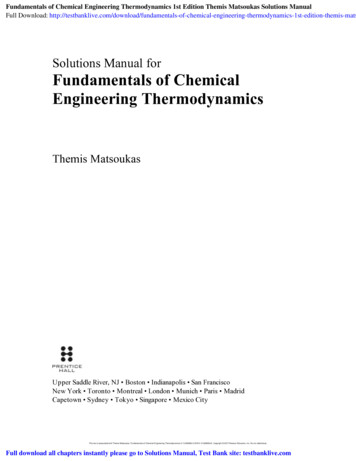
Transcription
Fundamentals of Chemical Engineering Thermodynamics 1st Edition Themis Matsoukas Solutions ManualFull Download: mis-matsSolutions Manual forFundamentals of ChemicalEngineering ThermodynamicsThemis MatsoukasUpper Saddle River, NJ Boston Indianapolis San FranciscoNew York Toronto Montreal London Munich Paris MadridCapetown Sydney Tokyo Singapore Mexico CityThis text is associated with Themis Matsoukas, Fundamentals of Chemical Engineering Thermodynamics 0-13-269306-2 (978-0-13-269306-6). Copyright 2013 Pearson Education, Inc. Do not redistribute.Full download all chapters instantly please go to Solutions Manual, Test Bank site: testbanklive.com
Note to the InstructorAn effort was made to update all solutions requiring steam tables to conform with thetables in Appendix E of the book, which are based on IAPWS95). It is possible, however,that some problems may make use of older tables. Be alert as such discrepancies couldconfuse students even though the final answers are not much different.Please report any mistakes or typos to Themis Matsoukas: matsoukas@psu.edu.The author and publisher have taken care in the preparation of this book, but make noexpressed or implied warranty of any kind and assume no responsibility for errors oromissions No liability is assumed for incidental or consequential damages in connectionwith or arising out of the use of the information or programs contained herein.Visit us on the Web: InformIT.com/phCopyright 2013 Pearson Education, Inc.This work is protected by United States copyright laws and is provided solely for the useof instructors in teaching their courses and assessing student learning. Dissemination orsale of any part of this work (including on the World Wide Web) will destroy theintegrity of the work and is not permitted. The work and materials from it should neverbe made available to students except by instructors using the accompanying text in theirclasses. All recipients of this work are expected to abide by these restrictions and tohonor the intended pedagogical purposes and the needs of other instructors who rely onthese materials.ISBN-10:0-13-269320-8ISBN-13: 978-0-13-269320-2This text is associated with Themis Matsoukas, Fundamentals of Chemical Engineering Thermodynamics 0-13-269306-2 (978-0-13-269306-6). Copyright 2013 Pearson Education, Inc. Do not redistribute.
Contents1Introduction52Phase Diagrams of Pure Fluids133Energy & the First Law594Entropy & the Second Law1035Calculation of Properties1256Balances in Open Systems1437VLE of pure Fluid1938Phase Behavior of Mixtures2239Properties of Mixtures24510 VLE of Mixture27111 Ideal Solution29112 Non-Ideal Solution31113 Miscibility, Solubility and other Phase Equilibria34714 Reactions3713This text is associated with Themis Matsoukas, Fundamentals of Chemical Engineering Thermodynamics 0-13-269306-2 (978-0-13-269306-6). Copyright 2013 Pearson Education, Inc. Do not redistribute.
This text is associated with Themis Matsoukas, Fundamentals of Chemical Engineering Thermodynamics 0-13-269306-2 (978-0-13-269306-6). Copyright 2013 Pearson Education, Inc. Do not redistribute.
1 Introduction5This text is associated with Themis Matsoukas, Fundamentals of Chemical Engineering Thermodynamics 0-13-269306-2 (978-0-13-269306-6). Copyright 2013 Pearson Education, Inc. Do not redistribute.
1. I NTRODUCTIONProblem 1.1 SolutionWe will need the following unit conversions:1 ft D 0:3048 m;1 lb D 0:454 kg;1 lbmol D 454 mol(Note on the conversion mol to lbmol: one mol has a mass equal to the molecular weight in g, while onelbmol has a mass equal equal to the molecular weight in lb.) We also need the molar mass of ammoniawhich isMm D 17 g/mol D 17 10 3 kg/mola) Specific Volume:V D1D 0:02421 ft3 /lb D 0:00151 m3 /kg D 1:51 cm3 /g41:3 lb/ft3b) Molar VolumeVmolar D VMm D 2:567 105m3 /mol D 25:67 cm3 /mol D 0:4116 ft3 /lb-mol6This text is associated with Themis Matsoukas, Fundamentals of Chemical Engineering Thermodynamics 0-13-269306-2 (978-0-13-269306-6). Copyright 2013 Pearson Education, Inc. Do not redistribute.
Problem 1.2 Solution First we write the given equation asTK DAB.ln Pbar / .ln 10/Cwhere TK refers to T in kelvin, Pbar refers to pressure in bar, and the logarithm is natural. Next we useTF D 1:8.TK273:15/ C 32andPbar DPpsi14:5and substitute these values into the above equation. After some manipulation the result isTF DB.1:8/.ln 10/A ln 10 C ln.14:5/ ln PpsiC.1:8/.1:8/.273:15/ C 32Doing the algebra,TF D5062:3713:2153 ln Ppsi302:217Therefore,A0 D 13:2153;B 0 D 5062:37C 0 D 302:2177This text is associated with Themis Matsoukas, Fundamentals of Chemical Engineering Thermodynamics 0-13-269306-2 (978-0-13-269306-6). Copyright 2013 Pearson Education, Inc. Do not redistribute.
1. I NTRODUCTIONProblem 1.3 Solution a) The mean velocity is vN Dwhere kB D 1:38 10238kB T m 1 2J K, T D 273:16 K. The mass of the water molecule is18 10 3 kg molMmDD 2:98904 10NAV6:022 1023 mol 1mD26kg:The mean velocity isvN D 566:699 m s D 2040:12 km h D 1267 mphThis result depends only on temperature and since all three phases are the same temperature, molecules havethe same mean velocity in all three phases.b) The mean kinetic energy isEN kin D 12 mv 2 D 21 mv 2where v 2 is the mean squared velocity,v2 D3kB T:mWith this the mean kinetic energy is3EN kin D kB T D 5:65441 10221JThis is the mean kinetic energy per molecule in all phases. The number of molecules in 1 kg of water isN D1 kg6 022 1023 D 3:34556 102518 10 3 kg molThe total kinetic energy in 1 kg of water at 0.01 ı C (regardless of phase) is EN kin D 5:65441 10 21 J 3:34556 1025 D 189; 171 J D 189 kJComment: This is the translational kinetic energy of the molecule, i.e., the kinetic energy due to themotion of the center of mass. A water molecule possesses additional forms of kinetic energy that arisefrom the rotation of the molecule, the bending of bonds, and the vibration of oxygen and hydrogen atomsabout their equilibrium positions. These are not included in this calculation as the Maxwell-Boltzmanndistribution refers specifically to the translational kinetic energy.c) The above calculation shows that the mean kinetic energy depends only on temperature (it is independentof pressure or of the mass of the molecule). Therefore, oxygen at 0.01 ı C has the same kinetic energy aswater at the same temperature:3EN kin D kB T D 5:65441 10221J8This text is associated with Themis Matsoukas, Fundamentals of Chemical Engineering Thermodynamics 0-13-269306-2 (978-0-13-269306-6). Copyright 2013 Pearson Education, Inc. Do not redistribute.
Problem 1.4 SolutionPotentialF HJouleL4. 10-222. 10-220-2. 10-22-4. 10-22345678910r HÞLa) The potential has a minimum just above 4 Å or so. To determine this value accurately we must set thederivative of the potential equal to zero solve for the value of r. To do this easily, we define a new variablex D r and rewrite the potential as: ˆ D a x 12 x 6By chain rule we now have:dFdF dxDDdrdx dr12x13C 6x7 dxdrSetting this to zero and solving for x we have:12x13C 6x7D0)x D 21 6Since r D x, the value of r that minimizes the potential isr D 21 6 D .4:24964/.3:786 Å/ D 4:25 Åb) If we imagine N molecules to be situated at the center of cubes whose sides are equal to r ,LLthe volume occupied isV D N.r /3These N molecules correspond to N NAv mol and their total mass isM DNMmNAVwhere Mm is the molar mass of methane (Mm D 16 10 3 kg/mol). For the density, therefore, we obtainthe following final formula:Mm DNAV .r /3By numerical substitution we finally obtain the density: D16 10 3 kg/mol.6:024 1023 mol 1 /.4:25 Å/.1010m/Å/D 346 kg/m3 D 0:346 g/cm39This text is associated with Themis Matsoukas, Fundamentals of Chemical Engineering Thermodynamics 0-13-269306-2 (978-0-13-269306-6). Copyright 2013 Pearson Education, Inc. Do not redistribute.
1. I NTRODUCTIONc) Specific volumes of saturated liquid methane are listed in Perry’s Handbook from which we can computethe densities. We notice (as we would have expected) that liquid volumes near the critical point (Tc D190:55 K) vary with pressure, from 162.3 kg/m3 at the critical point to 454 kg/m3 around 90 K. Our valuecorresponds to Perry’s tabulation at about 160 K. Our calculation is approximate and does not incorporatethe effect of pressure and temperature. Notice that if we pick a distance somewhat different from r theresult will change quite a bit because if the third power to which this distance is raised. But the importantconclusion is that the calculation placed the density right in the correct range between the lowest and highestvalues listed in the tables. This says that our molecular picture of the liquid, however idealized, is fairly closeto reality.10This text is associated with Themis Matsoukas, Fundamentals of Chemical Engineering Thermodynamics 0-13-269306-2 (978-0-13-269306-6). Copyright 2013 Pearson Education, Inc. Do not redistribute.
Problem 1.5 SolutionAssuming molecules to be sitting at the center of a cubic lattice with spacing L, the volume occupied by Nmolecules is NL3 and the density isMm DNAV L3where Mm is the molar mass. Solving for the intermolecular distance, MmLDNAV a) For liquid water with D 1000 kg m3 , LD18 10 3 kg mol.6:022 1023 mol 1 /.1000 kg m3 / 1 3D 3:10 1010m D 3:01 Åb) For steam with D 0:4 kg m3 LD18 10 3 kg mol.6:022 1023 mol 1 /.0:4 kg m3 / 1 3D 4:2 109m D 42 ÅThe intermolecular distance is about 10 times larger in the vapor.11This text is associated with Themis Matsoukas, Fundamentals of Chemical Engineering Thermodynamics 0-13-269306-2 (978-0-13-269306-6). Copyright 2013 Pearson Education, Inc. Do not redistribute.
1. I NTRODUCTIONProblem 1.6 SolutionP0P 02RFThe force needed to separate the two haves is equal to the force that is exerted by pressure on one hemisphere:F D P Awhere P is the pressure difference between the atmospheric pressure and the contents of the sphere, andA is the cross-sectional area of the sphere ( R2 ). Assuming the sphere to be fully evacuated, the pressuredifference is equal to the atmospheric pressure ( 1 bar D 105 Pa). The force now isF D P0 . R2 / D .105 Pa/. 0:52 m2 / D 19; 635 NThis force corresponds to a massM DF39269:9 NDD 2; 000 kg:g9:81 N kgAssuming an average weight of 80 kg/person, it would take the weight of 25 people (!) to separate thespheres.12This text is associated with Themis Matsoukas, Fundamentals of Chemical Engineering Thermodynamics 0-13-269306-2 (978-0-13-269306-6). Copyright 2013 Pearson Education, Inc. Do not redistribute.
2 Phase Diagrams of Pure Fluids13This text is associated with Themis Matsoukas, Fundamentals of Chemical Engineering Thermodynamics 0-13-269306-2 (978-0-13-269306-6). Copyright 2013 Pearson Education, Inc. Do not redistribute.
2. P HASE D IAGRAMS OF P URE F LUIDSProblem 2.1 Solution a) 25 ı C, 1 bar: liquid, because the temperature is below the saturation temperatureat 1 bar (99.63ı C).10 bar, 80ı C: liquid, because the temperature is below the saturation temperature at 10 bar (179.88 ı C).120 ı C, 50 bar: At 120 ı C the vapor pressure is 198.54 kPa 1.9884 bar. Since the actual pressure is higher,the state is liquid. (Or, at 50 bar the saturation temperature is 263.91 ı C. Since the actual temperature islower the state is liquid.)b) Liquid, because the pressure (1 atm 760 mm Hg) is higher than the vapor pressure of bromobenzene(10 mg H) at the same temperature.c) Liquid, because the temperature is lower than the boiling point at the same pressure.Note: All of these statements will make better sense if you plot your information on the P V graph.14This text is associated with Themis Matsoukas, Fundamentals of Chemical Engineering Thermodynamics 0-13-269306-2 (978-0-13-269306-6). Copyright 2013 Pearson Education, Inc. Do not redistribute.
Problem 2.2 Solution a) From steam tables at 40 bar we collect the following data:600 ı C650 ı C340 bar 0:0989 m kg 0:1049 m3 kgBy interpolation at V D 100 g cm3 D 0:1 m3 kg we findT D 600 C6500:1049600.0:10:09890:0989/ D 609:2 ı CThe system is at 40 bar, 609.2 ı C: the phase is vapor.b) At 6 bar we find that the desired volume lies between that of the saturated liquid (VL D 0:0011 m3 kg)and saturated vapor (VV D 0:3156): the system is a vapor/liquid mixture. The vapor and liquid fractions areobtained by lever rule:0:3156 0:1D 0:314 D 31:4%0:3156 0:0011xV D 1 0:314 D 0:686 D 68:6%xL D15This text is associated with Themis Matsoukas, Fundamentals of Chemical Engineering Thermodynamics 0-13-269306-2 (978-0-13-269306-6). Copyright 2013 Pearson Education, Inc. Do not redistribute.
2. P HASE D IAGRAMS OF P URE F LUIDSProblem 2.3 Solution a) At 1 bar, 210 ı C, the specific volume of steam is found by interpolation to beV D 2219:2 cm3 /g D 2:2192 m3 /kgSince the total volume of the vessel is 3.5 m3 , the mass isM DVt3:5 m3DD 1:577 kgV2:2192 m3 /kgb) Since the system is a mixture of liquid and vapor, it is saturated. From the saturated tables at 20 bar wefind by interpolation the following:T D 212:37 ı C;VL D 1:18 cm3 /g;VV D 99:54 cm3 /gThe specific volume of the mixture isV D lVL C vVV D .0:85/.1:18/ C .0:15/.99:54/ D 15:93 cm3 /gThe total volume required for storage is V t D M V , where V is the specific volume calculated above:V t D M V D .525 kg/.1000 g/kg/.15:93 cm3 /g/ D 8363250 cm3 D 8:36 m316This text is associated with Themis Matsoukas, Fundamentals of Chemical Engineering Thermodynamics 0-13-269306-2 (978-0-13-269306-6). Copyright 2013 Pearson Education, Inc. Do not redistribute.
Problem 2.4 Solution Solution Initial state:P D 45 bar; T D 257:41 ı C; V L D 1:269 cm3 /g; V V D 44:037 cm3 /gThe specific volume is obtained from the lever rule with X L D 0:25:V D x L V L C .1x L /V V D .0:25/.1:269/ C .10:25/.44:037/ D 33:345 cm3 /ga) We know P D 80 bar, V D 33:345 cm3 /g. From the steam tables we find:T D 375 ı C;V D 32:222 cm3 /gT D 400 ı C; V D 34:31 cm3 /gBy interpolation at V D 33:345 cm3 /g we obtain T D 388:5 ı C.b) From saturated tables we find:T D 272 ı C;P D 56:802 bar;V D 34:42 cm3 /gT D 274 ı C;P D 58:587 bar;V D 33:29 cm3 /gBy interpolation at V D 33:345 cm3 /g we find P D 57:76 bar.c) The volume fraction of the liquid, L , and the mass fraction of the liquid, x L , are related as follows:xLV Lvol. occupied by liq.D L Lvol. occupied by liq. vol. occupied by vapx V C xV V Vfrom which,xL DV V LV L C .V V V L / LThe saturated volumes are those at the initial state and with L D 0:25 we findxL D.44:037/.0:25/D 0:920429.1:269/ C .44:037 1:269/.0:25/Therefore, the new specific volume in the tank isV 0 D .0:920429/.1:269/ C .10:920429/.44:037/ D 4:6721 cm3 /gIf we have mass M initially and mass M 0 at the end, we can write for the tank volume:V t D NV D V tM0orMM0)VM0D 0MVV33:3451DD 6:140MV4:6721Therefore, we must add 6.14 times the original mass (that’s 614%).D17This text is associated with Themis Matsoukas, Fundamentals of Chemical Engineering Thermodynamics 0-13-269306-2 (978-0-13-269306-6). Copyright 2013 Pearson Education, Inc. Do not redistribute.
2. P HASE D IAGRAMS OF P URE F LUIDSProblem 2.5 SolutionP .bar/0:5153V .m kg/ 1:1240:031 .kg m3 / 0:88968 32:2581If we interpolate for V we findV D 0:257138 m3 kg:If we interpolate for we find D 25:7681 kg m3)V D 1 D 0:0388077 m3 kg:These results are quite different. Which one should we pick?For the molar volume we haveV DZRT:PUsing D 1 V , the molar density isP(2.1)ZRTWe conclude that while V is inversely proportional to P , 1 V is proportional, provided that Z does not varymuch in the given range. Therefore, we would accept the interpolation in . D18This text is associated with Themis Matsoukas, Fundamentals of Chemical Engineering Thermodynamics 0-13-269306-2 (978-0-13-269306-6). Copyright 2013 Pearson Education, Inc. Do not redistribute.
Problem 2.6 Solutiona) 198.3 Cb) We first obtain the specific volumes of the saturated liquid and vapor at 15 bar:V L D 0:0015 m3 kg;V V D 0:1317 m3 kgNext we calculate the total mass of each phase:mL D .0:5 m3 /.0:0015 m3 kg/ D 434:8 kgmV D .11:5 m3 /.0:1317 m3 kg/ D 87:3 kgThe total mass ism D 434:8 C 87:3 D 522:1 kgc) The quality of the steam is:87:3D 0:167522:1d) The specific volume after 87% of the mass is removed isxV DV0 D0:13 522:1D 0:1768 m3 kg12 m3The temperature is obtained by interpolation at 15 bar between 250 ı C and 300 ı C. We findT 0 D 320 ı C19This text is associated with Themis Matsoukas, Fundamentals of Chemical Engineering Thermodynamics 0-13-269306-2 (978-0-13-269306-6). Copyright 2013 Pearson Education, Inc. Do not redistribute.
2. P HASE D IAGRAMS OF P URE F LUIDSProblem 2.7 Solution a) Since the cooker contains both vapor and liquid, the state is saturated steam.Therefore, T D T sat D 120:23 ı C.b) From steam tables we obtain the specific volumes of the saturated phasees:V L D 1:061 cm3 /g;V V D 885:44 cm3 /gThe total volume of the liquid in the cooker is V L;t ot D .0:25/.8/ liter 2000 cm3 . Therefore, the mass ofthe liquid ismL DV L;tot2000DD 1885:0 gL1:061VThe volume of the vapor in the cooker is V L;tot D .0:75/.8/ liter 6000 cm3 and its mass is given bymV D6000V L;totDD 6:78 g885:44VVThe total mass ism D 1885:0 C 6:78 D 1891:78 g D 1:89 kgc) The mass fractions of the liquid (xL ), and of the vapor (xV ) arexL D1885:0D 0:9964 D 99:64%;1885:0 C 6:78xV D 1xL D 0:004 D 0:4%Even though the vapor occupies 75% of the volume, it only represents 0.4% of the total mass.d) The quick solution is to take a look at the P V graph. The initial state is at A and the final state, B, isreached by constant-volume cooling. This state is obviously in the two-phase region because the originatingstate was also inside that region as well. We conclude that P D P sat .25 ı C/ D 3:166 kPa. (If, however,state A were in the superheated region, we would be able to tell if B is superheated or vapor/liquid and wewould have to do the solution in more detail as shown below.)Detailed solution: The total volume of the system as well as the specific volume remain constant. Thespecific volume isV DVcooker8000 cm3DD 4:229 cm3 /gMtot1891:78 gFrom the saturated steam tables at 25 ı C we find, V L D 1:003 cm3 /g, V V D 43400 cm3 /g. The specificvolume of the system is between these two values, therefore we still have a saturated system. We concludethat P D P sat .25 ı C/ D 3:166 kPa D 0:03166 bar.20This text is associated with Themis Matsoukas, Fundamentals of Chemical Engineering Thermodynamics 0-13-269306-2 (978-0-13-269306-6). Copyright 2013 Pearson Education, Inc. Do not redistribute.
Pinitial state120.21 C2 bar25 C0.003175barfinal stateVconst. VprocessThis solution is more general and will work regardless of where the initial state is.e) When the system has cooled, the outside pressure is 1 bar and the inside pressure is 0.03166 bar. Therefore, the lid remains closed under the action of this pressure difference. The force isF D R2 .PoutPin / D . /.0:2 m2 /.10:03166/ bar 105 N/bar D 12166 NTo put this force into perspective we calculate the mass whose weight is 12166 N:M DF12166 NDD 1240 kg D 2732 lbg9:81 m/s2If you can lift 2700–2800 lb then you could remove that lid! (Note: Whether you take the outside pressureto be 1 bar or 1 atm or something similar, the conclusion remains that the required force is indeed very large.21This text is associated with Themis Matsoukas, Fundamentals of Chemical Engineering Thermodynamics 0-13-269306-2 (978-0-13-269306-6). Copyright 2013 Pearson Education, Inc. Do not redistribute.
2. P HASE D IAGRAMS OF P URE F LUIDSProblem 2.8 SolutionIt is very helpful to draw the P V graph, shown below:part (a)part (b)D'D1 barA1 barBC80 CC'EHFGpart (c)part (d)a) The initial state (A) is compressed liquid (80 ı C, 1.013 bar). The process is conducted under constantvolume. Assuming the isotherm to be vertical, the final state, B, is at the same temperature and on thesaturation line. Therefore: T D 80 ı C, P D 47:36 kPa.Notice that the temperature has not changed. This is a consequence of the fact that we have approximatedthe isotherm with a vertical line. In reality, the isotherm is not vertical and state B should be at a temperaturesomewhat below 80 ı C. However, the steepness of the isotherm means that this temperature is very close to80 ı C. If we had the value of and ˇ we could calculate this temperature and would verify that it is indeedextremely close to 80 ı C.b) The initial state is a V/L mixure (state C). The process is under constant volume, therefore, the final stateis located at the intersection of the vertical line through C and the saturation line (state D). From steamtables:1 bar; saturated:VL D 1:043; V V D 1693:7The mass fractions of the liquid and the vapor are:lD0:5 1:043D 0:999380:5 1:043 C 0:5 1693:7vD1l D 0:0006222This text is associated with Themis Matsoukas, Fundamentals of Chemical Engineering Thermodynamics 0-13-269306-2 (978-0-13-269306-6). Copyright 2013 Pearson Education, Inc. Do not redistribute.
The specific volume at the initial state is (in cm3 /g)V D .0:99938/.1:043/ C .0:00062/.1693:7/ D 2:085At the final state: V D 2:085, and saturated. From steam tables we find, T 367 ı C, P D 203:13 bar(saturated liquid).Note 1: Even though the mass fraction of the vapor is very nearly 0, it would not be correct to set it equal to0. While v is small, when multiplied by a large V V it makes a significant contribution to the specificvolume of the mixture. If we had set v , we would have concluded that the initial state is practicallysaturated liquid which means that the final pressure is almost 1 bar. Clearly, this approximation missesthe right pressure by more than 200 bar!!!Note 2: In this case the specific volume of the vapor-liquid mixture was very close to the liquid side and forthis reason the final state was liquid. In other words, under heating the vapor condenses and becomesliquid. If, however, the initial volume was much closer the vapor side (state C’), then heating wouldproduce vapor. In this case, heating would cause the liquid to evaporate. That is, after heating thecontents of the vessel the final state might either saturated vapor or saturated liquid. Can you establisha criterion for the initial specific volume to determine whether the final state is vapor or liquid?c) The final state saturated vapor (state F). The process is cooling under constant volume, therefore, theinitial state must be somewhere on the vertical line through F and above point F (since cooling implies thatthe initial state is at higher T ). We conclude the initial state is superheated vapor.d) By similar arguments as above, we determine that the initial state is vapor/liquid mixture. Notice thathere we are heating a vapor/liquid mixture and as a result the vapor condenses to produce saturated liquid!23This text is associated with Themis Matsoukas, Fundamentals of Chemical Engineering Thermodynamics 0-13-269306-2 (978-0-13-269306-6). Copyright 2013 Pearson Education, Inc. Do not redistribute.
2. P HASE D IAGRAMS OF P URE F LUIDSProblem 2.9 Solution The specific volume of water under these conditions in the tank isV D12 m3D 1:935 m3 kg:6:2 kgAccording to the steam tables, the volume of saturated vapor at 1.4 bar is between 1.694 m3 kg (at 1 bar)and 1.1594 m3 kg (at 1.5 bar). The calculated value is higher, therefore, the state is to the right of thesaturated vapor and it must be superheated.b) We need an entry in the steam tables such that pressure is 1.4 bar and the specific volume is 1:935 m3 kg.To locate this state, we interpolated in the steam tables between 1.0 bar and 1.5 bar at various temperaturesand construct the table below:T150200250300350400450V .at 1:0 bar/ 1:9367 2:1725 2:4062 2:6389 2:8710 3:1027 3:3342V .at 1:4 bar/ 1:4158 1:5901 1:7623 1:9335 2:1040 2:2742 2:4442V .at 1:5 bar/ 1:2856 1:4445 1:6013 1:7571 1:9123 2:0671 2:2217We can now see that the desired value is between 300 ı C and 350 ı C. By interpolation between these twotemperatures we findT D 300 ı C C.350.1:5300/ ı C.1:41:0/ bar1:0/ bar D 300:6 Cc) If we add more steam while keeping temperature constant to 300.6 C, pressure will increase and thespecific volume will decrease. The vapor will become saturated when the specific volume in the tank is thatof saturated vapor at 300.6 C. We obtain this value by interpolation in the saturated steam tables between300 ı C and 302 ı C:T ıCV m3 kg3000:02166300:593 0:02114983020:01994The specific volume when the tank is saturated is V D 0:0211498 m3 kg. The total mass is12 m36:2 kg D 567:4 kg0:0211498 m3 kgThe amount that must be added is.567:46:2/ D 561:2 kg24This text is associated with Themis Matsoukas, Fundamentals of Chemical Engineering Thermodynamics 0-13-269306-2 (978-0-13-269306-6). Copyright 2013 Pearson Education, Inc. Do not redistribute.
Problem 2.10 Solution SolutionThe graphs below show the P V in various combinations of linear and logarithmic coordinates and the ZPgraph.250250Pressure (bar)Pressure (bar)400 C200150100300 C400 C200150100300 C5050200 C100 C0200 C100 C0202040608062461024100610003Specific volume (cm /g)Specific volume (cm /g)1.0Compressibility Factor1000400 CPressure (bar)411003100300 C200 C101100 C0.10.010.8400 C100 C0.6200 C0.4300 C0.20.00101101021031045310Specific volume (cm /g)050100150200250Pressure (bar)Comments: The volumes span a very wide range and in order to see the shape of the saturation line, we must plotonly a smaller range. In the above graph, the volume axis ranges from 0 to 100 cm3 /mol. By doing the V axis in log coordinates we can now look at a very wide range of values withoutsqueezing the graph into nothingness. Notice that in the log plot the volume goes from 1 to 100,000cm3 /g. The steam tables do not contain data for the compressed liquid region and so our isotherms stop at thesaturated liquid. We could extrapolate them into the liquid by drawing them as vertical lines. The ZP graph has the familiar look. Notice that the isotherms are better separated on this graph.25This text is associated with Themis Matsoukas, Fundamentals of Chemical Engineering Thermodynamics 0-13-269306-2 (978-0-13-269306-6). Copyright 2013 Pearson Education, Inc. Do not redistribute.
2. P HASE D IAGRAMS OF P URE F LUIDSProblem 2.11 Solutiona) We find that for ethane,Tc D 305:3K; Pc D 48:72barThe reduced conditions areTr D298:15 KD 0:976;305:3 KPr D10 barD 0:2148:72 barThe desired isotherm is between Tr D 0:95 and Tr D 1:0. By graphical interpolation we find Z 0:92. theisotherm is fairly linear between Pr D 0 and the value of Pr corresponding to the given pressure, therefore,the truncated virial equation is valid.b) The reduced conditions areTr D244:15 KD 0:80;305:3 KPr D10 barD 0:2148:72 barZ cannot find at Tr D 0:80 under the given pressure. therefore, the truncated virial equation is not valid.c) Since the temperature isequation is not valid.35ı C, lower than that boiling point, the ethane is liquid. The truncated virial26This text is associated with Themis Matsoukas, Fundamentals of Chemical Engineering Thermodynamics 0-13-269306-2 (978-0-13-269306-6). Copyright 2013 Pearson Education, Inc. Do not redistribute.
Problem 2.12 Solution a) Vapor.b) The molar mass can be obtained from the relationship between molar volume and density: DMmV)Mm D VWe know density at two pressures, so we need the molar volume in one of them. We choose the lowestpressure because at 0.01 we are justified (below) to assume ideal-gas state:Justification: The critical pressure is not known but it must be higher than the saturation pressure at 25 C,which is 64.3 bar. That is, the reduced pressure is at most0:01 barD 0:000264:3 barFrom generalized graphs is it clear that at such low reduced pressures the state is essentially ideal.The molar volume isV D.8:314 J mol K/.298:15 K/RTDD 2:47882 m3 molP0:01 105 PaThe molar mass is Mm D 0:177 kg m3 .2:47882 m3 mol/ D 43:8 103kg molc) The second virial coefficient can be calculated from the truncated virial equationPVBPD1CRTRTWe justify the use of this equation at 25 ı C, 20 bar as follows:Justification: The reduced temperature is Tr D 0:97. The reduced pressure is not known but it must be lessthan20 barD 0:3164:3 bar 1,since the critical pressure must be higher than 64.3 bar. From generalized graphs we see that for Tr the isotherm in the pressure range Pr D 0 up to about 0.31 is quite linear. This of course is a judgement callbut is as good as we can do with the information we have.Solving the truncated virial for B:BDVRTPwhere V D Mm . Using the data at 20 bar with V D 0:00110239 m3 mol we obtain:BD1:370 104m3 mold) We will answer this question using the truncated virial equationJustification: If the truncated virial is valid at 25 ı C, 20 bar, as assumed above, it is valid for all pressuresless than 20 bar.27This text is associated with Themis Matsoukas, Fundamentals of Chemical Engineering Thermodynamics 0-13-269306-2 (978-0-13-269306-6). Copyright 2013 Pearson Education, Inc. Do not redistribute.
2. P HASE D IAGRAMS OF P URE F LUIDSSolving the truncated virial for V and using P D 12 bar:V DRTPB D 0:00192866 m3 molThe number of moles to be stored isnD20 kgD 456 mol43:9 10 3 kg moland the volume of the tank isV tank D .456 mol/.0:00192866 m3 mol/ D 0:88 m328This text is associated with Themis Matsoukas, Fundamentals of Chemical Engineering Thermodynamics 0-13-269306-2 (978-0-13-269306-6). Copyright 2013 Pearson Education, Inc. Do not redistribute.
Problem 2.13 Solution a) We collect the data for this problem:Tc D 190:56 KPc D 45:99 bar! D 0:011We calculate the second virial using the Pitzer equation:Tr D 1:5646Pr D 0:434877B0 D0:12319B1 D 0:112756BD0:0000420106 m3 molThe compressibility factor isZDBP
Solutions Manual for Fundamentals of Chemical Engineering Thermodynamics Themis Matsoukas Upper Saddle River, NJ Boston Indianapolis San Francisco New York Toronto