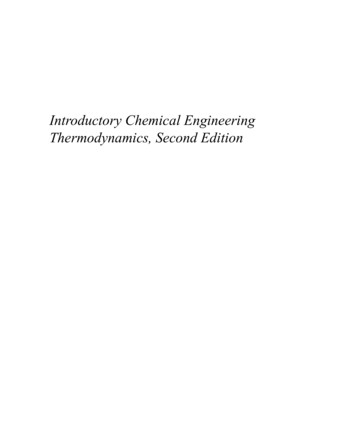
Transcription
Introductory Chemical EngineeringThermodynamics, Second Edition
Prentice Hall International Series in thePhysical and Chemical Engineering SciencesVisit informit.com/ph/physandchemfor a complete list of available publications.The Prentice Hall International Series in the Physical and ChemicalEngineering Sciences had its auspicious beginning in 1956 under the directionof Neal R. Amundsen. The series comprises the most widely adoptedcollege textbooks and supplements for chemical engineering education.Books in this series are written by the foremost educators and researchersin the field of chemical engineering.Make sure to connect with us!informit.com/socialconnect
Introductory Chemical EngineeringThermodynamics, Second EditionJ. Richard ElliottCarl T. Lira Upper Saddle River, NJ Boston Indianapolis San Francisco New York Toronto Montreal London Munich Paris Madrid Capetown Sydney Tokyo Singapore Mexico City
Many of the designations used by manufacturers and sellers to distinguish their products areclaimed as trademarks. Where those designations appear in this book, and the publisher was awareof a trademark claim, the designations have been printed with initial capital letters or in all capitals.The authors and publisher have taken care in the preparation of this book, but make no expressedor implied warranty of any kind and assume no responsibility for errors or omissions. No liabilityis assumed for incidental or consequential damages in connection with or arising out of the use ofthe information or programs contained herein.The publisher offers excellent discounts on this book when ordered in quantity for bulk purchasesor special sales, which may include electronic versions and/or custom covers and content particular to your business, training goals, marketing focus, and branding interests. For more information,please contact:U.S. Corporate and Government Sales (800) 382-3419 corpsales@pearsontechgroup.comFor sales outside the United States please contact:International Sales international@pearson.comVisit us on the Web: informit.comLibrary of Congress Cataloging-in-Publication DataOn file.Copyright 2012 Pearson Education, Inc.All rights reserved. Printed in the United States of America. This publication is protected by copyright, and permission must be obtained from the publisher prior to any prohibited reproduction,storage in a retrieval system, or transmission in any form or by any means, electronic, mechanical,photocopying, recording, or likewise. To obtain permission to use material from this work, pleasesubmit a written request to Pearson Education, Inc., Permissions Department, One Lake Street,Upper Saddle River, New Jersey 07458, or you may fax your request to (201) 236-3290.ISBN-13: 978-0-13-606854-9 ISBN-10:0-13-606854-5 Text printed in the United States at Hamilton in Castleton, New York. First printing, February 2012
CONTENTSPREFACENotes to StudentsAcknowledgmentsxviixviiixviiiABOUT THE AUTHORSxixGLOSSARYxxiNOTATIONxxvUNIT I FIRST AND SECOND LAWS1CHAPTER 1 BASIC CONCEPTS31.1 Introduction1.2 The Molecular Nature of Energy, Temperature, and PressureExample 1.1 The energy derived from intermolecular potentialsExample 1.2 Intermolecular potentials for mixtures1.3 The Molecular Nature of Entropy1.4 Basic Concepts1.5 Real Fluids and Tabulated PropertiesExample 1.3 Introduction to steam tablesExample 1.4 InterpolationExample 1.5 Double interpolationExample 1.6 Double interpolation using different tablesExample 1.7 Double interpolation using ExcelExample 1.8 Quality calculationsExample 1.9 Constant volume cooling1.6 Summary1.7 Practice Problems561214151522252727282931323334v
vi Contents1.8 Homework ProblemsCHAPTER 2 THE ENERGY BALANCE2.12.22.32.4Expansion/Contraction WorkShaft WorkWork Associated with FlowLost Work versus ReversibilityExample 2.1 Isothermal reversible compression of an ideal gas2.5 Heat Flow2.6 Path Properties and State PropertiesExample 2.2 Work as a path function2.7 The Closed-System Energy BalanceExample 2.3 Internal energy and heat2.8 The Open-System, Steady-State BalanceExample 2.4 Pump work for compressing H2O2.9 The Complete Energy Balance2.10 Internal Energy, Enthalpy, and Heat CapacitiesExample 2.5 Enthalpy change of an ideal gas: Integrating CPig(T)Example 2.6 Enthalpy of compressed liquidExample 2.7 Adiabatic compression of an ideal gas in a piston/cylinder2.11 Reference StatesExample 2.8 Acetone enthalpy using various reference states2.12 Kinetic and Potential EnergyExample 2.9 Comparing changes in kinetic energy, potential energy, internal energy, and enthalpyExample 2.10 Transformation of kinetic energy into enthalpy2.13 Energy Balances for Process Equipment2.14 Strategies for Solving Process Thermodynamics Problems2.15 Closed and Steady-State Open SystemsExample 2.11 Adiabatic, reversible expansion of an ideal gasExample 2.12 Continuous adiabatic, reversible compression of an ideal gasExample 2.13 Continuous, isothermal, reversible compression of an ideal gasExample 2.14 Heat loss from a turbine2.16 Unsteady-State Open SystemsExample 2.15 Adiabatic expansion of an ideal gas from a leaky tankExample 2.16 Adiabatically filling a tank with an ideal gasExample 2.17 Adiabatic expansion of steam from a leaky tank2.17 Details of Terms in the Energy Balance2.18 Summary2.19 Practice Problems2.20 Homework ProblemsCHAPTER 3 ENERGY BALANCES FOR COMPOSITE SYSTEMS3.1 Heat Engines and Heat Pumps – The Carnot CycleExample 3.1 Analyzing heat pumps for housing3.2 Distillation ColumnsExample 3.2 Start-up for a distillation column3.3 Introduction to Mixture Properties3.4 Ideal Gas Mixture 3105106
Contents3.5 Mixture Properties for Ideal SolutionsExample 3.3 Condensation of a vapor stream3.6 Energy Balance for Reacting SystemsExample 3.4 Stoichiometry and the reaction coordinateExample 3.5 Using the reaction coordinates for simultaneous reactionsExample 3.6 Reactor energy balances3.7 Reactions in Biological Systems3.8 Summary3.9 Practice Problems3.10 Homework ProblemsCHAPTER 4 ENTROPY4.1 The Concept of Entropy4.2 The Microscopic View of EntropyExample 4.1 Entropy change and “lost work” in a gas expansionExample 4.2 Stirling’s approximation in the Einstein solid4.3 The Macroscopic View of EntropyExample 4.3 Adiabatic, reversible expansion of steamExample 4.4 A Carnot cycle based on steamExample 4.5 Ideal gas entropy changes in an adiabatic, reversible expansionExample 4.6 Ideal gas entropy change: Integrating CPig(T)Example 4.7 Entropy generation and “lost work”Example 4.8 Entropy generation in a temperature gradient4.4 The Entropy BalanceExample 4.9 Entropy balances for steady-state composite systems4.5 Internal Reversibility4.6 Entropy Balances for Process EquipmentExample 4.10 Entropy generation by quenchingExample 4.11 Entropy in a heat exchangerExample 4.12 Isentropic expansion in a nozzle4.7 Turbine, Compressor, and Pump Efficiency4.8 Visualizing Energy and Entropy Changes4.9 Turbine CalculationsExample 4.13 Various cases of turbine outlet conditionsExample 4.14 Turbine efficiency calculationExample 4.15 Turbine inlet calculation given efficiency and outlet4.10 Pumps and CompressorsExample 4.16 Isothermal reversible compression of steamExample 4.17 Compression of R134a using P-H chart4.11 Strategies for Applying the Entropy Balance4.12 Optimum Work and Heat TransferExample 4.18 Minimum heat and work of purification4.13 The Irreversibility of Biological Life4.14 Unsteady-State Open SystemsExample 4.19 Entropy change in a leaky tankExample 4.20 An ideal gas leaking through a turbine (unsteady state)4.15 The Entropy Balance in Brief4.16 Summary4.17 Practice 85187
viii Contents4.18 Homework ProblemsCHAPTER 5 THERMODYNAMICS OF PROCESSES5.1 The Carnot Steam Cycle5.2 The Rankine CycleExample 5.1 Rankine cycle5.3 Rankine ModificationsExample 5.2 A Rankine cycle with reheatExample 5.3 Regenerative Rankine cycle5.4 RefrigerationExample 5.4 Refrigeration by vapor compression cycle5.5 LiquefactionExample 5.5 Liquefaction of methane by the Linde process5.6 Engines5.7 Fluid Flow5.8 Problem-Solving Strategies5.9 Summary5.10 Practice Problems5.11 Homework 214215215216UNIT II GENERALIZED ANALYSIS OF FLUID PROPERTIES223CHAPTER 6 CLASSICAL THERMODYNAMICS — GENERALIZATIONS FOR ANY FLUID2256.1 The Fundamental Property Relation6.2 Derivative RelationsExample 6.1 Pressure dependence of HExample 6.2 Entropy change with respect to T at constant PExample 6.3 Entropy as a function of T and PExample 6.4 Entropy change for an ideal gasExample 6.5 Entropy change for a simple nonideal gasExample 6.6 Accounting for T and V impacts on energyExample 6.7 The relation between Helmholtz energy and internal energyExample 6.8 A quantum explanation of low T heat capacityExample 6.9 Volumetric dependence of CV for ideal gasExample 6.10 Application of the triple product relationExample 6.11 Master equation for an ideal gasExample 6.12 Relating CP to CV6.3 Advanced Topics6.4 Summary6.5 Practice Problems6.6 Homework 244247248248CHAPTER 7 ENGINEERING EQUATIONS OF STATE FOR PVT PROPERTIES2517.1 Experimental Measurements7.2 Three-Parameter Corresponding States7.3 Generalized Compressibility Factor Charts252253256
ContentsExample 7.1 Application of the generalized charts7.4 The Virial Equation of StateExample 7.2 Application of the virial equation7.5 Cubic Equations of State7.6 Solving the Cubic Equation of State for ZExample 7.3 Peng-Robinson solution by hand calculationExample 7.4 The Peng-Robinson equation for molar volumeExample 7.5 Application of the Peng-Robinson equation7.7 Implications of Real Fluid BehaviorExample 7.6 Derivatives of the Peng-Robinson equation7.8 Matching the Critical PointExample 7.7 Critical parameters for the van der Waals equation7.9 The Molecular Basis of Equations of State: Concepts and NotationExample 7.8 Estimating molecular sizeExample 7.9 Characterizing molecular interactions7.10 The Molecular Basis of Equations of State: Molecular SimulationExample 7.10 Computing molecular collisions in 2DExample 7.11 Equations of state from trends in molecular simulations7.11 The Molecular Basis of Equations of State: Analytical TheoriesExample 7.12 Deriving your own equation of state7.12 Summary7.13 Practice Problems7.14 Homework ProblemsCHAPTER 8 DEPARTURE FUNCTIONS8.1 The Departure Function Pathway8.2 Internal Energy Departure FunctionExample 8.1 Internal energy departure from the van der Waals equation8.3 Entropy Departure Function8.4 Other Departure Functions8.5 Summary of Density-Dependent Formulas8.6 Pressure-Dependent Formulas8.7 Implementation of Departure FormulasExample 8.2 Real entropy in a combustion engineExample 8.3 Compression of methane using the virial equationExample 8.4 Computing enthalpy and entropy departures from the Peng-Robinson equationExample 8.5 Enthalpy departure for the Peng-Robinson equationExample 8.6 Gibbs departure for the Peng-Robinson equationExample 8.7 U and S departure for the Peng-Robinson equation8.8 Reference StatesExample 8.8 Enthalpy and entropy from the Peng-Robinson equationExample 8.9 Liquefaction revisitedExample 8.10 Adiabatically filling a tank with propane8.9 Generalized Charts for the Enthalpy Departure8.10 Summary8.11 Practice Problems8.12 Homework 309310310312314316317317318320320322323323325326
x ContentsCHAPTER 9 PHASE EQUILIBRIUM IN A PURE FLUID9.1 Criteria for Phase Equilibrium9.2 The Clausius-Clapeyron EquationExample 9.1 Clausius-Clapeyron equation near or below the boiling point9.3 Shortcut Estimation of Saturation PropertiesExample 9.2 Vapor pressure interpolationExample 9.3 Application of the shortcut vapor pressure equationExample 9.4 General application of the Clapeyron equation9.4 Changes in Gibbs Energy with Pressure9.5 Fugacity and Fugacity Coefficient9.6 Fugacity Criteria for Phase Equilibria9.7 Calculation of Fugacity (Gases)9.8 Calculation of Fugacity (Liquids)Example 9.5 Vapor and liquid fugacities using the virial equation9.9 Calculation of Fugacity (Solids)9.10 Saturation Conditions from an Equation of StateExample 9.6 Vapor pressure from the Peng-Robinson equationExample 9.7 Acentric factor for the van der Waals equationExample 9.8 Vapor pressure using equal area rule9.11 Stable Roots and Saturation Conditions9.12 Temperature Effects on G and f9.13 Summary9.14 Practice Problems9.15 Homework ProblemsUNIT III FLUID PHASE EQUILIBRIA IN 353353354356359359361361362363367CHAPTER 10 INTRODUCTION TO MULTICOMPONENT SYSTEMS 36910.110.210.310.4Introduction to Phase DiagramsVapor-Liquid Equilibrium (VLE) CalculationsBinary VLE Using Raoult’s LawMulticomponent VLE Raoult’s Law CalculationsExample 10.1 Bubble and dew temperatures and isothermal flash of ideal solutionsExample 10.2 Adiabatic flash10.5 Emissions and Safety10.6 Relating VLE to Distillation10.7 Nonideal Systems10.8 Concepts for Generalized Phase Equilibria10.9 Mixture Properties for Ideal Gases10.10 Mixture Properties for Ideal Solutions10.11 The Ideal Solution Approximation and Raoult’s Law10.12 Activity Coefficient and Fugacity Coefficient Approaches10.13 Summary10.14 Practice Problems10.15 Homework ProblemsCHAPTER 11 AN INTRODUCTION TO ACTIVITY MODELS11.1 Modified Raoult’s Law and Excess Gibbs 5407407411412
ContentsExample 11.1 Gibbs excess energy for system 2-propanol water11.2 Calculations Using Activity CoefficientsExample 11.2 VLE predictions from the Margules equationExample 11.3 Gibbs excess characterization by matching the bubble pointExample 11.4 Predicting the Margules parameter with the MAB model11.3 Deriving Modified Raoult’s Law11.4 Excess Properties11.5 Modified Raoult’s Law and Excess Gibbs Energy11.6 Redlich-Kister and the Two-Parameter Margules ModelsExample 11.5 Fitting one measurement with the two-parameter Margules equationExample 11.6 Dew pressure using the two-parameter Margules equation11.7 Activity Coefficients at Special CompositionsExample 11.7 Azeotrope fitting with bubble-temperature calculations11.8 Preliminary Indications of VLLE11.9 Fitting Activity Models to Multiple DataExample 11.8 Fitting parameters using nonlinear least squares11.10 Relations for Partial Molar PropertiesExample 11.9 Heats of mixing with the Margules two-parameter model11.11 Distillation and Relative Volatility of Nonideal SolutionsExample 11.10 Suspecting an azeotrope11.12 Lewis-Randall Rule and Henry’s LawExample 11.11 Solubility of CO2 by Henry’s LawExample 11.12 Henry’s constant for CO2 with the MAB/SCVP model11.13 Osmotic PressureExample 11.13 Osmotic pressure of BSAExample 11.14 Osmotic pressure and electroporation of E. coli11.14 Summary11.15 Practice Problems11.16 Homework ProblemsCHAPTER 12 VAN DER WAALS ACTIVITY MODELS12.1 The van der Waals Perspective for Mixtures12.2 The van Laar ModelExample 12.1 Infinite dilution activity coefficients from the van Laar theory12.3 Scatchard-Hildebrand TheoryExample 12.2 VLE predictions using the Scatchard-Hildebrand theory12.4 The Flory-Huggins ModelExample 12.3 Deriving activity models involving volume fractionsExample 12.4 Scatchard-Hildebrand versus van Laar theory for methanol benzeneExample 12.5 Polymer mixing12.5 MOSCED and SSCED TheoriesExample 12.6 Predicting VLE with the SSCED model12.6 Molecular Perspective and VLE Predictions12.7 Multicomponent Extensions of van der Waals’ ModelsExample 12.7 Multicomponent VLE using the SSCED modelExample 12.8 Entrainer selection for gasohol production12.8 Flory-Huggins and van der Waals Theories12.9 Summary12.10 Practice 2494
xii Contents12.11 Homework Problems495CHAPTER 13 LOCAL COMPOSITION ACTIVITY MODELS499Example 13.1 VLE prediction using UNIFAC activity coefficients13.1 Local Composition TheoryExample 13.2 Local compositions in a two-dimensional lattice13.2 Wilson’s EquationExample 13.3 Application of Wilson’s equation to VLE13.3 NRTL13.4 UNIQUACExample 13.4 Combinatorial contribution to the activity coefficient13.5 UNIFACExample 13.5 Calculation of group mole fractionsExample 13.6 Detailed calculations of activity coefficients via UNIFAC13.6 COSMO-RS MethodsExample 13.7 Calculation of activity coefficients using COSMO-RS/SAC13.7 The Molecular Basis of Solution Models13.8 Summary13.9 Important Equations13.10 Practice Problems13.11 Homework 532533533534CHAPTER 14 LIQUID-LIQUID AND SOLID-LIQUID PHASE EQUILIBRIA14.1 The Onset of Liquid-Liquid InstabilityExample 14.1 Simple vapor-liquid-liquid equilibrium (VLLE) calculationsExample 14.2 LLE predictions using Flory-Huggins theory: Polymer mixing14.2 Stability and Excess Gibbs Energy14.3 Binary LLE by Graphing the Gibbs Energy of MixingExample 14.3 LLE predictions by graphing14.4 LLE Using ActivitiesExample 14.4 The binary LLE algorithm using MAB and SSCED models14.5 VLLE with Immiscible ComponentsExample 14.5 Steam distillation14.6 Binary Phase Diagrams14.7 Plotting Ternary LLE Data14.8 Critical Points in Binary Liquid MixturesExample 14.6 Liquid-liquid critical point of the Margules one-parameter modelExample 14.7 Liquid-liquid critical point of the Flory-Huggins model14.9 Numerical Procedures for Binary, Ternary LLE14.10 Solid-Liquid EquilibriaExample 14.8 Variation of solid solubility with temperatureExample 14.9 Eutectic behavior of chloronitrobenzenesExample 14.10 Eutectic behavior of benzene phenolExample 14.11 Precipitation by adding antisolventExample 14.12 Wax precipitation14.11 Summary14.12 Practice Problems14.13 Homework 553554556556560561562563567569570570
ContentsCHAPTER 15 PHASE EQUILIBRIA IN MIXTURES BY AN EQUATION OF STATE15.1 Mixing Rules for Equations of StateExample 15.1 The virial equation for vapor mixtures15.2 Fugacity and Chemical Potential from an EOSExample 15.2 K-values from the Peng-Robinson equation15.3 Differentiation of Mixing RulesExample 15.3 Fugacity coefficient from the virial equationExample 15.4 Fugacity coefficient from the van der Waals equationExample 15.5 Fugacity coefficient from the Peng-Robinson equation15.4 VLE Calculations by an Equation of StateExample 15.6 Bubble-point pressure from the Peng-Robinson equationExample 15.7 Isothermal flash using the Peng-Robinson equationExample 15.8 Phase diagram for azeotropic methanol benzeneExample 15.9 Phase diagram for nitrogen methaneExample 15.10 Ethane heptane phase envelopes15.5 Strategies for Applying VLE Routines15.6 Summary15.7 Practice Problems15.8 Homework ProblemsCHAPTER 16 ADVANCED PHASE DIAGRAMS16.116.216.316.416.5Phase Behavior Sections of 3D ObjectsClassification of Binary Phase BehaviorResidue CurvesPractice ProblemsHomework 8599601603603604606613613617630636636UNIT IV REACTION EQUILIBRIA639CHAPTER 17 REACTION EQUILIBRIA64117.1 IntroductionExample 17.1 Computing the reaction coordinate17.2 Reaction Equilibrium Constraint17.3 The Equilibrium Constant17.4 The Standard State Gibbs Energy of ReactionExample 17.2 Calculation of standard state Gibbs energy of reaction17.5 Effects of Pressure, Inerts, and Feed RatiosExample 17.3 Butadiene production in the presence of inerts17.6 Determining the Spontaneity of Reactions17.7 Temperature Dependence of KaExample 17.4 Equilibrium constant as a function of temperature17.8 Shortcut Estimation of Temperature EffectsExample 17.5 Application of the shortcut van’t Hoff equation17.9 Visualizing Multiple Equilibrium Constants17.10 Solving Equilibria for Multiple ReactionsExample 17.6 Simultaneous reactions that can be solved by handExample 17.7 Solving multireaction equilibria with 658660
xiv Contents17.11 Driving Reactions by Chemical CouplingExample 17.8 Chemical coupling to induce conversion17.12 Energy Balances for ReactionsExample 17.9 Adiabatic reaction in an ammonia reactor17.13 Liquid Components in ReactionsExample 17.10 Oligomerization of lactic acid17.14 Solid Components in ReactionsExample 17.11 Thermal decomposition of methane17.15 Rate Perspectives in Reaction Equilibria17.16 Entropy Generation via Reactions17.17 Gibbs MinimizationExample 17.12 Butadiene by Gibbs minimizationExample 17.13 Direct minimization of the Gibbs energy with ExcelExample 17.14 Pressure effects for Gibbs energy minimization17.18 Reaction Modeling with Limited Data17.19 Simultaneous Reaction and VLEExample 17.15 The solvent methanol processExample 17.16 NO2 absorption17.20 Summary17.21 Practice Problems17.22 Homework ProblemsCHAPTER 18 ELECTROLYTE SOLUTIONS18.1 Introduction to Electrolyte Solutions18.2 Colligative PropertiesExample 18.1 Freezing point depressionExample 18.2 Example of osmotic pressureExample 18.3 Example of boiling point elevation18.3 Speciation and the Dissociation Constant18.4 Concentration Scales and Standard States18.5 The Definition of pH18.6 Thermodynamic Network for Electrolyte Equilibria18.7 Perspectives on Speciation18.8 Acids and BasesExample 18.4 Dissociation of fluconazole18.9 Sillèn Diagram Solution MethodExample 18.5 Sillèn diagram for HOAc and NaOAcExample 18.6 Phosphate salt and strong acidExample 18.7 Distribution of species in glycine solution18.10 ApplicationsExample 18.8 Dissociation and solubility of fluconazole18.11 Redox ReactionsExample 18.9 Alkaline dry-cell battery18.12 Biological ReactionsExample 18.10 ATP hydrolysisExample 18.11 Biological fuel cell18.13 Nonideal Electrolyte Solutions: Background18.14 Overview of Model Development18.15 The Extended Debye-Hückel Activity 3704709712713717721723726727730731737738739740742
Contentsxv18.16 Gibbs Energies for Electrolytes18.17 Transformed Biological Gibbs Energies and Apparent Equilibrium ConstantsExample 18.12 Gibbs energy of formation for ATP18.18 Coupled Multireaction and Phase EquilibriaExample 18.13 Chlorine water electrolyte solutions18.19 Mean Ionic Activity Coefficients18.20 Extending Activity Calculations to High Concentrations18.21 Summary18.22 Supplement 1: Interconversion of Concentration Scales18.23 Supplement 2: Relation of Apparent Chemical Potential to Species Potentials18.24 Supplement 3: Standard States18.25 Supplement 4: Conversion of Equilibrium Constants18.26 Practice Problems18.27 Homework CHAPTER 19 MOLECULAR ASSOCIATION AND SOLVATION76719.119.219.319.4Introducing the Chemical ContributionEquilibrium CriteriaBalance Equations for Binary SystemsIdeal Chemical Theory for Binary SystemsExample 19.1 Compressibility factors in associating/solvating systemsExample 19.2 Dimerization of carboxylic acidsExample 19.3 Activity coefficients in a solvated system19.5 Chemical-Physical Theory19.6 Wertheim’s Theory for Complex MixturesExample 19.4 The chemical contribution to the equation of state19.7 Mass Balances for Chain AssociationExample 19.5 Molecules of H2O in a 100 ml beaker19.8 The Chemical Contribution to the Fugacity Coefficient and Compressibility Factor19.9 Wertheim’s Theory of PolymerizationExample 19.6 Complex fugacity for the van der Waals modelExample 19.7 More complex fugacity for the van der Waals model19.10 Statistical Associating Fluid Theory (The SAFT Model)Example 19.8 The SAFT model19.11 Fitting the Constants for an Associating Equation of State19.12 Summary19.13 Practice Problems19.14 Homework ProblemsAPPENDIX A SUMMARY OF COMPUTER PROGRAMSA.1A.2A.3A.4A.5A.6Programs for Pure Component PropertiesPrograms for Mixture Phase EquilibriaReaction EquilibriaNotes on Excel SpreadsheetsNotes on 815
xvi ContentsAPPENDIX B MATHEMATICSB.1 Important RelationsB.2 Solutions to Cubic EquationsB.3 The Dirac Delta FunctionExample B.1 The hard-sphere equation of stateExample B.2 The square-well equation of stateAPPENDIX C STRATEGIES FOR SOLVING VLE PROBLEMSC.1 Modified Raoult’s Law MethodsC.2 EOS MethodsC.3 Activity Coefficient (Gamma-Phi) MethodsAPPENDIX D MODELS FOR PROCESS SIMULATORSD.1D.2D.3D.4D.5OverviewEquations of StateSolution ModelsHybrid ModelsRecommended Decision TreeAPPENDIX E THEMODYNAMIC PROPERTIESE.1 Thermochemical DataE.2 Latent HeatsE.3 Antoine ConstantsE.4 Henry’s Constant with Water as SolventE.5 Dielectric Constant for WaterE.6 Dissociation Constants of Polyprotic AcidsE.7 Standard Reduction PotentialsE.8 Biochemical DataE.9 Properties of WaterE.10 Pressure-Enthalpy Diagram for MethaneE.11 Pressure-Enthalpy Diagram for PropaneE.12 Pressure-Enthalpy Diagram for R134a 9849852854865866867869
PREFACE“No happy phrase of ours is ever quite original with us; there is nothing of ourown in it except some slight change born of our temperament, character, environment, teachings and associations.”Mark TwainThis textbook is designed for chemical engineering students from the sophomore level to the firstyear of graduate school. The approach blends molecular perspective with principles of thermodynamics to build intuitive reasoning regarding the behavior of species in chemical engineering processes and formulations. The molecular perspective is represented by descriptions encompassing:the relation of kinetic energy to temperature; the origin and consequences of intermolecular potentials; molecular acidity and basicity; methods used to incorporate molecular properties into molecular simulations; and the impact of molecular properties on macroscopic energy and entropy. Thistext is distinctive in making molecular perspectives accessible at the introductory level and connecting properties with practical implications.This second edition offers enhanced coverage of biological, pharmaceutical, and electrolyteapplications including osmotic pressure, solid solubility, and coupled reactions. Throughout thetext, topics are organized to implement hierarchical instruction with increasing levels of detail.Content requiring deeper levels of theory is clearly delineated in separate sections and chapters.Less complex empirical model approaches have been moved forward to provide introductory practice with concepts and to provide motivation for understanding models more fully. The approachalso provides more instructor flexibility in selecting topics to cover. Learning objectives have beenclearly stated for each chapter along with chapter summaries including “important equations” toenhance student focus. Every chapter includes practice problems with complete solutions availableonline, as well as numerous homework problems. Online supplements include practice tests spanning many years, coursecasts describing difficult concepts or how to use computational tools, ConcepTests to quickly check comprehension, and objective lists that can be customized for greaterdetail. We also recommend the related resources available at the www.learncheme.com.xvii
xviii PrefaceUnique features of the text include the level of pedagogical development of excess functionmodels and electrolytes. For mixture models, the key assumptions and derivation steps are presented, stimulating readers to consider how the molecular phenomena are represented. For electrolytes and biological systems, the text makes connections between pH and speciation and providestools for rapidly estimating concentrations of dissociated species. We emphasize speciation andproblem solving in this introduction, instead of focusing on advanced theories of electrolyte activity. The material is written at an intermediate level to bridge students from the introductions inchemistry to the more complex models of electrolytes provided by process simulators.We have created a number of homework problems with many variants, intending that differentparts can be assigned to different classes or groups, not intending that each student work all parts.NOTES TO STUDENTSThermodynamics is full of terminology and defined properties. Please note that the textbook provides a glossary and a summary of notation just before Unit I. Also consider the index a resource.We consider the examples to be an integral part of the text, and we use them to illustrate important points. Examples are often cross-referenced and are therefore listed in the table of contents. Weenclose important equations in boxes and we use special notation by equation numbers: (*) meansthat the equation assumes temperature-independent heat capacity; (ig) means the equation is limited to ideal gases. We include margin notes to highlight important concepts or supplemental information.Computer programs facilitate the solutions to homework problems, but they should not be usedto replace an understanding of the material. Computers are tools for calculating, not for thinking.To evaluate your understanding, we recommend that you know how to solve the problem by handcalculations. If you do not understand the formulas in the programs it is a good indication that youneed to do more studying before using the program so that the structure of the program makessense. It is also helpful to rework example problems from the text using the software.ACKNOWLEDGMENTSAs the above quote from Mark Twain alludes, we are indebted to many oth
The Prentice Hall International Series in the Physical and Chemical Engineering Sciences had its auspicious beginning in 1956 under the direction . 3.5 Mixture Properties for Ideal Solutions 106 Example 3.3 Condensation of a vapor stream 107 . CHAPTER 5 THERMODYNAMICS OF PROCESSES 199