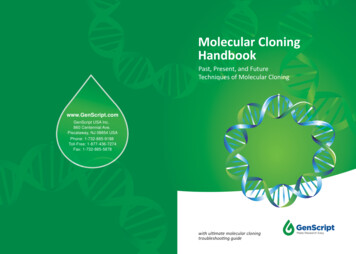
Transcription
Molecular CloningHandbookPast, Present, and FutureTechniques of Molecular Cloningwww.GenScript.comGenScript USA Inc.860 Centennial Ave.Piscataway, NJ 08854 USAPhone: 1-732-885-9188Toll-Free: 1-877-436-7274Fax: 1-732-885-5878with ultimate molecular cloningtroubleshooting guide
The New GenEZTM ORF ClonesTransfection/Expression Ready ORF ClonesFrom 99/cloneTable of ContentsTraditional Molecular Cloning OverviewLooking for an ORF or mutant ORF clone?GenEZTM Clones make ORFs more accessible and more customizable than ever.Search for your ORF using our ORF clone database, select your vector, and place your order online. GenEZTMORF Clones are delivered in our standard mammalian expression/transfection-ready vector, or can besubcloned into the vector of your choice and start at just 99/clone. GenEZTM clones are shipped in as few as5 business days.The History of Molecular CloningMolecular Cloning StrategiesTraditional Molecular Cloning BottlenecksMolecular Cloning Troubleshooting GuideOther Limitations of Traditional Molecular CloningGene Synthesis – The Solution to the Limitations of Traditional Cloning13571113How Gene Synthesis Revolutionized Molecular CloningWhat is Gene Synthesis?What Can Be Synthesized?How is Gene Synthesis Performed?Largest ORF databasein the world 2 million ORFs from 186 species 40,000 ORFs in-stock*Available for on-demand synthesisMutant ORFsMutant sequences for 149Just 3 days additional production time141617Cost-efficientStarting at 99/cloneFully sequence verifiedCustomized vectorsOnly 50 for subcloningChoose from 100 expression vectorsAdd up to 30 bp of 5' and 3' flanking sequencefor freeQuotations and Ordering:https://www.genscript.com/account/gene services orf.htmlgene@genscript.comGenEZTM Custom Molecular Clones and ORF ClonesPowered by Gene SynthesisWhat are Custom Clones?GenEZTM Custom Molecular ClonesWhat are ORF Clones?GenEZTM ORF ClonesGenEZTM ORF Custom Cloning and Mutant ClonesGenEZTM ORF ClonesetsReferences23232425262930
Traditional Molecular Cloning OverviewThe History of Molecular Cloningcutting and gluing of DNA molecules together. Later innovations such astransformation, PCR, Sanger sequencing, and more recently, gene synthesis wouldmake molecular cloning one of the most prolific tools of the molecular biologylaboratory.The key principles of molecular cloning were discovered a little over 50 years ago.Since then, molecular cloning has become one of the most powerful tools of themolecular biology laboratory enabling the expression of the smallest genes, as well asthe engineering of whole genomes.Figure 1. Timeline of molecular cloning history19771952-1953Sanger DNA sequencing & thefirst cloning vector, pBR322,developedGenetic basis for phage restriction describedThe advent of molecular cloning was spawned from a number of observations thatcentered around DNA recombination, namely the exchange of DNA bewteenbacterial and bacteriophage genomes [1, 2]. Key to these pioneering observationswas the discovery that bacteriophage λ formed a circle when it entered the hostbacterial cell. In particular, phage DNA was observed to have single stranded DNAflanking each end. The ends were complementary to each other and were calledcohesive sites or “cos sites”. The cos sites could reanneal to make the phage DNAcircular. Then, excision and insertion events led to the incorporation of bacteriophageDNA into the host genome [3]. Campbell’s model of λ prophage excision and insertionled to the classic principle of DNA recombination. In particular, today’s “sticky ends”,formed by DNA digestion using restriction enzymes mimic the functionality of cossites allowing for the insertion of a target sequence into its destination vector.Next came the discovery of another key tool in molecular cloning, the restrictionenzyme. It was discovered that methylation of phage DNA by host methyltransferasesprevented phage DNA from being destroyed by host enzymes called restrictionnucleases. Foreign DNA molecules not having the methylation patterns in accordancewith their host (or that were unmethlyated) were recognized as foreign anddestroyed by host restriction nucleases [4, 5]. The first restriction nucleases werecharacterized by Meselson and Yuan [6]. Then, Kelly and Smith showed thatrestriction enzymes recognize and cleave specific nucleotide sequences [7]. Today,restriction enzymes are used as biological scissors to cut out the precise DNA desiredto create recombinant sequences. The Escherichia coli strains of today used fortransformation of cloned recombinant DNA lack these enzymes.1962Model of DNArecombination inphages published1967DNA ligasesisolated &characterized1972Firsttransformation ofE. coli19761961First restriction nucleases isolated1970Synthesis offirst syntheticgene1983PCR conceivedof anddemonstratedSequence specificity of restriction nucleasesdemonstratedFinally, came the discovery of enzymes called ligases, which could join two DNAmolecules together [8-12]. The discovery of ligases solidified the groundwork for thebasic principles of molecular cloning – the creation of recombinant DNA via the1www.genscript.comwww.genscript.com2
Molecular Cloning StrategiesSince the seminal discoveries of the basic principles underlying molecular cloning, anumber of cloning strategies have been developed to improve on the ease and speedat which DNA fragments can be recombined.transformants will contain the gene in the sense direction and half will contain thegene in the antisense direction. However, cells transformed with toxic genes may alldisplay the genes in the antisense direction, since cells containing the sense directedgenes will not survive. In addition, survivor cells containing toxic genes oriented inthe sense direction may be mutated to encode a less toxic protein.Traditional cloningFigure 3: Schematic of TA cloningTraditional cloning, also called PCR cloning, requires the use of the polymerase chainreaction (PCR) to amplify the template sequence of interest (usually the gene ofinterest) and add restriction sites to the ends of the sequence. Restriction enzymesare used to cut both the template of interest and the target vector, and DNA ligase isused to join the sticky ends of the template and vector together. Traditional cloningallows for flexible DNA sequence manipulation, which facilitates the building of nearlyany desired construct. However, the checkpoints and optimization proceduresrequired for traditional cloning can be cumbersome, and the reagents required can beexpensive.MCS234567PPGene of interestis amplified byPCR. Plasmldcontains multiplecloning ion sites.Ligation ofdigestionproductsTransformationPlasmld n of PCRproduct and vector byrestriction enzymes.Dephosphorylation ofvector may be required.TA cloningTA cloning is one of the simplest forms of cloning. In this method, vectors containing5' thymine overhangs are used to accept PCR products in which additional 3'adenosine overhangs have been added on by the nature of TAQ polymeraseamplification. TA cloning has the advantage of ease and speed, since no restrictiondigestion step is required. In addition, TA cloning kits contain reaction buffers thatcontain the pre-mixed vector, ligase, and buffer, cutting ligation reaction time to asfew as 5 minutes. The disadvantage of TA cloning technology is that the cloning is notdirectional, meaning the gene of interest may be inserted into the target vector ineither the sense or antisense orientation. Normally, half of the subsequent3www.genscript.comAAT T23Gene of interest is Ligation ofamplified by PCRgene andand containsvectoradenosineoverhangs. Plasmidcontains thymineoverhangs.4Transformation5Plasmid isolation6Constructverification bysequencingSeamless cloningFigure 2: Schematic of PCR cloning11Seamless cloning technologies eliminate the requirement for restriction enzymes.This can be advantageous when an insert contains a number of restriction siteswithin its sequence, making it difficult to identify restriction enzymes that will not cutthe gene of interest during the cloning procedure. Seamless cloning takes advantageof homologous recombination and there are numerous variations on the technique.In general, the procedure consists of adding flanking sequences approximately 15 bpin length to both the insert and vector via PCR. Exonucleases are used to chew backthe insert and vector sequences and the DNA is joined using recombinase enzymesor DNA ligase. Seamless cloning has been simplified by the development of kits thatalready contain the target vector and a proprietary mix of enzymes required for therecombination reaction. For instance, GenScript’s CloneEZTM Kit can clone inserts upto 10 kb in 30 minutes, and can also be used for high-throughput cloning projects.Figure 4: Schematic of seamless cloning1PCRamplification ofgene of interest.Addition of 15bases flankingsequencehomologous tovector.2Exonucleaseschew back insertand vectorsequences.Joining of vectorand insert byrecombinase orligase.34Transformation5Plasmld script.com4
Traditional Molecular Cloning BottlenecksTechnical bottlenecks of traditional molecular cloning can cause the process to betime consuming and expensive. Smoothly run cloning experiments can take about2-3 weeks to complete. However, many cloning experiments require troubleshootingin at least one phase of the process, increasing both cost and time consumption.Below is a list of common bottlenecks that plague the traditional cloning process.Unstable, low yield RNA extraction – Molecular cloning often begins with total RNAextraction from the host containing the gene of interest. For the cloning of proteincoding sequences, mRNA extraction is required. mRNA copy numbers for somegenes can be low, making it difficult to amplify the sequence via RT-PCR. In addition,RNA extraction from some host tissues may prove difficult due to the harshconditions under which some cell types must be lysed. Finally RNA is inherently lessstable than DNA, and great precautions must be taken to prevent the action ofRNases and the degradation of RNA by repetitive freezing and thawing.Difficult PCR program optimization – Following the synthesis of cDNA from mRNAmolecule templates, a PCR program must be designed to amplify the gene ofinterest, as well as add additional elements such as restriction sites ordetection/purification tags. Intrinsic properties of gene sequences such as high GCcontent, long stretches of the same polynucleotide, and sequences encoding hairpinloop structures can all hinder PCR efficiency.DNA losses from purification steps – DNA must be purified at numerous steps in thetraditional cloning process. PCR products must be purified from reactioncomponents; digested genes and vectors must be purified from restriction enzymes,buffers, and digestion products; and plasmid DNA must be extracted and purifiedfrom cellular material. At each step, DNA recovery % can vary from as high as 95% toas low as 60% depending on the purification method and quality of the DNA. Lowrecovery percentages or contamination during the purification procedure can resultin DNA losses so great that the next step of cloning cannot be carried out, requiringsteps such as PCR, digestion, or transformation to be repeated.Low ligation efficiencies – Following the digestion of the gene insert and the targetvector, a ligation reaction is performed to join the two molecules. The efficiency ofligation reactions are dependent on a number of variables including vector to insertratio and salt concentration. If the ratio is too low, or plasmid digestion isincomplete, excess vector may re-anneal without the insert. In some cases the vector5www.genscript.commay require an additional de-phosphorylation reaction step or the insert may requirean extra phosphorylation step, to prevent vector re-annealing and improveinsert-vector ligation efficiency, respectively. In addition, solvent contaminants fromDNA purification procedures such as agarose gel extraction can lower ligationefficiency.Mutations – Mutations can be introduced into the molecular cloning process duringPCR amplification or plasmid propagation. Typical Taq DNA polymerases do notcontain proofreading subunits. Though high-fidelity Taq DNA polymerases thatcontain proofreading subunits, such as Pfu, have been developed, reaction conditionssuch as sustained high temperatures can still cause DNA damage that results inmutations.During plasmid propagation, toxic genes may be expressed at low levels due to RNApolymerase read through of antibiotic resistance genes, or leaky promoters. Proteinsor enzymes encoded by toxic genes may cause the transformed cells to die, resultingin only the survival of transformants containing mutated genes encoding less potentversions of the target protein or enzyme.Figure 5: Traditional molecular cloning steps and timelineAt best, traditional cloning may take only 2 weeks, but troubleshooting aftereach phase may extend the cloning process to multiple weeks or months.Day 41. Purify digested products2. Set up ligation reactionDay 1Order primers, prepare sourceof template123Day 81. Receive plasmidsequenceDay 61. Verify positive colonies via PCR2. Set up overnight culture45678Day 5Transform E. coliDay 31. Troubleshoot & optimize PCR2. Purify PCR products3. Digest PCR products & plasmidDay 71. Perform plasmid extraction2. Sequence plasmidwww.genscript.com6
ecificproducts fromPCR reactionLow or no PCRproduct yieldProblemPrimer design not optimal (causingnon-specific annealing, or primerdimer formation)DNA from a bacterial source or eukaryotic source may be blockedby Dam and Dcm methylation or CpG methylation, respectively. Use a Dam- or Dcm- strain. Follow general rules of primer design: Length from 18-30nucleotides, GC content from 40-60%, Tm of primers within 5 Cof each other. Avoid stretches of 4 or more of the same nucleotide ordinucleotide repeats. Avoid self-complementary sequences within primers.Primer design not optimal (causingnon-specific annealing, or primerdimer formation)Methylation of template sequenceprevents enzyme from cutting Follow general rules of primer design: Length from 18-30nucleotides, GC content from 40-60%, Tm of primers within 5 Cof each other. Avoid stretches of 4 or more of the same nucleotide ordinucleotide repeats. Avoid self-complementary sequences within primers.Reaction mix components arecompromised Check enzyme expiration date. Avoid multiple freeze thaw cycles of enzyme. Check expiration date of components. Aliquot biological components of reaction mixture and avoidmultiple freeze-thaw cycles.Reaction component concentrationsnot optimalEnzyme quality is compromised Check recommended primer concentrations (normally from0.05–1 mM) and Mg concentrations (normally from 0.2–1 mM).Not enough template For plasmids use 1 pg–10 ng of DNA / 50 μl reaction. For genomic DNA use 1 ng–1 μg of DNA / 50 μl reaction. Increase concentration of DNA template.Target sequence is not in DNAtemplateTemplate concentration is not optimal Re-extract DNA from source. Test another region of template.Primer concentration too low Use less primer. Check primer concentration; increase concentration ifnecessary.Reaction is missing Taq polymerase orother reaction componentPrimer concentration too high Make sure each component was added to PCR reaction.Thermocycler program annealing andextension temperatures are notoptimal Incrementally increase annealing temperature. Follow general rules of PCR design: Annealing temperature lowest primer Tm - 5 C, Extension temperature 72 C. Decrease annealing temperature by 6 to 10 C in stepwisefashion.Template is contaminated with PCinhibitorsAnnealing temperature too low Check DNA ratio of absorbance at 260 and 280 nm. Pure DNAshould have a 260/280 ratio of 1.8. Use less volume of the template in the reaction. Use DNA clean-up kit or ethanol precipitation to removecontaminants. Re-extract template. Try new reaction mixture. Use filter pipette tips and wear gloves during reaction set-up. Use electrophoresis to check DNA quality.Template is degradedTemplate or reaction mixturecomponents are contaminated Increase number of PCR cycles by 5.SolutionsNumber of PCR cycles is insufficientPotential CausesMolecular Cloning Troubleshooting Guide
9www.genscript.comwww.genscript.com10Sequencing oftransformantplasmid revealswrong plasmidsequenceNo insert in colonytransformantplasmidsNo plasmid incolonytransformantsFew or nocolonytransformantsTemplate/vectorappears to beover digestedDigestionproduct issmeared on gelIncomplete ornotemplate/vectordigestion Use a lower incubation temperature (25 – 30 C). Use a cell strain and vector designed for tightly controlled transcription.Competent cell viability is lowDNA insert encodes protein that istoxic to cells Repeat sequencing reaction. Aliquot working volumes of antibiotic and avoid freeze-thaw cycles. Add antibiotic to liquid plate media after sufficient cooling.Antibiotic is degradedInconclusive sequencing artifacts Use antibiotic concentration recommended by manufacturer.Antibiotic concentration too low Use a high-fidelity polymerase. Switch to a Rec A- cell strain.Construct recombined with genomic DNAMutations introduced by initial PCR Vector insert ratio not optimal. Use a vector:insert molar ratiofrom 1:1 to 1:10. Use a DNA concentration of 1-10 μg/ml.Low ligation efficiency Use a lower incubation temperature (25 – 30 C). Use a cell strain and vector designed for tightly controlled transcription. Use no more than 1-10 ng of DNA in 5 μl for a 100 μl reaction or in1-3 μl for a 50 μl reaction.Too much DNA in reactionDNA insert encodes protein that istoxic to cellsLigation reaction components can inhibit transformation. Dilute ligation reaction with TE buffer (up to 5 times).Too much ligation mixture was used forthe transformation Vector insert ratio not optimal. Use a vector:insert molar ratiofrom 1:1 to 1:10. Use a DNA concentration of 1-10 μg/ml. Dephosphorylate DNA with phosphatase to prevent re-ligation. Use electroporation for vectors over 10 kb.Construct is too bigVector re-ligation Reduce incubation time from 45 to 25 seconds.Heat-shock incubation too longThaw competent cells on ice and use immediately.Check expiration date of cells.Do not re-freeze cells.Do not vortex cells - gently tap to mix. Ensure the correct antibiotic was applied to plates. Use only concentration recommended by competent cell orantibiotic manufacturer.Enzyme is bound to DNA substrateWrong antibiotic was used or antibioticconcentration was too high Decrease units of enzyme. Remove enzyme from DNA by adding 0.1–0.5% SDS to loadingbuffer.Template is contaminated withnucleasesUse minimum incubation time.Reduce number of enzyme units in reaction.Use compatible buffer with enzyme.Use a high-fidelity enzyme. Use commercial kit to clean up template. Use running buffer and agarose gel made with fresh nuclease-freewater.Incompatible buffer was used Use recommended buffer with enzyme.Enzyme concentration too lowStar activity (enzyme cleaved similarrecognition sequence) Use 3–5 units of enzyme / μg of DNA.Template/plasmid contamination withinhibitors Add 1 to 2 hours to incubation time.Inhibitor contamination can originate from miniprep kits, or leftoverPCR reaction components: Use dialysis or spin column to remove contamination. Dilute or use less volume of DNA - DNA solution should composeno more than 25% of digestion reaction. Use PCR clean up kit to remove PCR reaction components.Not enough incubation time
Other Limitations of Traditional MolecularCloningIn addition to the bottlenecks encountered in traditional molecular cloning, otherlimitations avail. Traditional cloning is most useful for stitching together naturallyoccurring sequences. Engineering optimized or non-natural sequences can be muchmore difficult. In particular, gene sequences may be engineered to contain mutationsfor performing functional assays of the resulting protein or for characterization of thedisease state of a protein. In other cases, a researcher may desire to optimize thecodons of a natural gene sequence to enhance protein expression. In both cases,additional manipulation of the cloned gene is required, which may result inadditional DNA losses and unwanted mutations.Codon OptimizationIn order to characterize a gene’s function or purify a protein for structural study,researchers frequently need to express a gene in a host cell or model organismdifferent from the one in which it appears in nature. Unfortunately, heterologouslyexpressed genes often suffer from low rates of protein expression. A common causeof low expression levels is the variation in codon usage frequency between differentspecies. This can cause the translation of heterologously expressed genes to stall dueto tRNA scarcity, leading to lower protein levels and increased rates of improperprotein folding. The degeneracy of the genetic code makes it possible to change theDNA sequence in a way that does not alter the final amino acid sequence, but doeshave significant effects on the efficiency of transcription, mRNA stability, translation,and protein folding. Aside from codon usage bias, many other features of a DNAsequence affect the efficiency of transcription, the proper splicing and processing ofmRNA, and mRNA stability, all of which in turn reduce the ultimate protein yield(Figure 6).Figure 6: DNA sequence features that influence protein expression levels2. mRNA processing and stabilityDNADeviant foldingcryptic splice sitesmRNA secondary structurestable free energy of mRNANatural folding44. Protein folding211. Transcriptioncis-regulatory elements (TATA box,termination signal, protein bindingsites, etc.)chi sitespolymerase slippage sitesCGUcodon contextcodon-anticodoninteractiontranslation pausesites33. Translationcodon usage biasribosomal binding sites (e.g. IRES)premature polyA sitesModifying a DNA sequence to optimize it for efficient heterologous proteinexpression while preserving the amino acid sequence is called codon optimization. Ina study by Burgess-Brown et al. [13] that investigated the expression of 30 humangenes in E. coli, codon optimization increased both the total protein yield and theprotein solubility, compared to heterologous expression of native gene sequences.Codon optimization algorithms have improved since this 2008 study, and codonoptimization is now widely used by researchers to facilitate the purification ofproteins for structural studies, enzyme kinetics, and other biochemical investigations.MutagenesisThe mutation of gene sequences is useful for identifying amino acids crucial toprotein function, studying disease states of native proteins, facilitating drug discoveryor developing new protein variants (mutants) with properties desired for medical,industrial, agricultural, or other applications. Traditional methods for inducingrandom mutations include subjecting live cells or animals to UV radiation or chemicalmutagens in order to induce DNA damage. DNA repair enzymes can, in some cases,introduce or preserve mutations resulting from damage such as single- or doublestrand breaks, but this method leads to high rates of lethality and low rates of usefulmutant phenotypes.11www.genscript.comwww.genscript.com12
Depending on the type of mutagen used, mutations may be biased, limiting therange of possible mutants to only a fraction of those that are theoretically possibleor that can be obtained through other strategies.Site-directed mutagenesis may be designed based on experimental data such asX-ray crystallography data that identifies residues of interest. Site-directedmutagenesis can be performed using PCR-based methods with mutated primersequences to create and selectively amplify mutated sequences. This type ofmutagenesis must be followed by sequence verification, and can be tedious,error-prone, and costly.Gene Synthesis – The Solution tothe Limitations of Traditional CloningLuckily, a method that circumvents the limitations of traditional molecular cloningstrategies has been developed – gene synthesis. Gene synthesis is the de novosynthesis of a DNA strand. No template is required for gene synthesis and nearly anysequence that can be designed can be synthesized. In the next section theapplications and techniques that demonstrate how the versatility of gene synthesishas both accelerated and revolutionized molecular cloning are discussed.How Gene Synthesis Has RevolutionizedMolecular CloningWhat is Gene Synthesis?Gene synthesis technologies have revolutionized biology research and provide a morestraightforward, faster, and less costly alternative to traditional molecular cloning.Gene synthesis involves the de novo chemical synthesis of DNA, differing fromtraditional molecular cloning in that no template is required. Gene synthesis allowsresearchers to specify a desired sequence (native or engineered) and custom-build itdirectly.Gene synthesis refers to chemically synthesizing a strand of DNA base-by-base. UnlikeDNA replication that occurs in cells or by PCR, gene synthesis does not require atemplate strand. Rather, gene synthesis involves the step-wise addition of nucleotidesto a single-stranded molecule, which then serves as a template for creation of acomplementary strand.Any DNA sequence can be synthesized, including sequences that do not exist innature, or variants on naturally-occurring sequences that would be tedious toproduce through site-directed mutagenesis, such as codon-optimized sequences forincreased heterologous protein expression. Synthetic DNA can be cloned intoexpression vectors and used in any protocol that requires natural or recombinantDNA. Synthetic genes are used to study all the diverse biological roles that nucleicacids play, from encoding proteins and regulating gene expression in the nucleus, tomediating cell-cell communication and building biofilms from extracellular DNA.Due to gene synthesis, scientists are no longer limited to classical methods ofmanipulating a single gene at a time; they now have the power to design orreprogram entire genomes and cells which can advance the fields of healthcare,agriculture, energy, and other fields of human endeavor. For instance, newlyidentified viral genomes can be quickly synthesized to accelerate vaccinedevelopment. Novel enzymes can be engineered to fight cancer and producesustainable biofuels. Genes can be engineered to enhance crop yields and reducevulnerability to the common pests and plant diseases that endanger food suppliesand contribute to global hunger. Designer metabolic circuits using interchangeablesynthetic parts can be built to create synthetic genomes and artificial cells forstudying the basic requirements of life.138www.genscript.comwww.genscript.com914
Methods for de novo chemical synthesis of DNA have been refined over the past 60years. Synthetic short oligonucleotides (oligos) serve as custom primers and probesfor a wide variety of applications. Longer sequences that serve as genes or evenwhole genomes can be synthesized as well; these sequences are typically producedby synthesizing 40-200 bp oligos and then assembling them in the proper order.Many methods for oligo assembly have been developed that rely upon a DNApolymerase enzyme for PCR-based amplification, a DNA ligase enzyme for ligation ofoligos, or enzymes that mediate homologous recombination in vitro or in vivo. Mostsequences up to 1000 base pairs (1 kb) can be assembled in a standard molecularbiology lab, and commercial gene synthesis providers routinely synthesize sequencesover 10 kb.Figure 7: Timeline of gene synthesis technology development20041950Microchip- andmicrofluidics-based methodsfor high-throughput genesynthesis are first published.DNA polymerase isolated from E. coli. ArthurKornberg wins 1957 Nobel Prize.1990s1967DNA ligase isisolated andcharacterized byfive independentlaboratories.The history of gene synthesis began in 1955, when Sir Alexander Todd published achemical method for creating a phosphate link between two thymidine nucleosides,effectively describing the first artificial synthesis of a DNA molecule [14] The firstsuccessful synthesis of an entire gene was reported by Gobind Khorana’s group in1970; the 77 bp DNA fragment took 5 years to synthesize [15] Subsequentimprovements in DNA synthesis, sequencing, amplification, and automation havemade it possible now to synthesize genes over 1 kb in just a few days, and tosynthesize much longer sequences including entire genomes. Gene synthesis cannow be easily and cost-effectively outsourced to commercial providers. GenScript, apioneer in gene synthesis, was founded in 2002 and is the largest gene synthesissupplier in the world.1983PCR is invented.Kary Mullis wins1993 Nobel Prize.1983Caruthers andMatteucci inventphosphoramiditeDNA synthesis.Improvedassembly methodsallow synthesis ofincreasingly longgenes.Sc2.0 projectlaunched to buildthe first syntheticeukaryotic genome.1989First automatedoligonucleotidesynthesizer machinesbecome commerciallyavailable.197019552011First synthesis of an entire gene, a 77 bp yeasttRNA, by Gobind Khorana's group.First DNA molecule synthesized when two thymidine nucleosidesare joined by a phoshate link. Sir Alexander Todd wins 1957Nobel Prize.20082003Firstcompletesynthesisof abacterialgenome.Firstsynthesisof an entireviral genome,that of phiX174bacteriophage.What Can Be Synthesized?Gene synthesis can generate recombinant, mutated, or completely novel DNAsequences without a template, simplifying the creation of DNA tools that would belaborious to produce through traditional molecular cloning techniques. A widevariety of types of sequences can be produced to aid in diverse research applications(See Table 1). In addition to DNA sequences, RNA and oligos contain
The key principles of molecular cloning were discovered a little over 50 years ago. Since then, molecular cloning has become one of the most powerful tools of the molecular biology labor