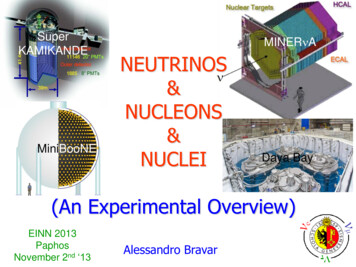
Transcription
UCLEIDaya Bay(An Experimental Overview)EINN 2013PaphosNovember 2nd ‘13Alessandro Bravar
Neutrinos In the “Current” Standard ModelStandard Model :using 19 parameters the SMpredicts the interactions ofelectroweak and strong forces,the properties of 12 fermions,and 12 bosons carrying the forceneutrinos : 3 types (flavors) lefthanded only neutral fermions– interact only weakly all have equal (weak)interactions assumed massless in the SM
But Where They Are ?Neutrinos are naturally produced in the Sun, the atmosphere, earth, our bodies, They can be also “fabricated” in nuclear reactors or by accelerators,
Neutrino SourcesGlashow resonancene e nm mne e ne e
Neutrino Physics SituationEspecially since 1998, neutrino physics has made great progress– discovery of oscillation (nm disappearance) in atmospheric n by SK (1998)confirmation in accelerator nm beam by K2K (2004) / MINOS (2006)– ne disappearance ( nm/nt)established by solar neutrino measurements by SNO / SK (2002)confirmation in reactor n by KamLAND (2004)– ne appearance nm ne by T2K (2.5 s in 2011 and 7.5 s in 2013)q13 0 by DayaBay (2012)confirmed 3 flavor mixing picture of neutrinosSurprises ( Mysteries) are– neutrino has really finite (but small) mass:first evidence of deviations from Standard Model– neutrino has finite (but big) flavor mixing (unlike quarks)lepton flavor is violated
Neutrino Oscillation n oscillations are a quantum mechanical effect neutrino flavor eigenstates (e, m, t)are different from mass eigenstates (1, 2, 3)n U i 1, 2, 3ini propagation in time (& space) described by the free Hamiltonian neutrino oscillations: probability of observing a given n flavorwill vary with time (flavor changes to other flavor in flight) only occur when neutrinos have finite mass and mixnmtMu neutrinoTau neutrino
3 Flavor Mixing of NeutrinosFlavor eigenstatesnenmnt n e n 1 n m U P MNS n 2 n n t 3 m1Mass eigenstatesm2m3Pontecorvo-Maki-Nakagawa-Sakata Matrix (CKM matrix in lepton sector)q23 450 60SuperK (atm. n)K2K / MinosT2Kq13 9.10 0.60Daya BayRenoT2Kq12 33.60 1.00solar nKamLANDfuture solar n exp.6 independent parameters govern oscillationq12,q23,q13,dcp,Dm122,D m232,neutrinolessdouble betadecayDm132
Neutrinos Oscillations In Time Evolution(three flavor oscillations)nmneEnmνntn (t ) U i en (t 0) U i n ii2ne iEi tnimi2Ei p 2pi U i U i U i U i U i U i e2iPm enttt 0P n (t ) n (t 0)ne-i(Ei -E j ) ti j2 Dm2a22222 31 L 4C13 S13 S 23 sin1 2S13 ) 1 2 4 E Dm31 leading, q13 driven22Dm32LDm321 LDm21L 8C13 S12 S13 S 23 (C12C23 cos d S12 S13 S23 ) cossins inCPC4E4E4E222Dm32LDm31LDm21L2CPV 8C13 C12C23 S12 S13 S 23 sin d sinsinsin4E4E4E222222222 Dm21 L 4 S12 C13 (C12 C23 S12 S 23 S13 2C12C23 S12 S 23 S13 cos d ) sinsolar4E2Dm32LDm321 L2222 aL 8C13 S13 S23 (1 2 S13 )cossinmatter effect4E4E4E2
Two flavor Oscillation in Vacuum(to make it simple) cos qU sin qFor two flavors, n and n ,the mixing matrix reduced tosin q cos q then the oscillation probability P(n n ) is given by ( Ei E j )t P(n n ) sin 2q sin 2 mi2Making the approximation Ei p 2p22(and including the factors h and c)the oscillation probability becomes L [km]22 P(n n ) sin 2q sin 1.27Dm [eV ] E [GeV] 2maximum oscillation amplitude2L : distance ν- sourceE : ν-energy at t 0 (source)oscillationfrequency
Present Knowledgen3atmo.solarreactorn2ORn1dCP unkownwhich ? both ordering allowed by data0.14big difference w.r.t. CKM matrix
Today’s Questions In Neutrino Physics Mass hierarchywe do not know if the neutrino n1 (contains more ne) is the lightest one or not Long baseline accelerator neutrino experiments Is CP symmetry violated ?help solve origin of matter-antimatter asymmetry in universe (leptogenesis) Long baseline accelerator neutrino experiments Absolute neutrino mass Tritium beta decay spectrum neutrino-less double beta decay Existence of sterile neutrinos Neutrino is Dirac ? or Majorana ? neutrino-less double beta decayUnraveling full nature of neutrino could provide breakthroughto approach our goals in particle physics
How Do We Make an Oscillation Experiment1. measure n spectrum at near detector before oscillations2. make prediction at far detector assuming no oscillations expFD POSC RF / N obsNDs FD En ) eff ND #ev FD En ) POSC En ) #ev ND En ) RF / N s ND En ) eff FD3. compare measured n spectrum at far detector with predictions (2)deviations ? oscillationsdoes not cancel 4. extract oscillation parametersbecause different spectra !nm disappearancene disappearancesin 2 2q13 0.150 0.0390.034q 23 450 60 (90%C.L.)20.12Dm32 2.32 0.08 10 3 eV 2Dm2sinq
Why Neutrino Cross–Sections ?existing n scattering data ( 1 – 20 GeV) poorly understoodmainly (old) bubble chamber datalow statistics sampleslarge uncertainties on n fluxneed detailed understanding of nm and anti-nm cross sectionsn oscillationprecision neutrino oscillation measurementsall experiments use dense nuclear targets (CH, H20, Ar, Fe, ) additional complications whose impact needs to be understoodbackgrounds (i.e. NC p0’s)neutrinos – weak probe of nuclear (low E) and hadronic (high E) structureelastic :axial form factors of the nucleoninclusive : quark structure of the nucleon (parton distribution functions)nucleons are confined in nuclei and are not free expect deviations from n – free nucleon (p or n) interactions quark densities modifications in nuclei (EMC effect)(today we have very high intensity neutrino beams that allow us to study all this)
n -sections
T2K ND280 Off-Axis Event Gallery15
Probing Nucleon StructureCharged lepton scattering data show that quark distributions are modifiedin nucleons confined (bound) in a nucleus:PDFs of a nucleon within a nucleus are different from PDFs of a free nucleon.The EMC effect (valence region) does not shows a strong A dependence for F2A / F2Dn probes same quark flavorsas charged leptons butwith different “weights” expect different shape expect different behavior ? x 1? is shadowing the same ?F2A / F2DNuclear effects in neutrino scattering are not well established, and have not beenmeasured directly : experimental results to datehave all involved one target materialA / D Ratio (e / m DIS)per experiment (Fe or Pb or ).Should be studied using D targets.anti-shadowingshadowingEMC effectxBj
How To Make a nm Beam(conventional horn focused beam)FocusingDevicesProton TargetBeamDecay PipemnmSimon van der Meerp(1925 2011)Focusing device: Electromagnetic HornBeam DumpAluminumCurrentp beamBBp p I [ A] 10 65r [m]B 4.3 T , r 15 mm, I 320kAトロイダル磁場B [T ] pure nm beam ( 99%)ne ( 1%) from p m e chain and K decays (Ke3)nm / nm can be switched by flipping polarity of Horns
The NUMI Beam (FNAL)NuMI (Neutrinos at the Main Injector)120 GeV protons from Main Injector, 300 - 350 kW90 cm graphite target675 m decay tunnelBy moving the production target w.r.t. 1st hornand the distance between horn 1 and horn 2one can modify the n spectrum:LE (peak 3 GeV) ME (peak 6 GeV)Flux determinationmuon monitor dataspecial runs (vary beam parameters)nm – electron scatteringlow–n methodexternal hadron production data
The Off-Axis T2K n BeamDm2 3x10-3eV2qTarget 3 Horns Decay Pipe2.50p decay KinematicsVery narrow energy spectrum(almost monochromatic beam)Neutrino beam energy “tuned” tooscillation maximumEn (GeV)0 Enmax [GeV] 2 nm fluxSuper-K.OA0 OA2 OA2.5 OA3 30q [mrad ]neutrino energy En almost independentof parent pion energy2.5 3 horn focusing cancels partially the pTdependence of the parent pionpp (GeV/c)
Quasi-Elastic ScatteringEnQE mn2 (m p Eb ) 2 mm2 2(m p Eb ) Em2(m p Eb Em pm cos m ) 2QQE mm2 2 EnQE Em Em2 mm2 cos mEREC ETRUE ?QREC QTRUE ?)
n CCQE scatteringconsidered a possible standard candle for n oscillation experiments (En 1 GeV)En and Q2 can be determined from outgoing m energy and angleMiniBooNENOMADRelativistic Fermi Gasmodel axial form factorsT. Katori 30% discrepancy in the QE x-section measurements between recent exp.identification of QE events (purity, backgrounds, )reconstructed En energyaxial mass MAnuclear effects, FSI, two body currents (MEC), tension between datasets and RFG model : increase MA in the axial FF ?
And If Experiments “Do Not Agree” ?NOMAD data consistent with “standard” QE prediction (with MA 1.0 GeV)MiniBooNE data is well above “standard” QE prediction ( 30%)(increasing MA 1.35 can reproduce s)
And If Experiments “Do Not Agree” ?or how neutrino physicists discovered nuclear physicsrecognize thatnucleons are not freein a nucleus(the RFG model doesnot work)many models and authorsincluding nuclear effects :correlations (SRC)two body currents (MEC)2p2hTEMFSI MA 1 GeV
What About En ?input En Dirac deffects of1) single nucleon(RFG smearing)2) multi nucleon scattering(20 -30% of events off correlated pairs)mN Em mm2 2QEQEEn EREC mN Em pm cos m
MiniBooNE @ FNALLiquid Scintillator CH2 target4π detector, complete angular coverageGood lepton reconstruction & pion rejectionEssentially blind to details of the nucleon final state in CC eventsDetect both scintillating light and Cherenkov light
n / anti-n CCQE -Sections d2s/dTmdcosqmflux averaged doubly differential cross sectionslargely model independent measurement of muon kinematicsOlder experimental data is consistent with dipole axial FF and MA 1.015 GeV.New data also described with dipole axial FF but require MA 1.35 GeVOld resonance scattering data (e.g. via D production) MA 1.3 GeV
anti-n d2s/dTmdcosqmTm (GeV)It is clear that the RFG model assumingMA 1 GeV does not adequatelydescribe these data in shape or innormalization.PRD 88, 032001 (2013)
The MINERnA Detector120 plastic scintillator modules for tracking and calorimetry ( 32k readout channels)Construction completed in Spring 2010. He and H20 targets added in 2011MINOS Near Detector serves as muon spectrometer (limited acceptance)nuclear targets: He, C, H20, Fe, Pbfully active scintillator tracker
9” H20625 kgNuclear TargetsActive Scintillator ModulesLiquid He250 kgWaterHe1” Fe / 1” Pb322 kg / 263 kg1” Pb / 1” Fe263 kg / 321 kg3” C / 1” Fe / 1” Pb160 kg / 158 kg / 107 kgTrackingRegion0.3” Pb225 kg.5” Fe / .5” Pb162 kg / 134 kg
n CCQE Events in MINERnAMeVVertex Energynm p m nRecoil EnergyRegionTRACKERn BeamECALModule number nm n m pHCALMINOS NDVertex EnergyRecoil EnergyRegionTRACKERECALHCAL
anti-n / n CCQE -Sections ds/dQ2(flux averaged)RFG, SFRFG, SFMA 1.35MA 1.35TEMTEM
Conclusions CCQERecent CCQE measurements on nuclear materials are consistent :a significant enhancement in the normalizationthat grows with decreasing muon scattering angleis observed compared to the expectation with MA 1.0 GeV.1) a significant enhancement ( 30%) in the normalization2) a significant deficit of events is observed at low Q2 (Q2 0.1 GeV2)3) a significant excess of events is observed at larger Q2 (Q2 0.3 GeV2)The RFG model assuming MA 1 GeV does not adequatelydescribe these data in shape nor in normalizationThe interpretation of MINERnA data suggests thatthe resulting final-state pairs would be predominantly ppin neutrino scattering and nn in anti-neutrino scattering.(these results are consistent with the observation inquasi-elastic e – C scattering suggesting that multi-bodyfinal states are dominated by initial-state np pairs [JLab])
Inclusive Scatteringin principle ignore Xin reality need Xto reconstruct En calorimetricallyEn Em EXcross section build up: elastic 1p np K (“schematic”)
T2K CC Inclusive n ScatteringND280
T2K Off-Axis nm Analysis0.6 En 2 GeV tailOff-Axis ND280 detector
T2K CC Inclusive n cross sectionsdoubly differential flux averaged cross section d2s /dp dcosq
T2K CC Inclusive n cross sections
Minerna Inclusive n –sectionsMINOS NDmatchedtrackEvent selection criteria:single muon track in MINERnAwell reconstructed and matched into MINOS NDreconstructed vertex inside fiducial tracker regionnuclear targets : z position consistent with nuclear targetrecoil energy EREC reconstructed calorimetrically :En Em EREC
An Event from Target 3CFePbviewlookingupstreamStrip Numbernm N m XModule Number
“Plastic” BackgroundProject the one track events to thepassive target’s center in zThis is the best guess of the vertexScintillator events wrongly accepted intopassive target sample are backgroundthese peaks are at thelocation of the firstmodule downstreamof the passive targetsTgt2Tgt3Tgt4Tgt5use events in the trackermodules to predict andsubtract the plasticbackground
Errors on absolutecross sectionsErrors on ratio ofcross sectionTaking ratios removes large uncertainties due to the neutrino fluxall targets in same beam flux largely cancels similar acceptance and reconstruction41
Cross Section Ratios – EnσCσCHσFeσCHσPbσCH“standard” MINERnA kinematical cuts2 neutrino energy 20 GeV0 muon angle 17 degNo evidence of tension betweenMINERnA data and GENIE 2.6.2event generator
Cross Section Ratios – xBjdσC/dxdσCH/dxdσPb/dxdσCH/dxObserve an excess at 0.7 x thatgrows with the size of the nucleusdσFe/dxdσCH/dxObserve a deficit for x 0.1 thatincreases with the size of the nucleusThese effects are not modeled inevent generator (GENIE) remove elastic-like events
b/dxdσCH/dx
Nuclear Modification Simulation in MINERnA“standard” GENIE modelBodek-Yang Model (2003)arXiv:hep-ex/0308007MINERnA modelsBodek-Yang Model (2013)arXiv:hep-ph/1011.6592Fit to charged lepton dataVery similar to widely used E139 fitAll nuclei has same modificationAll treated as isoscalar ironSpecific fits for C, Fe, Pb on CHNuclear modification fitfor iron to deuterium ratio
W–Q2 “acceptance” ME (2013–18)z axis : 103 events / 3 x 103 kg of C / 6e20POTSimulationGENIE 2.6.2DISRESCCQEkinematical distribution from GENIE 2.6.2 event generatorwith Minerna “standard” cuts (Em 2 GeV, qm 170)
Conclusion InclusiveFinally we have sufficiently intense neutrino beams to study in detailnucleon and nuclear structureFirst precise direct measurements of nuclear-dependence of neutrinocross sections in the few GeV regimeGood agreement with simulation as a function of EnDeficit increases with A for xBj 0.1 (Pb Fe C)Excess increases with A for xBj 0.7 (Pb Fe C)both effects are not modeled in event generatorsEnhanced statistics ( 10x) in higher energy, intensity NOvA-era beamhigher energy lower xBj reachmuch more DIS extract structure functions
OutlookNeutrino Physics is entering an era of precision measurementsPrecise knowledge and detailed understanding of n – Across sections required (sys. oscill. 1-2% for CPV !)(neutrino interaction simulation models rarely handle nuclearmodifications correctly)Today we have very high intensity neutrino beams that allow usto study n – nucleon and n – nucleus interactions in detailExpect several, new n – A cross section measurements in the1 – 20 GeV region in the next yearsNeutral Currents Ds(first understand nuclear effects, however times are mature fora dedicated experiment using (liquid) H and D targets)
Neutrino Physics Situation Especially since 1998, neutrino physics has made great progress – discovery of oscillation (nm . (EMC effect) (today we have very high intensity neutrino beams that allow us to study all