . . . . . . . . . . . . . . . . . . . . . . . . . . . . . . . . . . . . . . . . . . . . . . . . . . . . . . . . . . . . . . . . . . . . .References . . . . . . . . . . . . . . . . . . . . . . . . . . . . . . . . . . . . . . . . . . . . . . . . . . . . . . . . . . . . . . . . . . . . . . . . . . . . . . . . . . . . . . . . . . . 2232232242242242252252251. Overview1.1. IntroductionMuon colliders have been proposed to provide lepton–lepton collisions while circumventing theenergy limitations on electron–positron storage rings caused by synchrotron radiation. The largermuon mass suppresses synchrotron radiation energy losses by a factor m4e m4 5 10 10 relative to
154I. Bigi et al. / Physics Reports 371 (2002) 151 – 230those of a circulating electron beam of the same energy and, incidentally, also opens up promisingpossibilities for s-channel Higgs boson production [1].Recent feasibility and design studies for future muon colliders [2,1] have begun to focus attentionon the exciting physics possibilities for experiments using neutrino beams from the decays of thecirculating high-energy muons. This report explores the potential for a “neutrino experiment at amuon collider”, or MC for short. A MC program could operate either parasitically during a colliding beam experiment, or it could be installed as part of a program in neutrino physics at a dedicatedmuon storage ring.Amongst the potential physics topics for MCs, neutrino oscillations have garnered the mostintense experimental and theoretical activity, and particular possibilities for long baseline oscillationexperiments exploiting a muon storage ring are covered elsewhere [3,4]. In this report, we wishto also highlight the superb capabilities of neutrinos as probes of the strong and weak interactiondynamics of quarks and the parton structure of nucleons, as well as the power of a MC in searchesfor evidence of new types of weak interactions.The remainder of this section lays out the expected experimental parameters and capabilities of a MC and provides concise overviews for the more detailed physics discussions that follow.1.2. Experimental overview1.2.1. High-current muon storage ringsRecent ideas for neutrino experiments at either muon colliders [5,6] or dedicated neutrino factories[7] represent reincarnations of earlier proposals for neutrino experiments at muon storage rings thatdate back at least to the 1960s. The essential advantages of modern MCs derive from the verylarge muon currents that might be produced and stored using the technologies developed for muoncolliders. Current design scenarios for muon colliders [2,1] and neutrino factories [10,4] envision oforder 1021 positive and negative muons per year circulating and decaying in the storage ring.Neutrinos from decays in the longest straight sections of the storage ring will emerge in intensecollinear beams that are highly suitable for experiments. Beams from such production straight sectionsshould provide many orders of magnitude higher event rates than considered in the early versionsof muon storage rings and, indeed, should be considerably more intense than today’s “conventional”neutrino beams produced from K decays. No need exists for a conventional beam’s muon shieldingberm, and detectors can be placed relatively close to the end of the production straight section.Coupled with the relativistic kinematics of muon decay, this permits the possibility of detectors onlytens of centimeters across and allows for the use of high-precision vertex detectors as active neutrinotargets.Additional physics advantages over K decay neutrino beams will result from the unique and precisely understood Pux composition of the MC beams. Negative and positive muons decay accordingto Le e ; L e e ;(1)
I. Bigi et al. / Physics Reports 371 (2002) 151 – 230155producing pure 2-component neutrino beams 1 via perhaps the best understood of all weak decayprocesses. These beams will be designated as Le or L e , respectively, in the rest of this report.Experimental requirements for the two broad classes of neutrino physics at MCs diJer greatly,chiePy because the experiments would be conducted at very diJerent baseline distances from beamproduction to the detector. Experiments for neutrino interaction physics will be conducted as closeto the muon ring as possible (“short baseline”) in order to maximize event rates and to subtendthe neutrino beam with a target of small transverse cross section. On the other hand, the choice ofbaseline for neutrino oscillation studies will be dictated by the speci/c range of possible oscillationparameters under investigation, as discussed further in Section 7. Oscillation parameters of currentinterest motivate the use of very long baselines, even extending to the possibility of transcontinentalexperiments [7].As an important caveat on the contents of this report, it should always be borne in mind that theambitious technologies of these high-current muon storage rings still only exist at the feasibility orearly design study stage and it is by no means guaranteed that realizable devices will appear anytimesoon. Nevertheless, recent progress has been impressive, and the pace of R& D is accelerating. Thereader is referred to the specialist literature for a more thorough overview of the technologicalchallenges in building a muon collider [2,1] or neutrino factory [10,4].1.2.2. Event ratesEvent rates in all MC experiments will be dominated by the charged current (CC) and neutralcurrent (NC) deep inelastic scattering (DIS) of neutrinos or antineutrinos with nucleons (N p orn): ‘ N ‘ X( ‘ -CC) ; L‘ N ‘ X( L‘ -CC) ; ‘ ( L‘ ) N ‘ ( L‘ ) X( ‘ ( L‘ )-NC) ;(2)where ‘ e or and X represents a typically multi-particle hadronic /nal state. Neutrino–nucleonDIS cross sections scale with neutrino energy E to a good approximation for neutrino energiesabove a few GeV, with numerical values of [12] -CC6:8 -NC 2:1 E (GeV) :(3) N (fb) L -CC 3:4 -NCL1:3At the many-GeV energies of MCs, N DIS is well described as the quasi-elastic scattering ofneutrinos oJ one of the many quarks or antiquarks inside the nucleon through the exchange of1We implicitly assume here the absence of a signi/cant lepton family number violating decay of the type e e L but caution that the current experimental limit on the branching fraction for this decay is only 1.5%. This limit will clearlybe greatly improved upon from the consistency of the observed MC spectra with predictions.
156I. Bigi et al. / Physics Reports 371 (2002) 151 – 230a virtual W or Z boson: ‘ ( L‘ ) q ‘ ( L‘ ) q(NC) ;(4) ‘ qd (qLu ) ‘ qu (qL d )( -CC) ;(5) L‘ qu (qLd ) ‘ qd (qL u )( -CC)L:(6)All quarks q participate in the NC process. The CC interactions change quark Pavor, with neutrinoLinteractions producing u- and d-type/nal state quarks, qu and qL d , from d- and u-typeLtargets, qdand qLu . Antineutrinos participate in the charge-conjugate processes. Much of the richness of neutrinointeraction physics derives from the variety of processes contained in Eqs. (4) – (6).1.2.3. Neutrino production spectra and event rates in detectorsNeutrino Pux spectra at MCs will be precisely predictable since the decay of muons is awell-understood purely electroweak process. Characteristics of the parent muon beam in the production straight section can be reliably calculated and modeled through a knowledge of the focusingmagnet lattice and through beam monitoring. Calibration of the muon energies in the storage ringmight reach the level of a few parts per million fractional uncertainty [11].Due to the diJering angular coverages, the neutrino spectrum seen by an oscillation detector ata long baseline will diJer from that seen by detectors placed at short baselines to study interactionphysics. Long-baseline detectors will sample the very forward-going neutrinos, at angles in the muonrest frame ( ) and laboratory frame ( ) close to 0, while detectors close to the productionstraight section will instead accept a production solid angle bite that is comparable to the boostedforward hemisphere of the decaying neutrinos: 2 sin 1 ! m c 210 4: E E (TeV)(7)Fig. 1 gives an illustrative example of the neutrino spectra at MCs for detectors at both shortand long baselines [10], and Table 1 gives beam and event rate parameters for several other MCscenarios. Further explanation for the choices of storage ring parameters in Table 1 and a derivationfor the following simple numerical expressions for event rates used to /ll Table 2 are providedelsewhere [9].For short-baseline detectors,N sb (events yr 1 g 1 cm2 ) 2:1 10 15 E (GeV) N ss (yr 1 ) ;(8)where N sb is the number of neutrino interactions per year of running per g cm2 of a cylindricallysymmetric target centered on the beam, E is the muon beam energy and N ss is the number offorward-going muons (as opposed to muons circulating in the opposite direction in, e.g., a colliderring) decaying in the production straight section per year. Eq. (8) assumes the parent muon beam tohave an angular divergence in the production straight section that is small compared to the " 1 !natural divergence of the neutrino beam. This will normally be the case [13] unless the choice ofstraight section is in the /nal focus region of a collider storage ring. Table 2 accounts simplisticallyfor this exception (in this case for the illustrative parameter set at 500 GeV) by increasing theangular divergence of the neutrino beam by a simple scale factor. In this circumstance, the angular
I. Bigi et al. / Physics Reports 371 (2002) 151 – 230157Neutrino Fluxes from a 50 GeV Muon Beamνµθ 0οθ 90νeneutrino energy, GeVFig. 1. Example neutrino event spectra for and Le from a 50 GeV negative muon beam from a neutrino factory [10].Solid curves indicate the spectra for decays at zero degrees in the center of mass system. This is the spectrum expectedfor a detector located very far from the muon decay region. The dashed curves indicate the spectra for decays within theforward hemisphere in the center of mass frame. This is what would be expected for a detector close enough to the muondecay region to subtend an angle of 1 !.coverage of the target would need to be increased by this same scale factor in order to retain theevent rate predicted by the parameter N sb .In contrast to short-baseline detectors, the event rate in long-baseline detectors is not sensitiveto the geometry of the detector since the entire detector will always be bathed uniformly by theforward-going neutrino Pux. The number of interactions in the detector will vary in proportion tothe target mass M and inversely as the square of the baseline length L. This leads to a de/nition,analogous to Eq. (8), for the event rate benchmark N lb :N lb (events yr 1 kt 1 (103 km)2 ) 1:6 10 20 N ss (yr 1 ) (E (GeV))3;(! " )2(9)where N lb is the number of neutrino interactions per kiloton per year of running with a targetcentered on the beam at a 1000 km distance from the production point. The previously discussedangular divergence scaling factor, ! " , has been explicitly included.
158I. Bigi et al. / Physics Reports 371 (2002) 151 – 230Table 1Neutrino Puxes and event rates for representative example parameter sets for dedicated neutrino factories or muon colliders[9] spanning the energy range E 20 GeV–5 TeV. The angular divergence scaling factor, ! " , is the factor by whichthe divergence of the parent muon beam increases the neutrino beam’s angular divergence beyond the characteristic size," 1 !, expected for a divergenceless muon beamDescription -FactoryHiggs factoryTop factoryMuon energy E yr (N 1020 )Flight time to beam dump (tD ! )Ring circumference C (m)Straight section (SS) length lss (m)Fractional SS length (fss lss C) yr in SS (N ss fss N 1020 ) from SS yr ( 1020 ) angular divergence ( ! " ) angular divergence (mrad)N sb (events yr g cm2 )Target thickness (g cm2 ) for 1010 eventsN lb [events yr kt (103 km)2 ]20 GeV3.0No dump300900.300.901.815.33:8 10626001:2 10450 GeV6.0No dump345400.120.721.412.16:5 10615001:4 105175 GeV6.0No dump9001100.120.721.410.602:7 1073706:2 106DescriptionFrontier2nd GenerationMuon energy E yr (N 1020 )Flight time to beam dump (tD ! )Ring circumference C (m)Straight section (SS) length lss (m)Fractional SS length (fss lss C) yr in SS (N ss fss N 1020 ) from SS yr ( 1020 ) angular divergence ( ! " ) angular divergence (mrad)N sb (events yr g cm2 )Target thickness (g cm2 ) for 1010 eventsN lb [events yr kt (103 km)2 ]500 GeV3.20.520001500.120.380.30102.12:3 1074305:0 1055 TeV3.6No dump15 0004500.030.110.2210.0211:0 1081002:2 1010The event rates given in Table 2 are truly impressive. Samples of thousands of events per kilotonmight be recorded at oscillation experiments with baselines as long as thousands of kilometers. Forneutrino interaction physics, samples as large as 10 billion events can be reasonably contemplatedin compact targets close to the production straight section. Eq. (7) shows that the radial extent ofsuch targets can be as small as 10 –20 cm.1.2.4. Detector design considerations for MCsEvent rates for oscillation experiments will probably be less of an extrapolation from today’sexperiments than will be the case for interaction experiments due to the compensating rate decrease atthe expected longer baselines. Correspondingly, the innovations in neutrino detector design required
I. Bigi et al. / Physics Reports 371 (2002) 151 – 230159Table 2Speci/cations, integrated luminosities and event rates for the high-rate neutrino targets discussed in this report, assumingthe 50 and 175 GeV muon storage ring parameters of Table 1. The target is assumed to be situated 100 m (350 m)downstream from the center of the 50 GeV (175 GeV) production straight sectionTarget purposeGeneralPolarized eMaterialMean density
the neutrino beam with a target of small transverse cross section. On the other hand, the choice of baseline for neutrino oscillation studies will be dictated by the specic range of possible oscillation parameters under investigation, as discusse